Abstract
Objective
The objective of the present study was to determine the ability of cerium oxide (CeO2) nanoparticles to protect against monocrotaline (MCT)-induced hepatotoxicity in a rat model.
Method
Twenty male Sprague Dawley rats were arbitrarily assigned to four groups: control (received saline), CeO2 (given 0.0001 nmol/kg intraperitoneally [IP]), MCT (given 10 mg/kg body weight IP as a single dose), and MCT + CeO2 (received CeO2 both before and after MCT). Electron microscopic imaging of the rat livers was carried out, and hepatic total glutathione (GSH), glutathione reductase (GR), glutathione peroxidase (GPX), glutathione S-transferase (GST), superoxide dismutase (SOD), and catalase (CAT) enzymatic activities were quantified.
Results
Results showed a significant MCT-induced decrease in total hepatic GSH, GPX, GR, and GST normalized to control values with concurrent CeO2 administration. In addition, MCT produced significant increases in hepatic CAT and SOD activities, which also ameliorated with CeO2.
Conclusions
These results indicate that CeO2 acts as a putative novel and effective hepatoprotective agent against MCT-induced hepatotoxicity.
Introduction
A recent increase in interest in and use of bioreactive nanoparticles represents a new era in the intersection of nanotechnology and biotechnology. These studies have revealed a growing realization of the potential utility of novel environmentally benign technologies in diagnosis and therapeutic use in biological systems.
Most recently, cerium oxide (CeO2) nanoparticles have been tested for their ability to serve as free radical scavengersCitation1,Citation2 to provide protection against chemical, biological, and radiological insults that promote the production of free radicals. The chemistry of engineered CeO2 nanoparticles supports its potential role as a safe and effective biological free radical scavenger or antioxidant. The intracellular CeO2 nanoparticles promote cell longevity and decrease toxic insults by virtue of their antioxidant effects,Citation3 preventing the accumulation of reactive oxygen species (ROS) and reducing the activation of the apoptotic response and death of the cells.Citation4 In previous studies, CeO2 nanoparticles showed no toxic effect on normal breast epithelial (CRL 8798) cells and only a slight effect on breast cancer (MCF-7) cells at concentrations >50 nM.Citation5 Furthermore, CeO2 selectively conferred radioprotection to the normal cells (CRL 8798) as compared with the tumor cells (MCF-7).Citation5
In this study, CeO2 was chosen because of its free radical scavenging activity.Citation6 The metal oxide is a nonstoichiometric compound with the cerium atom characterized by both +4 and +3 oxidation states and possesses a cubic fluorite structure. Recent research using X-ray photoelectron spectroscopy and X-ray absorption near-edge spectroscopy suggests that the concentration of Ce3+ relative to Ce4+ increases as particle size decreases, with a conservative (Ce3+) minimum of 6% in 6 nm nanoparticles and 1% in 10 nm particles.Citation7 This dual oxidation state means that these nanoparticles have oxygen vacancies.Citation8 The loss of oxygen and the reduction of Ce4+ to Ce3+, shown in , are accompanied by creation of an oxygen vacancy. This property is responsible for the interesting redox chemistry exhibited by ceria nanoparticles and makes them attractive for catalytic applications.
Monocrotaline (MCT), a plant-derived pyrrolizidine alkaloid (PA), causes oxidative veno-occlusive disease of the liver, which is thought to be predominantly due to the hepatic formation of a pyrrolic metabolite. Several studies have shown that this metabolite may be detoxified by conjugation with the free radical scavenger reduced glutathione (GSH).
Human exposure occurs from consumption of contaminated grains, herbal teas, and medicines. Intraperitoneal (IP) injection of MCT in rats produced time-dependent hepatic parenchymal cell injury beginning at 12 h.Citation9 Thus, the animal and human health risk posed by exposure to PAs is of great concern.Citation4
To date, about 660 PAs and their N-oxide derivatives have been identified, and at least half are toxic.Citation10,Citation11 There are three major types of PAs: heliotridenes, retronecines, and otonecines. In general, many studies consider that PAs should be metabolized in vivo, predominantly in the liver through cytochrome P450 enzyme systems to form toxic pyrrole metabolites ().Citation12,Citation13
Figure 2 Nomenclatures and structures of the tumorigenic retronecine-type pyrrolizidine alkaloids.Citation13

MCT is a retronecine-type PA that is present in various species of leguminous Crotalaria plants. MCT exposure has been responsible for numerous outbreaks of poisoning worldwide. Citation14 Typically, exposed people develop hepatomegaly and veno-occlusive disease of the liver. In nonhuman primates and a variety of other species, MCT also causes pulmonary arterial hypertension and right ventricular hypertrophy. Citation15 MCT undergoes hepatic bioactivation to the reactive pyrrole dehydromonocrotaline. It is believed that the release of reactive dehydromonocrotaline from the liver is responsible for toxicity to extrahepatic organs, such as the heart and lungs. Dehydromonocrotaline is detoxified by conjugation with GSH.Citation16 Thus, the toxicity of MCT is affected by the GSH status of the liver. MCT, in turn, influences the metabolism of GSH and related sulfur-containing compounds.Citation17 Within 24 h of exposing rats to MCT or related PAs, there is a change in sulfur amino acid metabolism from the cysteine–taurine axis to the cysteine + GSH axis.Citation17,Citation18 Many studies report a marked decrease in the hepatic GSH level in rats treated with MCT when compared with the control group.Citation19
Dehydromonocrotaline can alkylate cell macromolecules in the liver, with such alkylation probably representing the bio-chemical basis of its toxicity.Citation20,Citation21 It can also be released into the circulation to bind covalently to macromolecules in extrahepatic organs.Citation17,Citation20 The amount of dehydromonocrotaline available for these presumably intoxicating pathways is affected markedly by the GSH content of the liver.Citation22 GSH conjugates with dehydromonocrotaline to form glutathione dehydropyrrolizidine (GSDHP), a compound of much lower toxicity that is released in high concentration into the bile.Citation20 Sulfur amino acids, such as methionine and cysteine, that elevate hepatic GSH content also protect against PA toxicity.Citation23,Citation24
Nanoparticles may offer a novel therapeutic alternative for scavenging environmentally elevated ROS. In this study, the use of nanoparticle-based antioxidants as a potential treatment for hepatotoxicity, which is a life-threatening problem, was explored. One obvious use of the nanoparticles would be for enhancing the performance of antioxidants. Therefore, the aims of this study were to design a rat model for hepatotoxicity and to determine the extent to which rare earth CeO2 nanoparticles safeguard against MCT-induced hepatotoxicity in the model.
Material and methods
This study was approved by the Committee of Scientific Ethics at Beni-Suef University, Egypt, and was carried out in accordance with its guidelines for animal use.
Chemicals
MCT was purchased from Toronto Research Chemicals Inc. (North York, Canada) in a synthetic form (MCT pyrrole, 3,8-didehydromonocrotaline; C16H21NO6). CeO2 nanoparticles (>25 nm particle size, 10 wt% in H2O) were obtained from Sigma-Aldrich (St Louis, MO, USA). GSH and glutathione S-transferase (GST) assay kits were obtained from Sigma- Aldrich. Glutathione reductase (GR), catalase (CAT), glutathione peroxidase (GPX), and superoxide dismutase (SOD) assay kits were purchased from Fisher Scientific Company (LLC, San Diego, CA, USA).
Animals
Twenty male Sprague Dawley rats (n = 5 per group; housed two to three per cage) were acclimated for 2 weeks prior to the study, at optimal temperature (22°C), light (14–10 h light–dark schedule), and humidity (40%–60%).
Treatment protocol
Following acclimation, rats were arbitrarily assigned to one of the four following treatment groups, dosed, and euthanized by carbon dioxide asphyxiation 24 h following final injection: 1) control: rats in this group received a single dose of sterile phosphate-buffered physiological saline (PBS; 0.5 mL IP); 2) CeO2: rats in this group received CeO2 (0.00001 mg/kg; 0.5 mL in PBS IP) on days 1 and 3; 3) MCT: rats in this group received a single dose of MCT (10 mg/kg body weight in 0.5 mL PBS IP); and 4) MCT + CeO2: rats in this group received CeO2 (as before) on days 1, 3, 5, and 7 and a single dose of MCT (as before) on day 4. Concurrent CeO2 (0.0001 nmol/kg in 0.5 mL PBS IP) was administered both before and after MCT administration. MCT with or without concurrent CeO2 effects was evaluated.
Hepatic cytosolic and mitochondrial extract preparation
The liver was rinsed with cold PBS to remove excess blood. Small portions of the liver from CeO2 treatment groups were preserved in glutaraldehyde and subjected to electron microscopic examination using conventional methods. This was performed to ensure homogeneous distribution of ceria and to demonstrate no adverse effects of CeO2 alone on hepatocellular health and architecture. The remaining liver samples from each treatment group were minced in 10% (w/v) 0.1 M PBS (pH 7.4) and homogenized using a Teflon Homogenizer (Tissue Tearor, BioSpec Products Inc, Bartlesville, OK, USA). The homogenate was centrifuged at 4000 rpm for 30 min at 4°C to separate supernatant from cellular debris. The supernatant was then used for the estimation of GSH, GR, GST, SOD, CAT, and GPX.
Statistical analysis
Statistical analysis was carried out using GraphPad InStat software (version 3, ISS, Rome, Italy), and one-way analysis of variance followed by Tukey–Kramer multiple comparison post hoc test were used to establish significant differences between groups (see ).
Table 1 Changes in hepatic cytosolic and mitochondrial GR, GSH, GST, and GPX activities in different groups
Table 2 Changes in hepatic CAT and SOD in different groups
Results
Electron microscopic examination of liver samples from rats receiving CeO2 alone demonstrated a homogeneous intrahepatocellular distribution of nanoparticles (–) without phenotypic alteration of hepatocellular architecture. Liver samples obtained from the CeO2 + MCT group also demonstrated regular intracellular distribution of nanoparticles and, importantly, did not exhibit alterations in cellular morphology, which is likely to be due to CeO2 protection against MCT-elevated oxidative damage to the liver.
Figure 3A 1) Regular distribution of cerium oxide (CeO2) nanoparticles intracellular in the endosomes. 2) Homogenous appearance of cytoplasm with no abnormal vaculation, original magnification ×12,500. 3) Regular distribution of CeO2 nanoparticles within normal lysosomes.
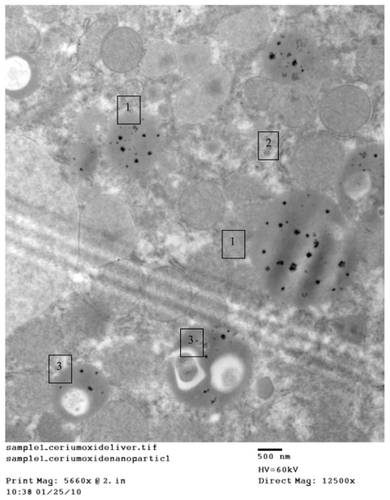
Figure 3B 1) Hepatocytes organelles (mitochondria) showing normal structure. 2) Regular distribution of cerium oxide nanoparticles with homogenous size all over the cytoplasm, original magnification ×5000.
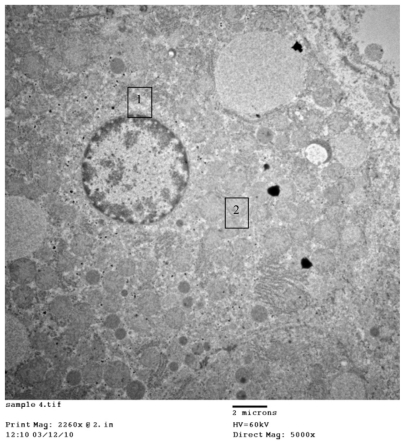
Figure 3C 1) Even distribution of cerium oxide over the cytoplasm of the hepatocytes. 2) Normal ribosomes (rough endoplasmic reticulum), original magnification ×8000.
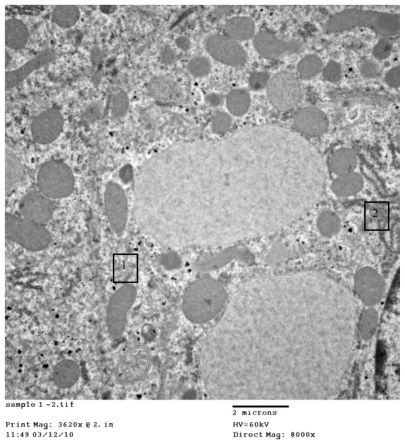
shows CeO2 nanoparticles intracellularly in the endosomes, cytoplasm with no abnormal vaculation, and regular distribution of CeO2 within normal lysosomes. illustrates hepatocyte organelles (mitochondria) with a normal structure and regular distribution of CeO2 with homogenous size all over the cytoplasm; furthermore, demonstrates normal ribosomes (rough endoplasmic reticulum).
Changes of hepatic oxidative/antioxidative parameters
Changes in hepatic cytosolic and mitochondrial GSH, GR, GPXs, and GST activities
Rats given a single dose of MCT showed a significant decrease in total GSH, as well as GR, GPX, and GST activities compared with the control group. Concurrent administration of CeO2 + MCT restored total GSH, GR, and GST activities to near control levels, suggesting that CeO2 may serve as an effective therapy against hepatic oxidative damage caused by MCT ().
Changes in hepatic CAT and SOD activities
Rats given a single dose of MCT showed a significant increase in hepatic CAT and SOD activities compared with control rats; concurrent administration of CeO2 before and after MCT, as previously described, similarly normalized CAT and SOD to near control levels ().
Visual effects of systemic nanoparticle application on rat models
No visible toxicity was observed for the route of administration, and beneficial properties were observed for the nanoparticle treatments as well.
Discussion
MCT is a toxic PA that is globally distributed and found naturally in arid plants such as Crotalaria spp. Toxicity is caused by bioactivation of MCT in the liver to the reactive alkylating pyrrole dehydromonocrotaline. Such a mechanism was suggested in 1968 by MattocksCitation25 and was recently verified with the isolation of dehydromonocrotaline from incubations of rat liver microsomes.Citation26
Dehydromonocrotaline is released from isolated liver samples perfused with MCT, which is believed to contribute to additional extrahepatic toxicity.Citation21 Dehydromonocrotaline has the ability to alkylate cell macromolecules and causes hepatic and extrahepatic oxidative cellular damage. Synthetic dehydromonocrotaline reproduces the toxicity of MCT.Citation27,Citation28
In an isolated liver, dehydromonocrotaline readily conjugates with GSH to form the less toxic secondary metabolite GSDHP,Citation22 which is excreted in high concentrations into the bile. MCT (0.5 mM) also induces a 30-fold increase in the biliary excretion of GSH in an isolated, perfused liver, which depletes hepatic GSH stores.Citation29 As a result, GSH levels fall in the MCT-exposed rat liver.Citation30
Oxidative stress occurs due to an imbalance of oxidants and antioxidants and can be quantified by evaluation of the activity of a panel of antioxidant-related enzymes. GPX is a selenium-containing antioxidative enzyme that widely exists across species and can cause detoxification of toxic superoxide to nontoxic hydroxyl compound through changing reduced GSH to oxidant glutathione.Citation31 CAT primarily exists in the peroxisomes of aerobic cells and serves to protect cells against the toxicity of hydrogen peroxide by catalyzing its decomposition into molecular oxygen and water without producing toxic free radicals. A recent study demonstrated that CAT is a classical oxidative biomarker and is the most abundant protein in peroxisomes, where oxidative stress most frequently occurs.Citation32 Increases in these enzyme activities suggest a response toward increased ROS generation.Citation33
The results obtained in this study concur with other studies suggesting that increased CAT and SOD () are common cellular defense mechanisms against ROS and oxidative stressors. These enzymes are also considered to be sensitive biomarkers for hepatic oxidative stressors.Citation34 Cytosolic GSTs are found in almost all aerobic species and have the capacity to catalyze the conjugation of electrophilic compounds with GSH. GSTs were responsible for detoxification of exogenous substances, which suggests GST as a major target of toxicity of exogenous substances.Citation35
The results obtained show a marked decrease in GST activity, which highlights GST as a major cellular defense mechanism against ROS.Citation36 GR is responsible for the regeneration of GSH, and GPX works together with GSH in disintegrating hydrogen peroxide and other organic hydroperoxide.Citation34
In this study, it was shown that the administration of MCT has a direct effect on the enzymes involved in the metabolism of GSH. The administration of MCT was found to cause a significant decrease in the GR and GPX activities (), which is confirmed by the marked fall in cytosolic and mitochondrial GSH levels (). In addition, a significant increase in GPX activity in the CeO2 group may be due to the antioxidant effects of CeO2 nanoparticles, which cause a marked increase in hepatic GPXs.
Nanotechnology is a multidisciplinary field that involves the design and engineering of objects <100 nm in diameter. Nanoparticles constitute a new generation of free radical scavengers and the chemistry of engineered CeO2 nanoparticles supports their potential role as biological free radical scavengers or antioxidants.
This study suggests that these nanoparticles may represent a novel therapeutic regenerative material that scavenges ROS caused by exogenously elevated ROS due to MCT exposure. When ROS are produced at high levels, cellular components are damaged. These ROS can positively affect biological systems as a defense mechanism against microorganisms and can act as signal transduction and transcription agents in development, stress responses, and programmed cell death. However, excessive oxidative stress arises from the strong cellular oxidizing potential of excess ROS, or free radicals, and has widespread adverse effects in multiple organ systems, including hepatocellular damage, increased risk of cataracts, cardiovascular disease, and cancer.Citation37
CeO2 has a protective effect against radiation-induced oxidative damage and pneumonitis, although it has the ability to scavenge oxygen free radicals and ROS.Citation37 In addition, CeO2 nanoparticles offer many active sites for free radical scavenging because of their large surface/volume ratio and also the mixed valence states for unique redox chemistry. A recent article reports SOD mimetic activity of CeO2.Citation38 Furthermore, the free radical scavenging property of CeO2 nanoparticles is regenerative, which is not the case for other antioxidants.Citation39 The chemical nature of CeO2 nanoparticles, in which an autoregenerative reaction cycle (Ce3+ → Ce4+ → Ce3+) continues on the surface, is probably the mechanism by which the material gains an unprecedented free radical scavenging ability.Citation37
CeO2 nanoparticles have been revealed to effectively protect mammalian cells against damage caused by increased ROS or nitrogen species, probably through their direct reaction with superoxide radicals, because each of these materials has been shown to act as an effective SOD mimetic in vitro.Citation40
Dehydromonocrotaline has the ability to alkylate cell macromolecules and cause hepatic and extrahepatic oxidative cellular damage. Synthetic dehydromonocrotaline reproduces the toxicity of MCT by formation of ROS.Citation27,Citation28
Results show that administration of CeO2 before and after MCT administration exerts an important protective effect as it corrects the oxidative stresses induced by administration of MCT. The experiments discussed here show that CeO2 nanoparticles were able to rescue cells from oxidative stress-induced cell damage in a manner that appears to be dependent on the structure of the particle but independent of its size within the 6–1000 nm range. There are three alternative explanations for the observation that the CeO2 particles protect from oxidative stress.Citation41 They may act as direct antioxidants, block ROS production by inhibiting a step in the programmed cell death pathway, or directly cause a low level of ROS production that rapidly induces an ROS defense system before the glutamate-induced cell death program is complete. The last is a form of preconditioning that could be caused by the exposure of cells to particulate material known to induce low levels of ROS.Citation42
The results obtained in this study were the first to demonstrate that CeO2 nanoparticles induce hepatoprotective biological responses and could be properly indicated by using a test approach such as oxidative stress.
From the current data, it can be concluded that CeO2 could be used to modulate oxidative stress and has a protecting effect against the hepatotoxicity induced by MCT administration.
Acknowledgment
This study was supported by a fund from Beni-Suef University, Egypt. We appreciate the help and advice of Dr, Prater R, VT.
Disclosure
The authors report no conflicts of interest in this work.
References
- ChenJPatilSSealSMcGinnisJFRare earth nanoparticles prevent retinal degeneration induced by intracellular peroxidesNat Nanotechnol20061214215018654167
- RzigalinskiBABaileyDChowLCerium oxide nanoparticles increase the lifespan of cultured brain cells and protect against free radical and mechanical traumaFASEB J2003174A606
- PatilSSandbergAHeckertESelfWSealSProtein adsorption and cellular uptake of cerium oxide nanoparticles as a function of zeta potentialBiomaterials200728314600460717675227
- FuPPXiaQLinGChouMWPyrrolizidine alkaloids – genotoxicity, metabolism enzymes, metabolic activation, and mechanismsDrug Metab Rev200436115515072438
- TarnuzzerRWColonJPatilSSealSVacancy engineered ceria nanostructures for protection from radiation-induced cellular damageNano Lett20055122573257716351218
- ChungDNanoparticles have health benefits tooNew Scientist200317924102416
- ZhangFWangPKobersteinJKhalidSChanSWCerium oxidation state in ceria nanoparticles studied with X-ray photoelectron spectroscopy and absorption near edge spectroscopySurf Sci2004563137482
- RobinsonRDSpanierJEZhangFChanSWHermanIPVisible thermal emission from sub-band-gap laser excited cerium dioxide particlesJ Appl Phys200292419361941
- CoppleBLGaneyPERothRALiver inflammation during monocrotaline hepatotoxicityToxicology2003190315516912927372
- StegelmeierBLEdgarJAColegateSMPyrrolizidine alkaloid plants, metabolism and toxicityJ Nat Toxins1999819511610091131
- RoederEMedicinal plants in China containing pyrrolizidine alkaloidsPharmazie2000551071172611082830
- GordonGJColemanWBGrishamJWInduction of cytochrome P450 enzymes in the livers of rats treated with the pyrrolizidine alkaloid retrorsineExp Mol Pathol2000691172610891289
- XiaQYanJChouMWFuPPFormation of DHP-derived DNA adducts from metabolic activation of the prototype heliotridine-type pyrrolizidine alkaloid, heliotrineToxicol Lett20081782778218395999
- HuxtableRJHuman health implications of pyrrolizidine alkaloids and herbs containing themCheekePRToxicants of Plant Origin. Vol 1 AlkaloidsBoca Raton (FL)CRC Press19894186
- HuxtableRJHepatic nonaltruism and pulmonary toxicity of pyrrolizidine alkaloidsGramTEMetabolic Activation and Toxicity of Chemical Agents to Lung Tissue and CellsNew YorkPergamon Press1993215239
- MattocksARCroswellSJukesRHuxtableRJIdentity of a biliary metabolite formed from monocrotaline in isolated, perfused rat liverToxicon19912945409415
- YanCCHuxtableRJThe effect of the pyrrolizidine alkaloids, monocrotaline and trichodesmine, on tissue pyrrole binding and glutathione metabolism in the ratToxicon19953356276347660367
- YanCCHuxtableRJEffects of the pyrrolizidine alkaloid, retrorsine, on sulfur metabolism, in the liverProc West Pharmacol Soc19953837407480015
- YanCCHuxtableRJEffects of taurine and guanidinoethane sulfonate on toxicity of the pyrrolizidine alkaloid monocrotalineBiochem Pharmacol19965133213298573199
- YanCCHuxtableRJQuantitation of the hepatic release of metabolites of the pyrrolizidine alkaloid, monocrotalineToxicol Appl Pharmacol1994127158638048054
- YanCCHuxtableRJThe relationship between the concentration of the pyrrolizidine alkaloid monocrotaline and the pattern of metabolites released from the isolated liverToxicol Appl Pharmacol19951301187839357
- YanCCHuxtableRJRelationship between glutathione concentration and metabolism of the pyrrolizidine alkaloid, monocrotaline, in the isolated, perfused liverToxicol Appl Pharmacol199513011321397839360
- CheekePRGarmanGRInfluence of dietary protein and sulfur aminoacid levels on the toxicity of Senecio jacobaea (tansy ragwort) to ratsNutr Rep Int19749197207
- MirandaCLBuhlerDRRamsdellHSCheekePRSchmitzJAModifications of chronic hepatotoxicity of pyrrolizidine (Senecio) alkaloids by butylated hydroxyanisole and cysteineToxicol Lett19821023177182
- MattocksARToxicity of pyrrolizidine alkaloidsNature196821751307237285641123
- GlowazSLMichnikaMHuxtableRJDetection of a reactive pyrrole in the hepatic metabolism of the pyrrolizidine alkaloid, monocrotalineToxicol Appl Pharmacol199211521681731641851
- BrunerLHHillikerKSRothRAPulmonary hypertension and ECG changes from monocrotaline pyrrole in the ratAm J Physiol19832452H3003066224428
- PanLCWilsonDWLaméMWJonesADSegallHJCOR pulmonale is caused by monocrotaline and dehydromonocrotaline, but not by glutathione or cysteine conjugates of dihydropyrrolizineToxicol Appl Pharmacol1993118187978430429
- YanCCHuxtableRJEffect of the pyrrolizidine alkaloid, monocrotaline, on bile composition of the isolated, perfused rat liverLife Sci19955766176267623629
- NigraLHuxtableRJHepatic glutathione concentrations and the release of pyrrolic metabolites of the pyrrolizidine alkaloid, monocrotaline, from the isolated perfused liverToxicon19923010119512021440625
- ArthurJRThe glutathione peroxidasesCell Mol Life Sci200057131418251835
- BocchettiRRegoliFSeasonal variability of oxidative biomarkers, lysosomal parameters, metallothioneins and peroxisomal enzymes in the Mediterranean mussel Mytilus galloprovincialis from Adriatic SeaChemosphere200665691392116678235
- KyleMEMiccadeiSNakaeDFarberJLSuperoxide dismutase and catalase protect cultured hepatocytes from the cytotoxicity of acetaminophenBiochem Biophys Res Commun198714938898963122747
- LeeCPShihPHHsuCLYenGCHepatoprotection of tea seed oil (Camellia oleifera Abel.) against CCl4-induced oxidative damage in ratsFood Chem Toxicol200745688889517188414
- CheekeRPToxicity and metabolism of pyrrolizidine alkaloidsJ Anim Sci1988669234323503049495
- LiuTYChenYWangZYJiLLWangZTPyrrolizidine alkaloid isoline-induced oxidative injury in various mouse tissuesExp Toxicol Pathol201062325125719540740
- ColonJHerreraLSmithJProtection from radiation-induced pneumonitis using cerium oxide nanoparticlesNanomedicine20095222523119285453
- HeckertEGKarakotiASSealSSelfWTThe role of cerium redox state in the SOD mimetic activity of nanoceriaBiomaterials200829182705270918395249
- DasMPatilSBhargavaNAuto-catalytic ceria nanoparticles offer neuroprotection to adult rat spinal cord neuronsBiomaterials200728101918192517222903
- KarakotiASinghSDowdingJMSealSSelfWTRedox-active radical scavenging nanomaterialsChem Soc Rev201039114422443220717560
- SchubertDDarguschRRaitanoJChanSWCerium and yttrium oxide nanoparticles are neuroprotectiveBiochem Biophys Res Commun20063421869116480682
- BeckerSSoukupJMGallagherJEDifferential particulate air pollution induced oxidant stress in human granulocytes, monocytes and alveolar macrophagesToxicol In Vitro200216320921812020593