Abstract
Background
Glioblastoma is the most devastating primary brain tumor of the central nervous system in adults. Magnetic nanocarriers may help not only for a targeted delivery of chemotherapeutic agents into the tumor site but also provide contrast enhancing properties for diagnostics using magnetic resonance imaging (MRI).
Methods
Synthesized hybrid chitosan-dextran superparamagnetic nanoparticles (CS-DX-SPIONs) were characterized using transmission electron microscopy (TEM) and relaxometry studies. Nonlinear magnetic response measurements were employed for confirming the superparamagnetic state of particles. Following in vitro analysis of nanoparticles cellular uptake tumor targeting was assessed in the model of the orthotopic glioma in rodents.
Results
CS-DX-SPIONs nanoparticles showed a uniform diameter of 55 nm under TEM and superparamagentic characteristics as determined by T1 (spin-lattice relaxation time) and T2 (spin-spin relaxation time) proton relaxation times. Application of the chitosan increased the charge from +8.9 to +19.3 mV of the dextran-based SPIONs. The nonlinear magnetic response at second harmonic of CS-DX-SPIONs following the slow change of stationary magnetic fields with very low hysteresis evidenced superparamagnetic state of particles at ambient temperatures. Confocal microscopy and flow cytometry studies showed an enhanced internalization of the chitosan-based nanoparticles in U87, C6 glioma and HeLa cells as compared to dextran-coated particles. Cytotoxicity assay demonstrated acceptable toxicity profile of the synthesized nanoparticles up to a concentration of 10 μg/ml. Intravenously administered CS-DX-SPIONs in orthotopic C6 gliomas in rats accumulated in the tumor site as shown by high-resolution MRI (11.0 T). Retention of nanoparticles resulted in a significant contrast enhancement of the tumor image that was accompanied with a dramatic drop in T2 values (P<0.001). Subsequent histological studies proved the accumulation of the nanoparticles inside glioblastoma cells.
Conclusion
Hybrid chitosan-dextran magnetic particles demonstrated high MR contrast enhancing properties for the delineation of the brain tumor. Due to a significant retention of the particles in the tumor an application of the CS-DX-SPIONs could not only improve the tumor imaging but also could allow a targeted delivery of chemotherapeutic agents.
Introduction
Despite multimodal treatment approach for glioblastoma multiforme that currently includes surgical resection of the tumor with radio- and pharmacotherapy with temozolomide (TMZ), the survival of patients does not exceed 15 months.Citation1,Citation2 Introduction of the tumor-treating fields (TTFields) as locoregional treatment only slightly improved the median overall survival in subgroups of patients, up to 20.5 months.Citation3 These data indicate the necessity for novel diagnostic and therapeutic modalities in neuro-oncology. Presumably, theranostic approaches could be based on the chitosan nanoparticles that show a high biocompatibility at relatively low costs. Previously, several studies reported the application of chitosan particles as nanocarriers of antiglioma agents.Citation4–Citation10 Thus, Liu et alCitation4 showed that chitosan surface-modified poly(lactide-co-glycolide)–chitosan nanoparticles (PLGA/CS NPs) loaded with carmustine (bis-chloroethylnitrosourea, BCNU) and its sensitizer (O6-benzylguanine, BG) had a strong antitumor effect in the F98 glioma-bearing mice model. In another study by Lollo et al,Citation11 the authors could demonstrate similar effects with paclitaxel (PTX)-loaded particles in GL261 glioma-bearing mice. Subsequent functionalization of the chitosan nanoparticle surface with bioligands further enhanced the ability of the particles to cross the blood–brain barrier (BBB) and their brain tumor accumulation level. Up-to-date, various ligands were proposed for decoration of the surface, including iRGD (tumor targeting peptide [CRGDKGPDC]) with high affinity to αv integrins,Citation4 transferrin receptor targeting agents,Citation5 and lactoferrin.Citation12
Alternative strategies based on chitosan particles could be their application, not only for the delivery of antitumor agents, but also for the detection of glioma. For the imaging of the glioblastoma in the current work, dextran-coated superparamagnetic iron oxide nanoparticles (DX-SPIONs) that were further coupled with chitosan were produced. The coupling of chitosan to preliminary activated dextran magnetic nanoparticles (MNPs) can increase the surface charge of the latter and thus improve the tumor uptake of SPIONs. Hence, as shown by Barrow et al,Citation13 an increase in charge of the 40 kDa dextran-based iron oxide nanoparticles (IONPs) from −1.5 to +18.2 mV provided a sixfold increase in uptake in mouse mesenchymal stem cells (MSCs) with respect to neutral dextran-coated particles. Furthermore, the hybrid chitosan–dextran-coated nanoparticles exert stronger membrane interactions as compared to monodextran particles.Citation14 Due to their unique magnetic and optical characteristics, such as high paramagnetism, low magnetic coercivity, and high Curie temperature, SPIONs show favorable characteristics compared to other types of magnetic nanocarriers.Citation15 Magnetic properties of the SPIONs were also employed for the development of the contrast agents for the magnetic resonance imaging (MRI) of brain tumors.Citation16,Citation17
In our study, the tumor targeting ability and the MRI contrast-enhancing properties of the synthesized hybrid dextran–chitosan SPIONs (CS-DX-SPIONs) were assessed in an orthotopic C6 Wistar rat model. In this study, we report that chitosan-based MNPs could be successfully employed for the MRI detection of brain tumors.
Materials and methods
Synthesis of the DX-SPIONs
The superparamagnetic (SPM) nanoparticles were obtained from iron salt solutions by coprecipitation at 80°C in alkaline media, as described previously.Citation18 Briefly, FeSO4 and FeCl3 (Fe2+/Fe3+ ratio of 1:2) salts were dissolved together with CsCl in water.Citation19 The magnetite precipitation was induced in an inert atmosphere by titration with NH4OH solution and collected using a permanent magnet. The nanoparticle suspension was treated by ultrasound at 22 kHz for 15 minutes. To prevent the sedimentation of the nanoparticles, 10 kDa molecular weight (MW) dextran (Sigma-Aldrich Co., St Louis, MO, USA) was added into dispersion in the process of ultrasound application. The obtained SPION stock solution was washed and separated into fractions by centrifugation and microfiltration using 0.2 μm pore size membranes (EMD Millipore, Billerica, MA, USA). The concentration of Fe in the suspension was assessed by ultraviolet (UV) absorption (at λ=480 nm) of the thiocyanate–Fe(+3) complex.
Preparation of the hybrid chitosan nanocarriers (CS-DX-SPIONs)
About 94% deacetylated chemical-grade chitosan (MW 30 kDa) was prepared from crab shells in formate solution at pH 6–7.4. DX-SPIONs were transferred into glass and aminated with epichlorohydrin. Chitosan was dissolved in 100 mL of water under magnetic stirring at pH 7.0. Hybrid nanoparticles were prepared by ionotropic gelation by mutual interaction of chitosan and dextran with negatively charged ion tripolyphosphate (TPP) (Vekton, St. Petersburg, Russia). An aqueous solution of TPP was added dropwise to the chitosan solution with suspended SPIONs. The hybrid nanoparticles were formed instantly after addition of TPP. The tertiary composition in reaction medium was as follows: polyvinylpyrrolidone (MW 3.6 MD; BASF), polyvinylpyrrolidone (PVP) (3% g/mL), TPP (0.04% g/mL), and chitosan (0.25% g/mL).
Physicochemical characterization of CS-DX-SPIONs
The particle size of SPIONs and size distribution were analyzed by transmission electron microscopy (TEM) using the JEOL-2000 microscope (JEOL, Tokyo, Japan) and dynamic light scattering (DLS) with the help of Malvern Instruments (Malvern, UK). Electrophoretic properties and hydrodynamic size were measured on Zetasizer Nano (Malvern Instruments). The relaxation times T1, T2, and T2* and 1H nuclear magnetic resonance (NMR) spectra were measured with the NMR spectrometer CXP-300 (Bruker Optik GmbH, Ettlingen, Germany) at the magnetic field of 7.1 T (corresponding to 300 MHz for 1H). The NMR spectra were registered with a one-pulse sequence by applying Fourier transform of free induction decay. The T2* values were estimated from line width at half height. To assess the T1 and T2 relaxation times, the inversion recovery and Carr–Purcell–Meiboom–Gill (CPMG) pulse sequences were employed. Proton relaxation times in buffer solution were recorded as a function of magnetic conjugate concentration. The R1, R2, and R2* (relaxivity) coefficients of relaxation efficiency were defined from the slopes of concentration plots. The 31P NMR spectra were obtained on a Bruker CXP-300 at 121 MHz using a 5 mm phosphorus probe and at 162 MHz on a WM 400 spectrometer using a 10 mm broadband probe. Phosphorus chemical shifts are referred relative to H3PO4 solution at pH 2. The 31P spin–lattice relaxation rate constants were obtained from 10 to 12 relaxation intervals using the inversion recovery pulse sequence at 120 MHz without proton decoupling.
Magnetic characterization of CS-DX-SPIONs
To characterize the magnetic state of the CS-DX-SPIONs (single-/multi-domain) and to estimate their average magnetic moment, we employed our home-built setup, registering the nonlinear longitudinal response (NLR) of MNPs to a weak ac magnetic field h(t) = h sin(ωt) parallel to steady field H (the frequency f = ω/2π =15.7 MHz and h =13.8 Oe). The setup included two coaxial Helmholtz coils (providing the linear scanning of steady field H with frequency, Fsc, varied in the range 8–10−2 Hz) combined with the electromagnet (for the creation of a constant field H0 if it is necessary) and a transmitter–receiver high frequency coil of a two-mode resonant system. The resonant system, on the one hand, creates ac field on a sample under study and, selects from the NLR the second harmonic M2 of the magnetization (NLR-M2) generated by a sample.Citation20 The M2 signal components, ie, ReM2(H) and ImM2(H), are recorded simultaneously as functions of H. The frequency of H-scan, Fsc, can be changed within 8–0.02 Hz, which allows the testing of the state (single-/multi-domain) of MNPs. Indeed, in the multi-domain state, the magnetization reversal is determined by movement of domain boundaries, which is fast, and H-hysteresis will not depend on Fsc. However, in single-domain MNPs, the field hysteresis appears in the blocking regime below blocking temperature, Tb. The latter can be found from condition τ = τmes (where τ is the time of the magnetic relaxation of MNPs ensemble and τmes is the time of measurements), and in our experiments, it depends on Fsc. Indeed, in the case of the uniaxial anisotropy applicable to our MNPs, 1/τ = f0exp(−Eb/kT) (where anisotropy energy Eb = KV, k is the Boltzmann constant, K is the anisotropy constant, V is an average volume of a particle, and the frequency f0 is of the order of 109 s−1) and Tb = KV/[k·ln(f0/Fsc)].Citation21 The dependence of H-hysteresis on Fsc evidences the single-domain state of MNPs in our study. Usually, NLR-M2 measurements are carried out under the condition M2 ∝ h2, which allows the application of the perturbation theory for analysis of the results. The main contribution to ReM2(H) arises due to the nonlinear behavior of M(H), so in the regime of the MNP ensemble close to the SPM one (a weak H-hysteresis/anhysteretic of M2(H) response), the ReM2(H) dependence can be semiquantitatively described by ∂2L(H)/∂H2 + kPMH, where L(H) is the Langevin function and the term kPMH takes into account possible small linear contributions from low-molecular paramagnetic fraction.Citation22 The ReM2(H) reaches extreme values near the inflection point of M(H), which is dependent on the average moment of MNPs that allows to estimate the latter. This description is not quantitative since it does not take into account the effect of the magnetic field on relaxation processes. This could be done by utilizing the Gilbert–Landau–Lifshitz formalism, but this is out of the scope of our study.Citation23
Assessment of cellular interactions of CS-DX-SPIONs
Cells
All employed cell lines (rat C6 glioma, human U87 glioma, and human cervix carcinoma HeLa cells) were obtained from the Russian Cell Culture Collection of the Institute of Cytology of the Russian Academy of Sciences (St Petersburg, Russia). C6 and HeLa cells were cultured in a CO2 incubator (at 37°C, 6% CO2) in DMEM culture medium supplemented with 2 mM L-glutamine, 10% fetal bovine serum (FBS), and antibiotics (ie, streptomycin [100 units/mL] and penicillin G [100 μg/mL]). Human U87 glioma cells were harvested in complete RPMI-1640 culture medium (Sigma-Aldrich Co.) supplemented with antibiotics and 10% FBS.
Cytotoxicity assay
For the analysis of cytotoxicity of CS-DX-SPIONs, the commercial non-radioactive cytotoxicity assay kit, CytoTox 96® (Promega Corporation, Fitchburg, WI, USA), was employed in accordance with the manufacturer’s protocol. Briefly, tumor cell lines (ie, C6 glioma, U87, and HeLa cells) were cocultured (for 1, 3, 12, and 24 hours) with SPIONs or hybrid CS-DX-SPIONs taken at different Fe concentrations (of 1, 5, 10, 50, and 150 μg/mL). The obtained supernatants from the samples were analyzed for the concentration of lactate dehydrogenase (LDH).
Confocal microscopy
For the assessment of cellular interactions of synthesized nanoparticles with tumor cells (ie, C6 glioma, HeLa cells, and U87 cells [1×106 cells/mL]), the latter were permitted to settle on poly-L-lysine-coated glass slides. SPIONs or CS-DX-SPIONs (Fe concentration: 150 μg/mL) were coincubated with the tumor cells for 1, 3, 12, and 24 hours in a CO2 incubator (37°C, 6% CO2). Prior to cell imaging, the nuclei of the cells were stained with 4,6-diamidine-2-phenylindole (DAPI) solution (Vector Laboratories, Burlingame, CA, USA). A series of the fluorescence z-images were recorded using a Leica TCS SP5 confocal microscope (Leica Microsystems, Heidelberg, Germany).
Flow cytometry analysis
For assessment of the nanoparticle internalization by tumor cells, a flow cytometer equipped with a coherent red solid state diode (635 nm), uniphase argon laser (488 nm), side scatter detector (SSD), and forward scatter detector (FSD), Cytomics FC500 (Beckman Coulter, Indianapolis, IN, USA) was used. The setups of the cytometer measured forward scattering (FSC) and side scattering (SSC) linearly and logarithmically. After an incubation period of 24 hours of the tumor cells (C6, U87, and HeLa) with DX-SPIONs, CS-DX-SPIONs (Fe concentration of 150 μg/mL), or phosphate buffered saline (PBS), the cells were analyzed by flow cytometry. The scatter parameters derived from the gated scatter region were used for counting cells within a large SSC (log) versus FSC (linear) histogram.
Gel-phantom model
For the assessment of the magnetic properties of the CS-DX-SPIONs following cellular uptake, the C6 glioma cells were coincubated with DX-SPIONs (Fe concentration: 150 μg/mL), CS-DX-SPIONs (Fe concentration: 150 μg/mL), or PBS for 24 hours. Following coincubation and washing with PBS, the cells were embedded into 1% agarose gel. For the assessment of the intracellular nanoparticle localization, several anatomical high-resolution turbo spin echo (SE) T2-weighted coronal images (20 slices in total) were obtained using the following parameters: repetition time (TR)/echo time (TE) 4,200/36 ms, flip angle (FA) 180°, slice thickness of 1.0 mm, interslice distance 1.2 mm, field of vision (FOV) 3.0×3.0 cm, and matrix 256×256. Subsequently, gradient echo (GE) scans (FLASH) (TR/TE 350/5.4 ms, FA 40°, slice thickness 1.0 mm, 3.0×3.0 cm, and matrix 256×256) and SE T1-weighted images (TR/TE 1,500/7.5 ms, FA 180°, slice thickness 1.0 mm, FOV 3.0×3.0 cm, and matrix 256×256) were obtained in the coronal plane. Accumulation of the DX-SPIONs or CS-DX-SPIONs in the samples was assessed using multi-scan multi-echo (MSME) sequences.
Orthotopic C6 glioma model
Male Wistar rats with a body weight of 280–300 g were obtained from the “Rappolovo” RAMN animal nursery (St Petersburg, Russia). For all animal experiments, the approval has been provided by the local ethical committee of First Pavlov Saint Petersburg State Medical University (St Petersburg, Russia) in accordance with institutional guidelines for the welfare of animals. Before mounting in a stereotactic frame (David Kopf Instruments, Tujunga, CA, USA), the rats were anesthetized with 0.2 mL 2% Rometar (xylazine hydrochloride; “Bioveta”, Ivanovice na Hané, Czech Republic) and 10 mg tiletamine hydrochloride and zolazepam (“Virbac santé Animale”, Carros, France) intraperitoneally. C6 rat glioma cells (1×106 cells in 10 μL of PBS) were stereotactically infused into the nucl. caudatus dexter (right caudate nucleus) using a Hamilton microsyringe.
Magnetic resonance assessment of CS-DX-SPIONs’ tumor-targeting potential
On the 15th day following the intracranial injection of the C6 tumor cells for the assessment of the accumulation of DX-SPIONs or CS-DX-SPIONs in the tumor site, the animals were randomly divided into three groups as follows (six rats in each group): 1) control group – intravenous (i.v.) injection of PBS via the tail vein; 2) i.v. administration of DX-SPIONs (Fe concentration of 2.5 mg/kg); and 3) i.v. administration of the CS-DX-SPIONs (Fe concentration of 2.5 mg/kg). Twenty-four hours after i.v. injection, intratumoral retention of MNPs was assessed using a high-field MRI scanner (11.0 T) (Avance III 400 WB; Bruker Optik GmbH) in the following conventional sequences: 1) T1-weighted fast-field echoes (TR/TE [TR] 1,500/7.5 ms, FOV 3.0×3.0 cm, matrix 256×256, FA 180° number of signals averaged [NSA]); 2) T2-weighted turbo SE (FA 180°, TR 4,200 ms, and TE 36 ms, FOV 3.0×3.0 cm, matrix 256×256); 3) FLASH scans (GE) (FA 40°, TR 350 ms, and TE 5.4 ms); and 4) MSME regimen. All images were obtained with a slice thickness of 1.0 mm in the coronal plane. Assessment of the MRI scans was carried out using the Paravision 3.1 software package (Bruker BioSpin GmbH, Rheinstetten, Germany) and Analyze software (AnalyzeDirect, Inc., Overland Park, KS, USA).
Histology
For histological analysis, the rats were sacrificed using an intraperitoneal injection of pentobarbital (150–200 mg/kg) with subsequent perfusion with PBS (100 mL) and 4% paraformaldehyde (PFA). For the analysis of the intratumoral distribution of nanoparticles, the obtained serial cryosections (5–7 μm) were additionally stained with DAPI and assessed by using a Leica TCS SP5 confocal microscope in a reflecting laser scanning regimen (laser 488 nm [Ar/Kr]).
Statistical analysis
For the analysis of the statistical differences, one-way analysis was carried out by the Kruskal–Wallis test. Student’s t-test was performed to compare the obtained animal data using Statistica Version 9.2 program. Differences were considered to be statistically significant when P<0.05.
Results
Synthesized CS-DX-SPIONs exhibit high-contrast-enhancing properties
The sequence of the steps in the synthesis of hybrid SPIONs is represented in . Following the addition of dextran to the prepared SPIONs for stabilization of the particles, the average size ranged between 30 and 40 nm. According to TEM measurements, DX-SPIONs are presented as round-shaped particles. For further coating of the particles with chitosan, the surface of DX-SPIONs was activated by NH2 groups through addition of epichlorohydrin. Chemical activation did not change the stability of nanoparticle dispersion (as assessed by DLS). Size distribution measured by the DLS method was 55 nm. The hybrid dextran–chitosan coat of the magnetite core was obtained in the cross-linking procedure with the help of TPP shown in as Step III. Negatively charged ions of TPP induce ionotropic gelation of chitosan and dextran. To study the molecular mechanism of interaction between TPP and chitosan, 31P NMR measurements were conducted with gradual increase in TPP concentration in the reaction solution. According to the 31P NMR spectra, three α-, β-, and γ-PO4 groups produce two well-resolved lines at different chemical shifts (). The low-shift line (ppm) belongs to α and γ phosphate groups. In the process of titration, initial addition of TPP did not lead to the corresponding increase in intensity resonance. According to the theoretical analysis, the 31P relaxation rates of phosphate groups in strong magnetic field (7.1 T) due to dipole–dipole (DD) and chemical shift anisotropy (CSA) mechanisms are well described by Bloembergen et al.Citation24,Citation25 In line with this theory, ion–ion interaction of PO4 groups with protonated NH3+ groups reduces molecular motion of TPP and leads to strong decrease in magnetic relaxation times T1 and T2 and thus loss of spectral resolution. At low TPP concentrations, the resonance lines are invisible. At saturation threshold of all free NH3+ groups, excess of TPP agent gives rise to narrow PO4 lines in spectra with intensity proportional to TPP concentration. The observed concentration dependencies are in good agreement with the hypothesis that one molecule of TPP binds 2–3 positively charged NH3 groups. Coverage of magnetic nuclei by the dextran–chitosan coat results in an increase in the hydrodynamic diameter of nanoparticles and initiation of magnetic moment in the whole nanostructure. With respect to the small size of the nanoparticles (40 nm) one might assume that the magnetic particle consists of a single domain nanocrystal. In this case, the nanoparticle would behave as an SPM unit exposing classical hysteresis behavior in accordance to the Langevin law.Citation26 Otherwise, the particles behave as a ferromagnetic material. To elucidate the magnetic nature of hybrid CS-DX-SPIONs, the novel NLR-M2 approach was applied to assess the particles for hysteresis criteria. Both components, ReM2 and ImM2 of nonlinear response, were measured versus steady field H at T=294 K at different rates of field scan, Fsc. The obtained H-dependencies display the characteristic signals, exhibiting extremes at a weak magnetic field H in H>0 range of H-scan, opposite signs in ReM2(H) and ImM2(H) components, and the weak field hysteresis, which decreases with a reduction in Fsc. These features correspond to M2 response of ensemble of MNPs in the single domain state ().Citation27 A weak field hysteresis is predictive for a close to SPM regime, which is in accordance with a single domain structure. M2 responses yielded by the Fe3O4 nuclei and magnetic parameters of the latter in the first approximation are not affected by the formation of the hybrid dextran–chitosan shell. The response of an ensemble of NPs in SPM regime is characterized by the absence of field hysteresis. It is obvious that in this case the magnetization M will be directed along the field, parallel to the z axis. As for complex magnetic materials such as manganites, cobaltites, and composite substances on co-compounds, the shape of hysteresis curves depends on Fsc and temperature, and SPM regime occurs above blocking temperature TB.Citation28,Citation29 The hybrid CS-DX-SPIONs have high magnetic moment to accelerate magnetic relaxation of aqueous protons in suspension due to large perturbation of the magnetic field in the neighborhood of MNPs. Inhomogeneous magnetic fields in the probe can be calculated as the sum of dipole fields induced by magnetite cores. The inhomogeneous character of the field shortens the magnetic relaxation times of H2O.
Figure 1 Schematic representation of the synthesis of chitosan-based superparamagnetic iron oxide nanoparticles (CS-DX-SPIONs).
Abbreviations: CS-DX-SPIONs, chitosan–dextran superparamagnetic iron oxide nanoparticles; MNP, magnetic nanoparticle; TPP, tripolyphosphate.
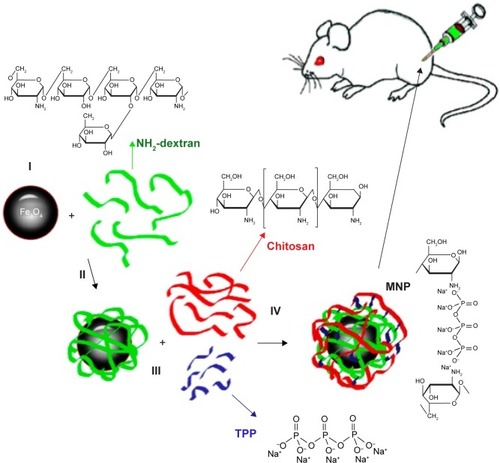
Figure 2 31Phosphorus NMR spectroscopy of the TPP.
Abbreviations: NMR, nuclear magnetic resonance; TPP, tripolyphosphate.
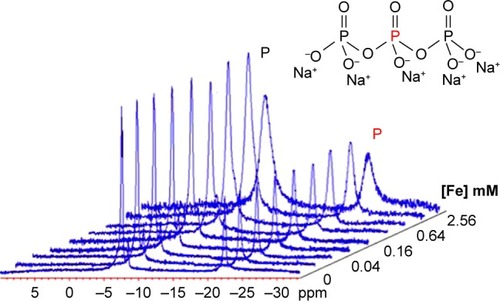
Figure 3 Second harmonic measurements of CS-DX-SPIONs in water at different frequencies of H-scan, Fsc=8 (panels (a) and (b)) and 0.25 Hz (panels (c) and (d)), T=294 K.
Notes: Solid and open circles display direct and reverse H-scans, respectively. Dashed-dot curve in panel (c) presents fit ReM2(H) dependence obtained at Fsc=0.25 Hz by ∂2L(H)/∂H2 + kPMH.
Abbreviation: CS-DX-SPIONs, chitosan–dextran superparamagnetic iron oxide nanoparticles.
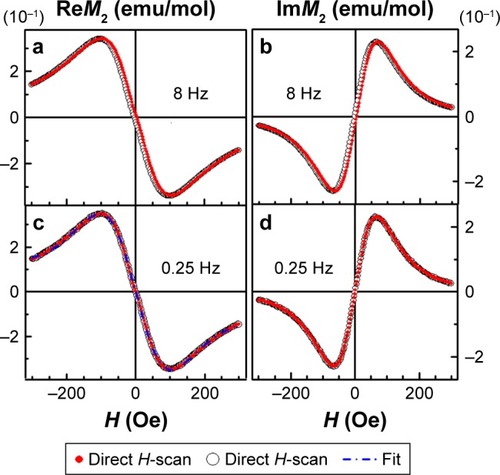
Assessment of cellular interactions of CS-DX-SPIONs
Uptake of the synthesized DX-SPIONs and hybrid CS-DX-SPIONs was analyzed in tumor cells (C6, U87, and HeLa) following coincubation of the latter with DX-SPIONs or CS-DX-SPIONs at various concentrations of Fe3+ (ie, 1, 10, 50, and 150 μg/mL) and incubation periods (ie, 1, 3, 6, 12, and 24 hours). Following an overnight coincubation, the confocal microscopic views show that DX-SPIONs were incorporated into the cytosol of the tumor cells (). Magnetic conjugates on the reflective laser scanning are presented as green dots throughout the cytosol of the tumor cells but are not detected in the nucleus of the cells (). In the case of hybrid CS-DX-SPIONs, an increase in the internalization of nanoparticles as compared to DX-SPIONs was detected (). The uptake of the CS-DX-SPIONs was further evaluated as related to their contrast-enhancing properties when coincubated with C6 glioma cells. Accumulation of the hybrid SPIONs in the cells led to the hyperintense T2-weighted MRI images and subsequent decrease in T2 values (). This indicates that CS-DX-SPIONs preserve their magnetic contrast-enhancing properties after internalization into cells. Thus, T2 values following internalization of the CS-DX-SPIONs constituted 6.0±1.34 ms which was significantly lower in comparison to the DX-SPIONs and control (PBS), 11.72±2.65 and 20.1±2.45 ms, respectively (P<0.001) (). To further assess the internalization of nanoparticles, flow cytometry was applied. These data clearly showed an enhanced accumulation of CS-DX-SPIONs in the C6, U87, and HeLa cells as compared to the dextran-coated particles after 24 h (). The retention of the particles significantly increased the granularity (SSC) of the tumor cells, but not their size (FSC) (). After internalization, the cytotoxicity of the nanoparticles, by the detection of LDH in the samples, was analyzed. A dose and time-dependent toxicity of the CS-DX-SPIONs after coincubation with tumor cells was observed (). Twenty-four hours after coincubation with CS-DX-SPIONs (at a Fe concentration of 150 μg/mL), the cytotoxicity of C6, U87, and HeLa cells was 30.1%±6.4%, 36.2%±7.9%, and 27.4%±6.2%, respectively.
Figure 4 Interaction of CS-DX-SPIONs with cells.
Notes: (A) Confocal microscopy images of the rat C6 glioma cells coincubated for 24 hours with PBS (control), DX-SPIONs, or CS-DX-SPIONs. Nanoparticles were detected using reflecting laser scanning at 488 nm (presented as green dots). Nuclei were stained with DAPI (blue). Scale bar, 7 μm. (B) T2 values (ms) of the C6 cells following coincubation with PBS (control), DX-SPIONs, or CS-DX-SPIONs for 24 hours in 0.1% agarose gel. Data are presented as mean ± SD. (C) SSC, (D) FSC, and (E) cell fluorescence for three cell lines (C6, U87, and HeLa) following coincubation with PBS (control), DX-SPIONs, or CS-DX-SPIONs for 24 hours. The mean and SD were calculated for the three experiments. T2 is the spin-spin relaxation time.
Abbreviations: CS-DX-SPIONs, chitosan–dextran superparamagnetic iron oxide nanoparticles; DAPI, 4,6-diamidine-2-phenylindole; DX-SPIONs, dextran-coated superparamagnetic iron oxide nanoparticles; FSC, forward scattering; PBS, phosphate buffered saline; SSC, side scattering.
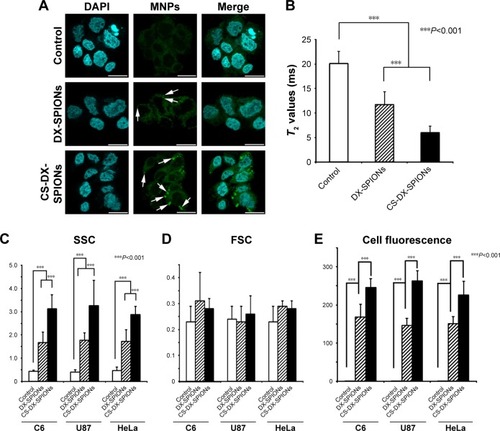
Figure 5 Cytotoxicity assay of the tumor cells (C6, U87, and HeLa) coincubated with PBS (control), DX-SPIONs, or CS-DX-SPIONs.
Note: The mean and SD was calculated for the three experiments.
Abbreviations: CS-DX-SPIONs, chitosan–dextran superparamagnetic iron oxide nanoparticles; DX-SPIONs, dextran-coated superparamagnetic iron oxide nanoparticles; h, hour; PBS, phosphate buffered saline.
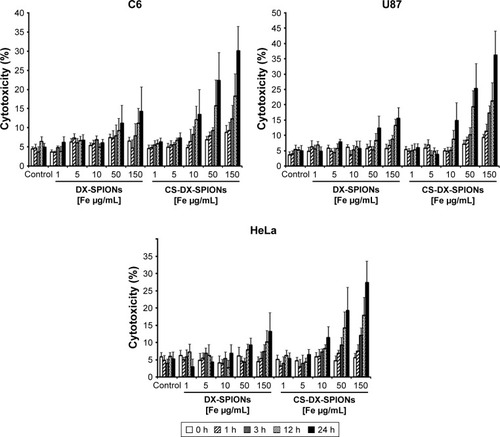
Targeting of the brain tumor by hybrid CS-DX-SPIONs
A total of 15 days after orthotopic injection of C6 glioblastoma cells, the animals were randomly divided into three groups (six animals each): 1) control group injected with PBS; 2) i.v. administration of the DX-SPIONs; and 3) i.v. injection of CS-DX-SPIONs. Twenty-four hours after the injection of the nanoparticles, brain tumors were imaged on the high-field MRI scanner. In control animals, the tumor was presented as hypointense on the T1-weighted images and hyperintense on T2 images (). In the DX-SPIONs-injected animal group, glioma was characterized by a hypointense signal in the T2-weighted imaging. Twenty-four hours after i.v. infusion of CS-DX-SPIONs, a significant decrease in the T2 relaxivity has been observed in comparison to control and SPION-injected animals (17.1±1.4, 10.2±1.4, and 5.8±1.2 ms, respectively; P<0.001). Reduction of the transverse relaxation time T2 is accompanied by the growth of image contrast due to the darkening of accumulation sites of MNPs. Subsequent histological assessment of brain tumor cryosections confirmed the accumulation of the MNPs inside glioma cells (). The distribution pattern of DX-SPIONs or hybrid CS-DX-SPIONs retention in the tumor did not differ.
Figure 6 Targeting of the C6 glioma with CS-DX-SPIONs.
Notes: (A) Magnetic resonance images of the brain tumors of the control (PBS), animals treated with DX-SPIONs and CS-DX-SPIONs. T1-weighted, T2-weighted and gradient echo (FLASH) images of the glioblastoma were obtained. Accumulation of the nanoparticles is shown by solid red arrows. (B) T2 values (ms) of the brain tumors in the control (PBS), animals treated with DX-SPIONs and CS-DX-SPIONs. Data are presented as mean ± SD. (C) Immunofluorescent images of the glioma. Nuclei stained with DAPI (blue). Nanoparticles detected using reflective laser scanning at 488 nm (green). Scale bar, 40 μm. (D) Quantification of the nanoparticles in the glioma tissue. Data are presented as mean ± SD. T1 – spin-lattice relaxation time and T2 – spin-spin relaxation time.
Abbreviations: CS-DX-SPIONs, chitosan–dextran superparamagnetic iron oxide nanoparticles; DAPI, 4,6-diamidine-2-phenylindole; DX-SPIONs, dextran-coated superparamagnetic iron oxide nanoparticles; MNPs, magnetic nanoparticles; PBS, phosphate buffered saline.
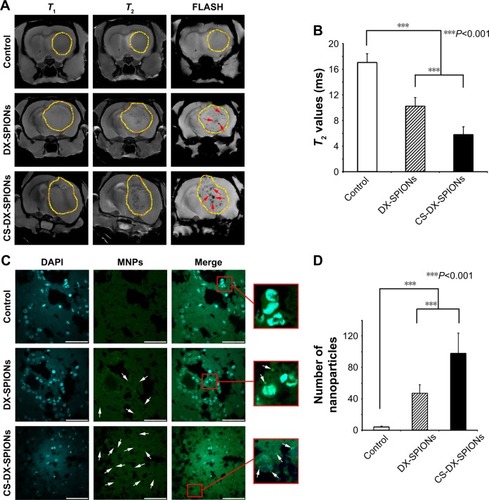
Discussion
Nanotechnology can provide a novel theranostic approach in managing glioblastoma multiforme.Citation30,Citation31 In our studies, SPIONs with a high MRI contrast enhancement capacity have been used.Citation32 For an improved biocompatibility, nanoparticles were coated with chitosan that increased the charge of the dextran-coated nanoparticles to +19.2 mV. Chitosan, a polysaccharide with a positive charge at neutral or acidic pH, has advantageous biocompatibility as a carrier material,Citation33 a strong permeation enhancing capability for hydrophilic compounds,Citation34 and bioadhesive properties.Citation35 An increase in charge of the nanoparticles also resulted in an enhanced uptake of CS-DX-SPIONs by tumor cells (). The same effect was previously reported for positively charged pullulan-coated NPs that were incorporated by bone marrow-derived rat MSCsCitation36 or mouse MSCs.Citation13 On the other hand, the decrease in the surface charge abrogated the internalization of the nanoparticles.Citation36
Although we observed an enhanced intracellular uptake of the particles, we subsequently determined an increased cytotoxicity of CS-DX-SPIONs (). This could be explained by the production of reactive oxygen species (ROS) and subsequent DNA damage.Citation37,Citation38 Increased amounts of ROS might also negatively affect mitochondrial DNA (mDNA).Citation39
Synthesized CS-DX-SPIONs also act as a negative MRI contrast agent. The measured proton relaxation rates of water in magnetic suspensions of CS-DX-SPIONs clearly indicated a high contrast property of the particles (). The registered relaxation rates in the hybrid CS-DX-SPIONs resembled the main relaxation features of previously reported dextran-coated MNPs and commercial NP formulations.Citation16,Citation17 The composite shell (between chitosan and dextran) cross-linked by TPP does not hamper the diffusion of protons into dephasing volume around MNPs thus preserving the MRI contrast-enhancing properties of the particles. Following coincubation with tumor cells, CS-DX-SPIONs accumulated in cytosol, which resulted in a decrease in T2 values when the samples were analyzed using MRI scanning (). This indicates that the obtained NPs preserve their magnetic properties after internalization into cells. In the current study, for the analysis of accumulation of nanoparticles in the glioma, standard MRI sequences (ie, T2-weighted scans, GE [FLASH], and multi-scan multi-echo [MSME]) were applied. Recently, other MRI methods for visualization of the MNPs were proposed including investigation of transverse relaxation rates R2 and R2*,Citation40 quantitative susceptibility mapping (QSM),Citation41 and susceptibility-weighted imaging (SWI).Citation42–Citation44 In the study by Blasiak et al, the highest contrast-to-noise ratio for the detection of the iron deposition in an U87MG glioma model was achieved when the SWI was employed in comparison to any other pulse sequences (ie, SE and GE).Citation42 The magnitude of magnetic relaxation rate of hybrid CS-DX-SPIONs corresponded to the contrast enhancement of the agent in MRI scans of brain tumors in rats (). Following intravenous administration, CS-DX-SPIONs accumulated in glioma cells, which significantly enhanced the contrasts of the tumor. Retention of the particles resulted in a significant decrease in T2 values compared to DX-SPIONs.
Accumulation of the particles inside glioma cells could be explained by an enhanced permeation and retention (EPR) effect.Citation45 Thus, particles <100 nm in diameter extravasate preferentially from the chaotic tumor vasculature, due to the increased permeability of vessels compared to normal tissues.Citation46 Presumably, further modifications of the surface of CS-DX-SPIONs with targeting bioligands (eg, antibodies, peptides, proteins, etc) could further increase the accumulation of particles inside glioma tissue. Previously, several studies demonstrated that coating with tumor-targeting molecules including anti-Hsp70 antibody,Citation16 peptide-targeting interleukin-13 receptor α2 (IL-13Rα2),Citation47 anti-VEGF antibodies,Citation48 antibodies toward EGFR deletion mutant (EGFRvIII),Citation49 anti-insulin-like growth factor binding protein 7 (anti-IGFBP7) single domain antibody,Citation50 and many others could show an enhanced particle retention inside tumor cells.Citation51–Citation54
Conclusion
Hybrid CS-DX-SPIONs synthesized by ionotropic gelation at the SPM state have a high MRI contrast-enhancing activity and tumor-targeting potential. In vitro experiments clearly demonstrated that the increase in the surface charge of the nanoparticles due to the chitosan coating subsequently enhanced the intracellular uptake of particles and thus increased their cytotoxic activity. Administration of the magnetic nanocarriers via clinically relevant routes resulted in a significant contrast enhancement of glioma. Diagnostic properties of the synthesized hybrid nanoparticles could be further explored for the development of targeted therapies.
Acknowledgments
The authors are grateful to Olga G Genbach, Nelly V Koroleva, Olga I Dobrotina, and Prof Dr Oleg V Galibin for help in experiments in vivo on rodents. The MRI measurements were performed in the Center for Magnetic Resonance at St Petersburg State University. The study was supported by the Alexander von Humboldt Fellowship and by the Russian Foundation for Basic Research 15-08-08148A; animal experiments were supported in part by a grant of the Russian Science Foundation 14-50-00068 and by the Federal Agency of Scientific Organizations, Russia, State Grant of the Ministry of Health of the Russian Federation No 32, and by a DFG grant SFB824/3, Germany.
Disclosure
The authors report no conflicts of interest in this work.
References
- StuppRHegiMEMasonWPEffects of radiotherapy with concomitant and adjuvant temozolomide versus radiotherapy alone on survival in glioblastoma in a randomised phase III study: 5-year analysis of the EORTC-NCIC trialLancet Oncol200910545946619269895
- WellerMvan den BentMTonnJCEuropean Association for Neuro-Oncology (EANO) guideline on the diagnosis and treatment of adult astrocytic and oligodendroglial gliomasLancet Oncol2017186e315e32928483413
- StuppRTaillibertSKannerAAMaintenance therapy with tumor-treating fields plus temozolomide vs temozolomide alone for glioblastoma: a randomized clinical trialJAMA20153142535254326670971
- LiuCYaoSLiXWangFJiangYiRGD-mediated core-shell nanoparticles loading carmustine and O(6)-benzylguanine for glioma therapyJ Drug Target20172523524627646474
- AgrawalPSinghRPSonaliTPGS-chitosan cross-linked targeted nanoparticles for effective brain cancer therapyMater Sci Eng C Mater Biol Appl20177416717628254282
- XuYAsgharSYangLNanoparticles based on chitosan hydrochloride/hyaluronic acid/PEG containing curcumin: in vitro evaluation and pharmacokinetics in ratsInt J Biol Macromol20171021083109128472690
- ChenYFengSLiuWYuanZYinPGaoFVitamin E succinate-grafted-chitosan oligosaccharide/RGD-conjugated TPGS mixed micelles loaded with paclitaxel for U87MG tumor therapyMol Pharm2017141190120328212490
- KievitFMStephenZRWangKNanoparticle mediated silencing of DNA repair sensitizes pediatric brain tumor cells to γ-irradiationMol Oncol201591071108025681012
- DanhierFMessaoudiKLemaireLBenoitJPLagarceFCombined anti-Galectin-1 and anti-EGFR siRNA-loaded chitosan-lipid nanocapsules decrease temozolomide resistance in glioblastoma: in vivo evaluationInt J Pharm201548115416125644286
- TrapaniADenoraNIacobellisGSitterbergJBakowskyUKisselTMethotrexate-loaded chitosan- and glycol chitosan-based nanoparticles: a promising strategy for the administration of the anticancer drug to brain tumorsAAPS PharmSciTech2011121302131121948322
- LolloGVincentMUllio-GamboaGDevelopment of multifunctional lipid nanocapsules for the co-delivery of paclitaxel and CpG-ODN in the treatment of glioblastomaInt J Pharm201549597298026428632
- XuYAsgharSYangLLactoferrin-coated polysaccharide nanoparticles based on chitosan hydrochloride/hyaluronic acid/PEG for treating brain gliomaCarbohydr Polym201715741942827987946
- BarrowMTaylorANievesDJTailoring the surface charge of dextran-based polymer coated SPIONs for modulated stem cell uptake and MRI contrastBiomater Sci2015360861626222421
- PattaniAPatravaleVBPanikerLPotdarPDImmune effects and membrane interaction of chitosan nanoparticlesMol Phar2009623452352
- Petri-FinkAHofmannHSuperparamagnetic iron oxide nanoparticles (SPIONS): from synthesis to in vivo studies – a summary of the synthesis, characterization, in vitro, and in vivo investigations of spions with particular focus on surface and colloidal propertiesIEEE Trans Nanobioscience2007628929718217622
- ShevtsovMANikolaevBPRyzhovVAIonizing radiation improves glioma-specific targeting of superparamagnetic iron oxide nanoparticles conjugated with cmHsp70.1 monoclonal antibodies (SPION-cmHsp70.1)Nanoscale20157206522066426599206
- ShevtsovMANikolaevBPYakovlevaLYSuperparamagnetic iron oxide nanoparticles conjugated with epidermal growth factor (SPION-EGF) for targeting brain tumorsInt J Nanomedicine2014927328724421639
- ShevtsovMANikolaevBPYakovlevaLY70-kDa heat shock protein coated magnetic nanocarriers as a nanovaccine for induction of anti-tumor immune response in experimental gliomaJ Control Release201522032934026522072
- MassartRPreparation of aqueous magnetic liquids in alkaline and acidic mediaIEEE Trans Magn19811712471248
- RyzhovVALarionovIIFomichevVNOn the spurious signal in the longitudinal nonlinear susceptibility of magnets at the second harmonic of excitation frequencyTech Phys199641620626
- GullityBDGrahamCDIntroduction to Magnetic MaterialsNew YorkIEEE Press. Wiley2009
- RyzhovVAPleshakovIVNechitailovAAMagnetic study of nanostructural composite material based on cobalt compounds and porous siliconAppl Magn Resonan201445339352
- LazutaAVRyzhovVARunovVVKhavroninVPDeriglazovVVTemperature evolution of superparamagnetic clusters in single-crystal La0.85Sr0.15CoO3 characterized by nonlinear magnetic ac response and neutron depolarizationPhys Rev B2015921440414411
- BloembergenNPurcellEMPoundRVRelaxation effects in Nuclear Magnetic Resonance AbsorptionPhys Rev194873679
- AbrahamAPrinciples of Nuclear MagnetismOxfordClarendon Press1961599
- KittelCIntroduction to Solid State Physics8th edIndianapolis, INJohn Wiley & Sons, Inc2005690
- RyzhovVALazutaAVMolkanovPIComparative study of heterogeneous magnetic state above TC in La0.82Sr0.18CoO3 cobaltite and La0.83Sr0.17MnO3 manganiteJMMM201232434323436
- LazutaAVLarionovIIRyzhovVASecond-harmonic nonlinear response of a cubic ferromagnet in the critical paramagnetic neighborhood of TCSov Phys JETP19917310861095
- RyzhovVALazutaAVLuzyaninIDThe nonlinear magnetic properties of the pseudocubic Nd0.77Ba0.23MnO3 single crystal in the critical paramagnetic region and phase separationJETP200294581592
- GlaserTHanIWuLZengXTargeted nanotechnology in glioblastoma multiformeFront Pharmacol2017816628408882
- PintoMPArceMYameenBVilosCTargeted brain delivery nanoparticles for malignant gliomasNanomedicine (Lond)201712597227876436
- ShevtsovMMulthoffGRecent developments of magnetic nanoparticles for theranostics of brain tumorCurr Drug Metab20161773774427280470
- LiuHGaoCPreparation and properties of ionically cross-linked chitosan nanoparticlesPoly Adv Technol200920613619
- KhanSAPatilKSYeolePGIntranasal mucoadhesive buspirone formulation: in vitro characterization and nasal clearance studiesPharmazie Die200863348351
- PapadimitriouSBikiarisDAvgoustakisKKaravasEGeorgarakisMChitosan nanoparticles loaded with dorzolamide and pramipexoleCarbohydr Polym2008734454
- JoJAokiITabataYDesign of iron oxide nanoparticles with different sizes and surface charges for simple and efficient labeling of mesenchymal stem cellsJ Control Release201014246547319932720
- WatanabeMYonedaMMorohashiAEffects of Fe3O4 magnetic nanoparticles on A549 cellsInt J Mol Sci201314155461556023892599
- LiuGGaoJAiHChenXApplications and potential toxicity of magnetic iron oxide nanoparticlesSmall201391533154523019129
- EspositoLAMelovSPanovACottrellBAWallaceDCMitochondrial disease in mouse results in increased oxidative stressProc Natl Acad Sci U S A1999964820482510220377
- LangkammerCKrebsNGoesslerWQuantitative MR imaging of brain iron: a postmortem validation studyRadiology2010257245546220843991
- HaackeEMLiuSBuchSZhengWWuDYeYQuantitative susceptibility mapping: current status and future directionsMagn Reson Imaging201533112525267705
- BlasiakBBarnesSFoniokTComparison of T2 and T2*-weighted MR molecular imaging of a mouse model of gliomaBMC Med Imaging2013132023865826
- BlasiakBLandryJTysonRMolecular susceptibility weighted imaging of the glioma rim in a mouse modelJ Neurosci Methods201422613213824525326
- HaackeEMXuYChengYCReichenbachJRSusceptibility weighted imaging (SWI)Magn Reson Med200452361261815334582
- MatsumuraYMaedaHA new concept for macromolecular therapeutics in cancer-chemotherapy – mechanism of tumoritropic accumulation of proteins and the antitumor agent smancsCancer Res198646638763922946403
- PerraultSDWalkeyCJenningsTFischerHCChanWCWMediating tumor targeting efficiency of nanoparticles through designNano Lett200991909191519344179
- WangBLvLWangZImproved anti-glioblastoma efficacy by IL-13Rα2 mediated copolymer nanoparticles loaded with paclitaxelSci Rep201551658926567528
- AbakumovMANukolovaNVSokolsky-PapkovMVEGF-targeted magnetic nanoparticles for MRI visualization of brain tumorNanomedicine20151182583325652902
- HadjipanayisCGMachaidzeRKaluzovaMEGFRvIII antibody-conjugated iron oxide nanoparticles for magnetic resonance imaging-guided convection-enhanced delivery and targeted therapy of glioblastomaCancer Res2010706303631220647323
- TomanekBIqbalUBlasiakBEvaluation of brain tumor vessels specific contrast agents for glioblastoma imagingNeuro Oncol2012141536322013169
- KratzerIWernigKPanzenboeckUApolipoprotein A-I coating of protamine-oligonucleotide nanoparticles increases particle uptake and transcytosis in an in vitro model of the blood–brain barrierJ Control Release2007117330131117239472
- ZensiABegleyDPontikisCAlbumin nanoparticles targeted with Apo E enter the CNS by transcytosis and are delivered to neuronsJ Control Release2009137788619285109
- MadhankumarABSlagle-WebbBMintzASheehanJMConnorJRInterleukin-13 receptor-targeted nanovesicles are a potential therapy for glioblastoma multiformeMol Cancer Ther200653162316917172420
- LiuLGuoKLuJBiologically active core/shell nanoparticles self-assembled from cholesterol-terminated PEG-TAT for drug delivery across the blood–brain barrierBiomaterials2008291509151718155137