Abstract
Background
Nucleobase-bearing peptides and their interaction with DNA and RNA are an important topic in the development of therapeutic approaches. On one hand, they are highly effective for modulating the nucleic-acid-based biological processes. On the other hand, they permit to overcome some of the main factors limiting the therapeutic efficacy of natural oligonucleotides, such as their rapid degradation by nucleases.
Methods and results
This article describes the synthesis and characterization of a novel thymine-bearing nucleoamino acid based on the l-diaminopropionic acid (l-Dap) and its solid phase oligomerization to α-peptides (oligoDapT), characterized using mass spectrometry, spectroscopic techniques, and scanning electron microscopy (SEM) analysis. The interaction of the obtained nucleopeptide with DNA and RNA model systems as both single strands (dA12, rA12, and poly(rA)) and duplex structures (dA12/dT12 and poly(rA)/poly(rU)) was investigated by means of circular dichroism (CD) and ultraviolet (UV) experiments. From the analysis of our data, a clear ability of the nucleopeptide to bind nucleic acids emerged, with oligoDapT being able to form stable complexes with both unpaired and double-stranded DNA and RNA. In particular, dramatic changes in the dA12/dT12 and poly(rA)/poly(rU) structures were observed as a consequence of the nucleopeptide binding. CD titrations revealed that multiple peptide units bound all the examined nucleic acid targets, with TLdap/A or TLdap/A:T(U) ratios >4 in case of oligoDapT/DNA and ~2 in oligoDapT/RNA complexes.
Conclusion
Our findings seem to indicate that Dap-based nucleopeptides are interesting nucleic acid binding-tools to be further explored with the aim to efficiently modulate DNA- and RNA-based biological processes.
Introduction
A large number of chimeric compounds that bear nucleobases or other types of heteroaromatic rings connected to amino acid-containing moieties were discovered in various natural sources or are the result of synthetic efforts.Citation1–Citation5 Among these, of great interest are nucleoamino acids or nucleobase amino acids (NBAs). The natural (S)- willardiine and its analogs,Citation6,Citation7 for example, act as potent agonists of α-amino-3-hydroxy-5-methylisoxazole-4-propionic acid (AMPA) receptors or kainate receptors, whereas a large number of these synthetic monomers constitute the building blocks for the assembly of synthetic nucleobase-containing peptides (nucleopeptides), investigated for their potential applications in biomedicine and biomaterial fields.Citation8–Citation13
The range of applications of nucleopeptides recalls that of peptide nucleic acids (PNAs), the known DNA analogs, which are achiral, non-charged oligomers with a pseudo-peptide backbone, largely used in numerous diagnostic, antigene, and antisense strategies.Citation14–Citation20 The presence of both nucleobases and peptide backbone in nucleopeptides offers the possibility of binding nucleic acids or protein targets, modulating important cell regulatory processes, such as the viral reverse transcriptase inhibition,Citation21–Citation23 similar to other oligonucleotide (ODN) systems.Citation24
NBA-containing peptides were used to select nucleic acid-related proteins derived from Saccharomyces cerevisiae and to detect the peptide/protein interactions on T7 phages displaying proteins.Citation25 Moreover, the ability of nucleopeptide to deliver ODNs into cells was recently demonstrated treating HeLa cells with NBA-containing peptides incubated in the presence of a fluorescent Cy5-labeled hairpin DNA. Fluorescence microscopy studies evidenced how nucleopeptides deliver hairpin DNA to the cytosols of live cells, while cell viability assays showed their biocompatibility even at high dosages after 72 h of treatment.Citation26
Despite all their favorable applications, one of the existing problems in the development of synthetic ODN analogs is the nonspecific interaction with DNA and RNA. A previous study reported that inserting short (R)-diethylene glycol (miniPEG) moieties in the PNA backbone can improve the specificity of the interaction.Citation27 Alternatively, the insertion of chiral amino acids, possibly positively charged, into the PNA backbone provided both an improved water solubility and chirality, which has proven crucial in conferring high-sequence selectivity to artificial ODNs.Citation8,Citation27
Overall, the important role that NBA-containing structures play in biomedicine, as well as the possibility to realize new DNA- and RNA-binding nucleopeptides, prompted us to realize and investigate a new nucleobase-decorated artificial peptide, whose repeating unit is composed of a dipeptide moiety, based on the l-diaminopropionic acid (l-Dap), connected via a short linker to the thymine nucleobase ().
The oligomerization of the building block depicted in leads to homothymine α-peptides (oligoDapT; ) in which the base-carrying l-Dap residues are alternated in the sequence with unmodified l-Dap, affording a cationic oligomer as a result of the protonation of the side chain amino groups at physiological pH. Thus, in addition to favorable H-bonding, potential electrostatic interactions are originated between oligoDapT and nucleic acids ().
We here describe a convenient synthesis of oligoDapT, as well as the evaluation of its biomolecular recognition properties toward both single- and double-stranded DNA and RNA targets assessed by circular dichroism (CD) and ultraviolet (UV) spectroscopies.
Materials and methods
Materials
O-(7-aza-1H-Benzotriazol-1-yl)-N,N,N′,N′-tetramethyluronium hexafluorophosphate (HATU) and benzotriazol-1-yl-oxytripyrrolidinophosphonium hexafluorophosphate (PyBOP) were purchased from Novabiochem (Merck S.p.a., Milan, Italy), 9-fluorenylmethoxycarbonyl (Fmoc)-l-Dap (tert-butoxycarbonyl [Boc])-OH was purchased from Bachem (Weil am Rhein, Germany). Anhydrous N,N-dimethylformamide (DMF) was obtained from Labscan (Gliwice, Poland); piperidine from Biosolve (Valkenswaard, The Netherlands); high-performance liquid chromatography (HPLC) solvents and acetic anhydride (Ac2O) from Riedel-de Haën (Seelze, Germany); poly(rA), thymin-1-yl acetic acid (TCH2COOH), trifluoroacetic acid (TFA), 2,4,6-trimethylpyridine (TMP), and Rink-amide resin from Fluka (München, Germany); dichloromethane (DCM) and TFA (for HPLC) from Romil (Cambridge, UK); dA12 from Biomers.net GmbH (Ulm, Germany); and deuterated dimethyl sulfoxide (DMSO) from Sigma-Aldrich Co. (Milan, Italy).
1H NMR (nuclear magnetic resonance) and 13C NMR spectra were recorded at 25°C on a Varian 600 MHz (150 MHz) unit. Chemical shifts (δ) are given in parts per million (ppm), and all coupling constants (J) are given in hertz. 1H NMR chemical shifts are referenced to residual CHD2SOCD3 (δ = 2.49, quin). 13C NMR chemical shifts are referenced to the solvent (CD3SOCD3: δ = 39.5). Samples were centrifuged at 4,000 rpm on a Z 200 A centrifuge (Hermle from Del Chimica, Napoli, Italy).
Samples of both monomer and nucleopeptides underwent liquid chromatography–mass spectrometry (LC-MS) analysis ( and , respectively) on an MSQ mass spectrometer (ThermoElectron, Milan, Italy) equipped with an electrospray ionization (ESI) source operating at 3 kV needle voltage and 320°C and with a complete Surveyor HPLC System, comprising an MS pump, an autosampler, and a photodiode array (PDA) detector, by using a Phenomenex (Castel Maggiore, Italy) Jupiter C18 300 Å (5 μm, 4.6 × 150 mm) column. We performed the gradient elution at 25°C (monitoring at 260 nm) building up a gradient starting with buffer A (0.05% TFA in water) and applying buffer B (0.05% TFA in acetonitrile) with a flow rate of 0.8 mL·min−1. We performed the semipreparative purifications on a Hewlett-Packard/Agilent 1100 series HPLC system (Agilent Technologies, Santa Clara, CA, USA), equipped with a PDA detector, and used a Phenomenex Jupiter C18 300 Å (10 μm, 4.6 × 250 mm) column. Gradient elution was performed at 25°C (monitoring at 260 nm) by using a gradient that started with buffer A’ (0.1% TFA in water) and applying buffer B’ (0.1% TFA in acetonitrile) with a flow rate of 4 mL·min−1. Samples were lyophilized from water in an FD4 freeze dryer (Heto Lab Equipment, Birkerød, Denmark) for 16 h.
Synthetic procedures
Synthesis of monomer 3
Commercial Fmoc/Boc-protected 2,4-diaminopropionic acid 1 (Fmoc-l-Dap(Boc)-OH: 50 mg, 0.12 mmol; ) was treated with a 1:1 TFA/DCM solution (2 mL) at 45°C, and the mixture was stirred for 1.5 h. Afterward, the solvent was removed in vacuo, and the crude mixture was treated with cold diethyl ether. After centrifugation, a white precipitate was recovered by filtration and repeated washings with diethyl ether, and was dried in vacuo. This product was dissolved in anhydrous DMF (1 mL) treated with N,N-diisopropylethylamine (DIEA; 0.9 equiv., 19 μL, 0.11 mmol) and TMP (0.6 equiv., 9 μL, 0.07 mmol), and then reacted with TCH2COOH (2: 2.2 equiv., 49 mg, 0.26 mmol), previously preactivated with HATU (2.0 equiv., 91 mg, 0.24 mmol) and DIEA (2.0 equiv., 42 μL, 0.24 mmol)/TMP (2.0 equiv., 32 μL, 0.24 mmol) in DMF (1 mL) for 2 min.
Figure 2 Schematic representation of the synthesis of the nucleobase-decorated peptides.
Abbreviations: Boc, tert-butoxycarbonyl; Fmoc, 9-fluorenylmethoxycarbonyl; TFA, trifluoroacetic acid; DCM, dichloromethane; HATU, O-(7-aza-1H-benzotriazol-1-yl)-N,N,N′,N′-tetramethyluronium hexafluorophosphate; DIEA, N,N-diisopropylethylamine; DMF, N,N-dimethylformamide; PyBOP, benzotriazol-1-yl-oxytripyrrolid-inophosphonium hexafluorophosphate; Ac2O, acetic anhydride; TIS, triisopropylsilane.
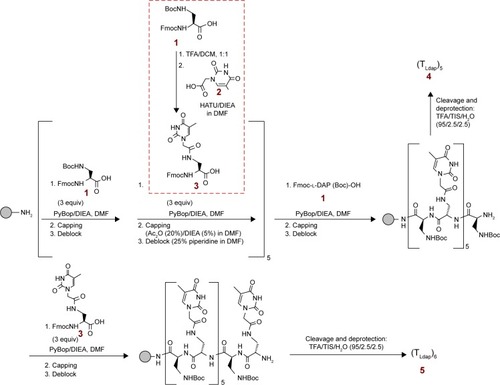
After 1.5 h, the solvent was removed in vacuo. The crude material was treated with water, sonicated, and filtered in order to remove the aqueous solution from the white precipitate. This solid was purified by semipeparative HPLCCitation8 and the desired product (3) was obtained as a pure compound in good yield (47 mg, 0.25 mmol, 81% yield): LC-ESI-MS () m/z 493.92 (found), 493.50 (expected for [C25H24N4O7+H]+ =[M+H]+); m/z 515.85 (found), 515.48 (expected for [C25H24N4O7+Na]+); m/z 986.06 (found), 985.99 (expected for [2(C25H24N4O7)+H]+); δH (600 MHz, DMSO-d6; ) 11.28 (1H, s, NH thymine), 8.28 (1H, bs, NH amide), 7.89 (2H, d, J = 7.2, aromatic protons, CH Fmoc), 7.73 (2H, d, J = 7.8, aromatic protons, CH Fmoc), 7.60–7.33 (6H, m, aromatic protons, CH Fmoc, Fmoc-NH, CH thymine), 4.33–4.11 (6H, m, CH2 linker, FmocCH–CH2 and CHα), and 3.58–3.31 (2H, m, CH2NH), 1.74 (3H, s, CH3 thymine); δC (150 MHz, DMSO-d6; ) 171.97 (COOH), 167.43 (CH2CONH), 164.47 (thymine C-4), 156.08 (OCONH), 151.01 (thymine C-2), 143.80 (aromatic carbons, Fmoc), 142.28 (thymine C-6), 140.75 (aromatic carbons, Fmoc), 127.68 (aromatic carbons, Fmoc), 127.14 (aromatic carbons, Fmoc), 125.29 (aromatic carbons, Fmoc), 120.15 (aromatic carbons, Fmoc), 108.02 (thymine C-5), 65.79 (Fmoc CH2), 53.77 (CHα), 49.14 (CH2 acetyl linker), 46.62 (Fmoc CH), 39.92 (CH2NH), and 11.93 (thymine CH3).
Nucleopeptide synthesis
Solid-phase synthesis was performed in analogy with other literature reportsCitation28 on a Rink-amide-NH2 resin (0.55 mmol/g, 18 mg). After resin Fmoc cleavage by treatment with 25% piperidine in DMF over 30 min and repeated DMF washings of the resin, a mixture of Fmoc-l-Dap(Boc)-OH 1 or monomer 3 (300 μL of a 0.1 M solution in DMF, 30 μmol, 3 equiv.), PyBOP (50 μL of a 0.6 M solution in DMF, 30 μmol, 3 equiv.), and DIEA (10 μL, 60 μmol, 6 equiv.) was introduced into the reactor containing the free-NH2 resin and stirred for 20 min. Then, the coupling mixture was removed from the resin, and the solid support was washed repeatedly with DMF. Unreacted amino functions were capped by treating the resin with Ac2O (20%)/DIEA (5%) in DMF over 15 min. Finally, Fmoc was removed by treatment with 25% piperidine in DMF over 15 min. This procedure was repeated for the sequential insertion of l-Dap and l-DapT on the solid support. The overall yield, as estimated by UV Fmoc test, was 65% in case of the hexathymine derivative 5, whereas pentathymine nucleopeptide 4 was obtained in 89% yield. The deprotection and detachment of the nucleopeptides from the resin were realized by treatment with TFA/triisopropylsilane/H2O (95%/2.5%/2.5%) over 2 h. After nucleopeptide precipitation with cold diethyl ether, the samples were treated with milliQ H2O and purified by reversed-phase (RP)-HPLC using a gradient (10% [for 5 min] to 30%) of B’ in A’ over 30 min, collecting the peak at tR = 16.8 min.
After HPLC purification, the nucleopeptide samples were dissolved in a known amount of milliQ water and quantified by UV (T = 85°C, absorbance measured at λ = 260 nm). The used molar extinction coefficients of 43,000 cm−1·M−1 (4) and 51,600 cm−1·M−1 (5) were calculated starting from that corresponding to the thymine-containing PNA monomer (ie, 8,600 cm−1·M−1). ESI-MS characterization of oligoDapT 4 (): m/z: 1,795.59 (found), 1,795.76 (expected for [C68H99N33O26+2H]++); 899.72 (found), 898.85 (expected for [C68H99N33O26+3H]+++); and 600.20 (found), 599.26 (expected for [C68H99N33O26+4H]++++) and 5 (): m/z: 1,025.23 (found), 1,024.50 (expected for [C78H111N37O30+2H]++); and 683.24 (found), 683.34 (expected for [C78H111N37O30+3H]+++).
Scanning electron microscopy (SEM) analysis
Samples for microscopic observations were obtained using compounds in solution. After slow solvent evaporation of a droplet taken from a 167 μM solution in H2O, the sample of oligoDapT was coated with a conductive layer of Au–Pd and examined in Nova NanoSem 450 SEM from FEI Technologies Inc. (Hillsboro, OR, USA) at 2 and 3 kV in a high-vacuum mode. DNA (dA12) and RNA (poly(rA))/nucleopeptide complex solutions were obtained from the CD titrations with oligoDapT.
CD and UV studies
CD and UV studies were carried out in analogy with other literature studies.Citation29–Citation31 In particular, we obtained the CD spectra in a 200–320 nm wavelength range at 5°C on a J-810 spectropolarimeter (Jasco Europe S.R.L., Cremella, Italy) equipped with a Peltier PTC-423S/15, using a Hellma (Milan, Italy) tandem quartz cell (2 × 0.4375 cm). UV spectra were recorded on a UV–Vis Jasco model V-550 spectrophotometer equipped with a Peltier ETC-505T temperature controller.
Results and discussion
Synthesis of the thymine-containing peptides
The artificial peptides were obtained following the synthetic procedure described in .
First, we synthesized monomer 3 by reacting commercial Fmoc/Boc-protected l-Dap monomer 1 with TCH2COOH (2) and characterized it by ESI-MS () and NMR spectroscopy ( and ). Subsequently, we used in a sequential manner 3 and 1 in order to synthesize in the solid phase, using Fmoc-chemistry protocol, two oligomers, ie, pentathymine 4 and hexathymine 5 (). Fmoc UV measurements during the synthesis showed general high coupling efficiencies and, thus, overall good yields of the final nucleopeptides. After detachment from the resin and deprotection under acidic conditions, the constructs 4 and 5 were purified by RP-HPLC and characterized by ESI-MS (). The obtained oligomers presented peptide chains in which half of the residues carried the DNA nucleobases, while the others were base free, thus ensuring positive charges to the overall structures under physiological conditions. This led to cationic nucleopeptides, highly soluble in aqueous solution, that had both base–base and ionic (−NH3+/−PO3−) binding possibilities toward natural nucleic acids.
SEM analysis
SEM imaging of dried oligoDapT led to the micrographs shown in .
Figure 3 SEM micrographs of oligoDapT (A–C), oligoDapT/DNA complex (D and E), and oligoDapT/RNA complex (F).
Notes: (A, B, D, E, and F) 100,000×, 1 μm; (C) 200,000×, 500 nm.
Abbreviation: SEM, scanning electron microscopy.
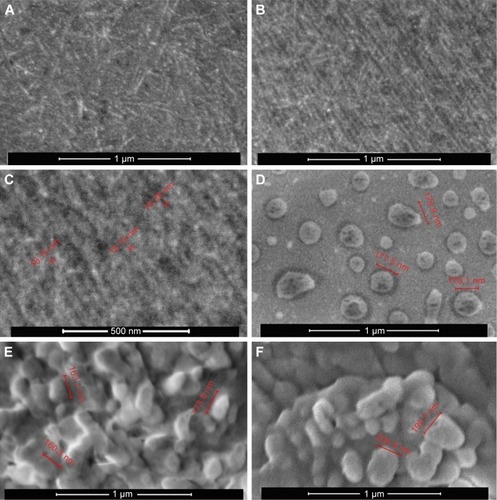
Surface morphology of oligoDapT appears with a low roughness and some thread structures (). Several dense and long thread like structures, fiber-like, clearly appear (). At high magnifications, we found a fiber thickness ranging between 10 and 20 nm () similar to that obtained by SEM, for self-assembling peptides rich in ionic and hydrophobic residues,Citation32 or by TEM for aromatic peptides.Citation33 In analogy with other nucleopeptide systems,Citation26 here the interaction between aromatic moieties (nucleobases) has a role in the formation of the observed fibril-like structures.
Moreover, we analyzed by SEM the peptide/nucleic acid complexes to elucidate both morphology and size of the resulting structures. SEM of oligoDapT/DNA () and oligoDapT/RNA () revealed the absence of thread like structures and the formation of spherical morphologies (width 160–200 nm), in analogy with other literature reports on nucleic acid–peptide complexes or hybrids,Citation34,Citation35 arising from the nucleic acid/nucleopeptide binding, which induces the clustering of several nanofibers into larger aggregates.Citation26
Nucleic acid-binding studies
Afterward, we studied the interaction of oligoDapT with nucleic acids, in both single- and double-stranded forms, by means of CD and UV spectroscopies. We recorded in a two-chamber quartz cuvette the sum of CD and UV spectra relative to the separated solutions of the nucleopeptide (4 μM concentration in thymine) and complementary single-stranded nucleic acids (dA12 DNA, rA12 RNA, and poly(rA) RNA, all at 4 μM concentration in adenine) in 10 mM phosphate buffer (pH 7.5) at 5°C and compared them with those obtained after the abovementioned solutions were mixed. Extensive changes in DNA and RNA CD spectra upon mixing the solutions of the two-cell reservoirs reflected strong oligoDapT/DNA and oligoDapT/RNA interactions (, , and ).
Figure 4 Nucleopeptide/DNA binding assays. Sum (black) and mixture (red) CD (A and C) and UV (B) spectra of oligoDapT (4, 4 μM in T) and dA12 DNA (4 μM in A) in 10 mM phosphate buffer (pH = 7.5; T = 5°C; optical path = 0.875 cm). (C) CD titration relative to the addition of oligoDapT (4) to dA12 DNA at the following TLdap/dA ratios: 2, 3, 4, and 5.
Abbreviations: CD, circular dichroism; UV, ultraviolet.
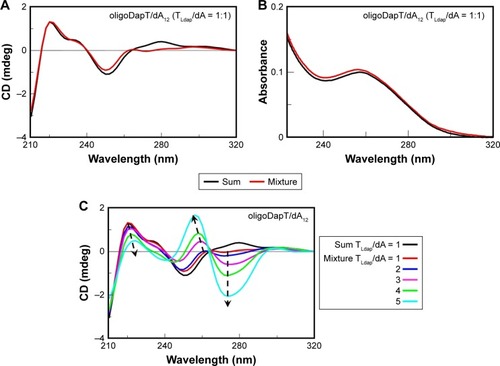
Figure 5 CD (A) and UV (B) analyses at 5°C in a two-chamber cell (optical path = 0.875 cm) relative to the formation of the complex nucleopeptide/rA12: sum spectra (black lines) of oligoDapT (4, 4 μM in TLdap) and RNA (4 μM in rA) solutions in 10 mM phosphate buffer (pH = 7.5) and mixture spectra at TLdap/rA = 1/1 (red) and 2/1 (blue) ratios.
Abbreviations: CD, circular dichroism; UV, ultraviolet.
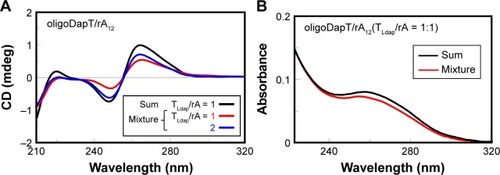
Figure 6 CD (A and C) and UV (B) analyses at 5°C in a two-chamber cell (optical path = 0.875 cm) relative to the formation of the complexes nucleopeptide/poly(rA) (A and B) and nucleopeptide/poly(rU) (C): sum spectra (black lines) of oligoDapT (4, 4 μM in TLdap) and RNA (4 μM in rA or rU) solutions in 10 mM phosphate buffer (pH = 7.5) and mixture spectra at TLdap/rA or TLdap/rU = 1/1 (red) and 2/1 (blue) ratios.
Abbreviations: CD, circular dichroism; UV, ultraviolet.
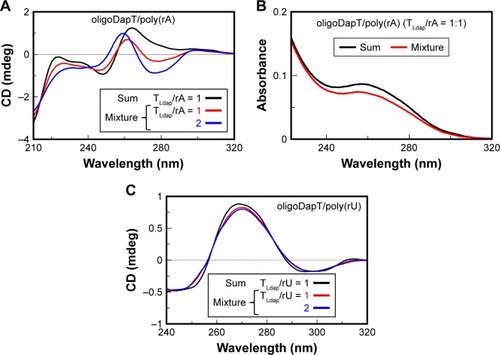
We have found only minor UV changes () for oligoDapT/dA12 mixed in a 1:1 ratio, suggesting a minor role of the base stacking in the formation of the TLdap/dA complex. On the other hand, the analogous oligoDapT/rA12 system largely involved nucleobase stacking as evidenced by the significant UV hypochromic effect (). An even more pronounced hypochromic effect was evidenced when poly(rA) was used instead of rA12 (). This RNA vs DNA selectivity, previously evidenced also for other l-diamino acid-based nucleopeptides by CD and surface plasma resonance (SPR) experiments,Citation36 can be associated with additional H-bonds with RNA structures due to ribose 2′-OH moieties (absent in DNA) that reinforce the overall ODN/nucleopeptide structuring.
By titrating the oligoDapT/DNA solution with further amounts of the nucleopeptide, we observed that the CD profiles underwent further modifications in correspondence of TLdap/dA ratios higher than 2:1, which resulted in dramatic changes in both shape and intensity of the CD bands (). More in detail, a negative band at 274 nm and a positive one at 260 nm appeared, with the 260 nm band showing also a hypsochromic shift of its maximum upon titration (from 260 nm at TLdap/dA = 2 to 256 nm at TLdap/dA = 5). A substantial stabilization of the CD signal was observed at a 5:1 (TLdap/dA) ratio, indicating a multimeric oligoDapT/DNA complex.
Analogously, we performed a titration experiment also with rA12 and poly(rA); the latter is an RNA suggested as an important anticancer target.Citation37,Citation38 The stoichiometry of the obtained oligoDapT/RNA complexes was investigated by adding oligoDapT aliquots to the 1:1 (TLdap/rA) complex solutions until stabilization of the CD signal: for both rA12 and poly(rA), the formation of complexes with a 2/1 = TLdap/rA stoichiometry was evidenced ( and ). However, upon titration with the nucleopeptide, more dramatic conformational changes in the poly(rA) with respect to rA12, reflected in strong CD profile changes, were observed.
To get more insight into the nature of the nucleopeptide/nucleic acid recognition, and in particular to establish the relative contribution of the electrostatic forces and sequence-specific recognition to the interaction, a binding experiment of oligoDapT with a noncomplementary poly(rU) strand was performed, and the obtained spectra were compared with those shown in for the poly(rA). CD experiments showed that the nucleic acid structure of the homouracil RNA underwent only negligible changes upon oligoDapT addition (), in contrast to the dramatic RNA structure perturbation observed for the oligoDapT/poly(rA) binding (). These findings indicate that the interaction is mainly driven by complementary base pairing, with the NH3+/phosphate ionic recognition being only of secondary importance, ie, useful to reinforce the overall interaction but not the major driving force.
The importance of new drugs able to bind double-stranded DNA, as well as to interfere with double-stranded RNA-driven biological processesCitation39–Citation41 or act as stabilizers and carriers of double-stranded RNA drugs,Citation42–Citation46 prompted us to study the interaction of oligoDapT with dA12/dT12 and poly(rA)/poly(rU), chosen as double-stranded DNA and RNA model systems, respectively. First, the target complexes were prepared by mixing equimolar amounts of DNA and RNA single strands, heating the resulting solutions at 90°C for 10 minutes and then slowly cooling overnight. Subsequently, by using a two-chamber quartz cuvette, we recorded and compared the CD spectra relative to the sum of the separated solutions of oligoDapT and double-stranded nucleic acids with those recorded after mixing the solutions and also after further oligoDapT additions. CD changes in the DNA/DNA mixture spectrum were evident when a 1/1 TLdap/[dA:dT] ratio was achieved, with a stabilization of the signal only after further oligoDapT addition, corresponding to a 4/1 TLdap/[dA:dT] ratio. Moreover, a bathochromic shift (4 nm) for the positive band at ~280 nm was observed in the CD titration ().
Figure 7 CD analysis at 5°C in a two-chamber cell (optical path = 0.875 cm): sum (green) spectrum of oligoDapT (4, 4 μM in TLdap) and the DNA duplex dA12:dT12 (5 μM in dA:dT) solutions and mixture spectra at various TLdap/[dA:dT] ratios in PBS buffer (pH 7.5).
Abbreviations: CD, circular dichroism; PBS, phosphate-buffered saline.
![Figure 7 CD analysis at 5°C in a two-chamber cell (optical path = 0.875 cm): sum (green) spectrum of oligoDapT (4, 4 μM in TLdap) and the DNA duplex dA12:dT12 (5 μM in dA:dT) solutions and mixture spectra at various TLdap/[dA:dT] ratios in PBS buffer (pH 7.5).Abbreviations: CD, circular dichroism; PBS, phosphate-buffered saline.](/cms/asset/71440b17-0253-463e-8156-213a4cd41032/dijn_a_12193970_f0007_c.jpg)
More dramatic changes were observed studying the interaction of oligoDapT with the RNA:RNA duplex, with a significant decrease in the overall CD signal intensity and a hypsochromic shift of the positive band at 266 nm, relative to the poly(rA):poly(rU) complex, to 259 nm in the spectrum of the ternary complex oligoDapT/RNA:RNA when a 2/1 TLdap/[rA:rU] ratio was reached (). CD titration experiments suggested the formation of a complex with a 2/1 stoichiometry relative to the TLdap and rA:rU bases, since a certain CD signal stabilization was obtained for this mixture.
Figure 8 CD analysis at 5°C in a two-chamber cell (optical path = 0.875 cm): sum (black) spectrum of oligoDapT (4, 4 μM in TLdap) and the RNA duplex poly(rA):poly(rU) (5 μM in rA:rU) solutions and mixture spectra at various TLdap/[rA:rU] ratios in PBS buffer (pH 7.5).
Abbreviations: CD, circular dichroism; PBS, phosphate-buffered saline.
![Figure 8 CD analysis at 5°C in a two-chamber cell (optical path = 0.875 cm): sum (black) spectrum of oligoDapT (4, 4 μM in TLdap) and the RNA duplex poly(rA):poly(rU) (5 μM in rA:rU) solutions and mixture spectra at various TLdap/[rA:rU] ratios in PBS buffer (pH 7.5).Abbreviations: CD, circular dichroism; PBS, phosphate-buffered saline.](/cms/asset/9e97dda1-1882-42f4-baba-e8f7e3c6ea24/dijn_a_12193970_f0008_c.jpg)
Taken together, the abovementioned findings demonstrated the ability of oligoDapT to bind all the nucleic acid systems selected for this study. Furthermore, CD titration experiments evidenced some differences in the binding stoichiometry of our nucleopeptide toward DNA vs RNA. In fact, the TLdap/A or TLdap/[A:T(U)] ratios were >4 in the case of oligoDapT/DNA and ~2 in the case of oligoDapT/RNA complexes.
Thermal stabilities of oligoDapT/nucleic acid complexes
Another aspect we have investigated is the thermal stability of the complexes formed by oligoDapT with DNA and RNA. To this aim, UV thermal denaturation experiments were performed (–), whose results are summarized in .
Table 1 Melting temperatures for homothymine/homoadenine natural (DNA/DNA or RNA/RNA) and artificial (nucleopeptide/DNA and nucleopeptide/RNA) complexes
In the case of DNA, our nucleopeptides 4 and 5, containing, respectively, 5 and 6 TLdap units, formed with the dA12 strand more stable complexes than that obtained with the natural and longer dT12 ( and ). Regarding RNA, we observed a Tm of 47.9°C for the poly(rU)/poly(rA) complex, whereas an almost 20°C more stable complex was formed, under the same experimental conditions, when poly(rA) bound oligoDapT ( and and ). This stabilization is greater also than that relative to other literature nucleotide/RNA complexes.Citation47 Moreover, oligoDapT forms more stable complexes with RNA than with DNA of the same nucleotide sequence, as observed comparing Tms of oligoDapT 4/dA12 and oligoDapT 4/rA12 (ΔTm ~10°C; ).
All the results obtained for the nucleopeptide/nucleic acid complexes can be explained considering the contribution of several factors to the recognition ability: 1) the H-bonding of complementary nucleobases; 2) the electrostatic interactions between the positive amino groups along the nucleopeptide chain and the negative phosphates of the nucleic acid strand; 3) the possibility for nucleopeptides to form multimeric structures also in complex with nucleic acids;Citation26 and 4) the additional H-bonding possibilities involving the 2′-OH groups of the ribose ring (in RNA).
Conclusion
Here, we reported the synthesis of a novel thymine-bearing nucleoamino acid based on l-Dap and its convenient oli-gomerization in the solid phase to α-peptides (oligoDapT), characterized by ESI-MS and SEM analyses. Nucleic acid-binding studies, based on CD and UV experiments carried out on oligoDapT nucleopeptides with both single- and double-stranded DNA and RNA, evidenced sequence-specific interactions in all cases. Remarkable changes in the structures of the dA12/dT12 and poly(rA)/poly(rU) complexes were observed by CD spectroscopy upon oligoDapT addition. CD titrations revealed that multiple oligoDapT units bound all the examined nucleic acids, with TLdap/A or TLdap/[A:T(U)] ratios twofold higher for oligoDapT/DNA than for oligoDapT/RNA complexes. Additionally, UV melting experiments showed higher thermal stabilities of oligoDapT/nucleic acid complexes with respect to the natural DNA/DNA and RNA/RNA complexes and a higher stability of oligoDapT/RNA vs oligoDapT/DNA complexes, probably associated with the additional H-bonding given by 2′-OH in ribose moieties of RNA.
In future, we plan to synthesize oligoDap oligomers of different lengths containing all four nucleobases in order to evaluate their binding properties toward complementary nucleic acids and their ability to form suitable nucleopeptide-based biomaterials.Citation48
Overall, the here-reported oligoDapT showed interesting properties exploitable in the context of molecular devices for potential biomedical applications; indeed, this is a nucleic acid binder able to target complementary DNA and RNA of interest in antigene, antisense, and double-stranded RNA targeting antiviral strategies, as well as to be used for the delivery of therapeutic ODNs, a recently evidenced property of nucleopeptides.Citation26
Acknowledgments
We thank Prof Antonio Roviello and Mr Leopoldo Zona for their precious suggestions.
Supplementary materials
This section provides
a structural representation of a hexathymine oligoDapT;
a structural representation of the interaction between oligoDapT and nucleic acids;
liquid chromatography-electrospray ionization-mass spectrometry (LC-ESI-MS) characterization of the thymine-based monomer 3 and nucleopeptides 4 and 5;
1H and 13C NMR spectra of the nucleoamino acid 3; and
ultraviolet (UV) melting curves relative to oligoDapT/DNA, oligoDapT/RNA, and controls (DNA/DNA and RNA/RNA).
Figure S2 H-bonding and ionic-based interactions occurring between oligoDapT and complementary nucleic acids.
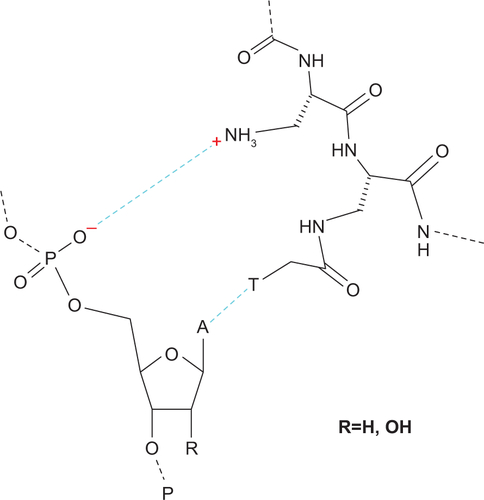
Figure S3 LC-ESI-MS (positive mode) analysis of the thymine-based monomer (3) used in this study (HPLC method on RP C18: 15%–70% of CH3CN [0.05% TFA] in H2O [0.05% TFA]). (A) ESI-MS spectrum of the chromatographic peak at 12 min; (B) extracted TIC chromatogram from 10.55 to 13.12 min; (C) extracted PDA chromatogram from 10.55 to 13.12 min in micro absorbance units.
Abbreviations: LC-ESI-MS, liquid chromatography-electrospray ionization-mass spectrometry; HPLC, high-performance liquid chromatography; RP, reversed phase; TFA, trifluoroacetic acid; ESI-MS, electrospray ionization-mass spectrometry; PDA, photodiode array; RT, retention time; TIC, total ion current.
![Figure S3 LC-ESI-MS (positive mode) analysis of the thymine-based monomer (3) used in this study (HPLC method on RP C18: 15%–70% of CH3CN [0.05% TFA] in H2O [0.05% TFA]). (A) ESI-MS spectrum of the chromatographic peak at 12 min; (B) extracted TIC chromatogram from 10.55 to 13.12 min; (C) extracted PDA chromatogram from 10.55 to 13.12 min in micro absorbance units.Abbreviations: LC-ESI-MS, liquid chromatography-electrospray ionization-mass spectrometry; HPLC, high-performance liquid chromatography; RP, reversed phase; TFA, trifluoroacetic acid; ESI-MS, electrospray ionization-mass spectrometry; PDA, photodiode array; RT, retention time; TIC, total ion current.](/cms/asset/615a925b-8e96-420a-b56a-e123b94b01cd/dijn_a_12193970_sf0003_c.jpg)
Figure S4 ESI-MS (positive mode) analyses of the thymine-based nucleopeptides 4 (A) and 5 (B).
Abbreviation: ESI-MS, electrospray ionization-mass spectrometry.
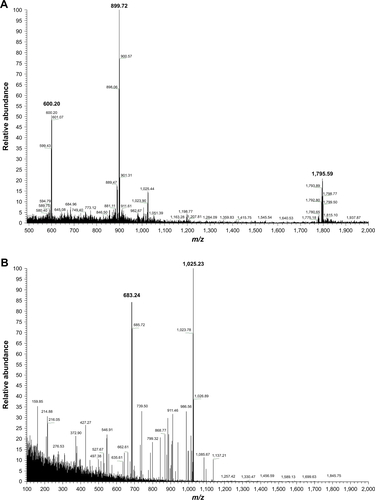
Figure S5 1H NMR spectrum of the nucleoamino acid 3 recorded at 25°C in deuterated DMF on a Varian 600 MHz unit.
Abbreviations: NMR, nuclear magnetic resonance; DMF, N,N-dimethylformamide.
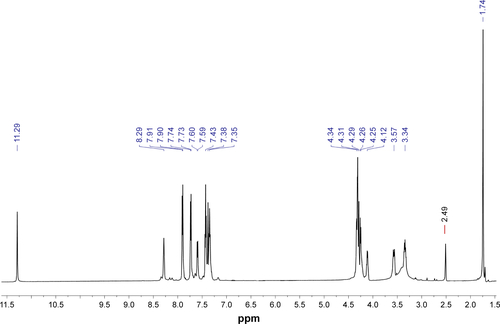
Figure S6 13C NMR spectrum of the nucleoamino acid 3 recorded at 25°C in deuterated DMF on a Varian 600 MHz (150 MHz) unit.
Abbreviations: NMR, nuclear magnetic resonance; DMF, N,N-dimethylformamide.
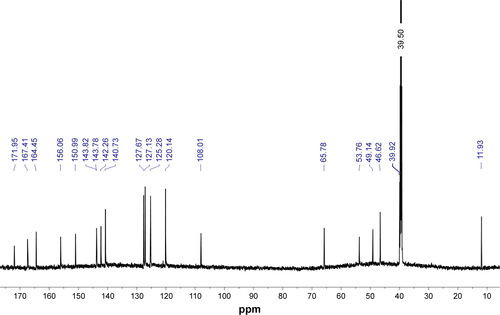
Figure S7 UV melting profiles for the complexes formed in 10 mM phosphate buffer (pH = 7.5) between dA12 (4 μM) and (A) 1 equiv. dT12, (B) 3.5 equiv. oligoDapT 4, and (C) 3.5 equiv. oligoDapT 5.
Abbreviation: UV, ultraviolet.
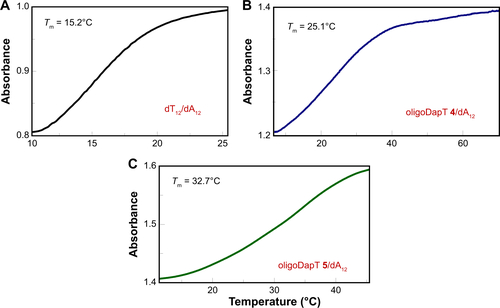
Figure S8 Normalized (0–1) UV melting profiles for the complexes formed in 10 mM phosphate buffer (pH = 7.5) between dA12 (4 μM) and 1 equiv. dT12 (black line), 3.5 equiv. oligoDapT 4 (blue), and 3.5 equiv. oligoDapT 5 (red).
Abbreviation: UV, ultraviolet.
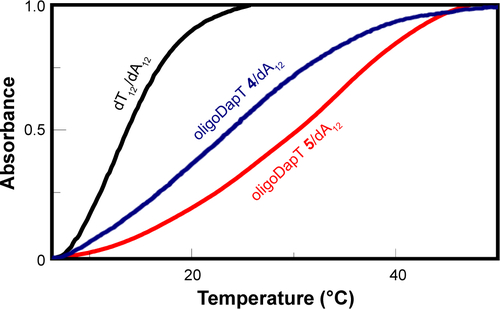
Figure S9 (A) UV melting profile for the complex formed between rA12 (4 μM) and 2.5 equiv. oligoDapT 4 in 10 mM phosphate buffer (pH = 7.5). (B) Normalized (0–1) UV melting profiles for the complexes oligoDapT/dA12 (blue) and oligoDapT/rA12 (red) formed in 10 mM phosphate buffer (pH = 7.5).
Abbreviation: UV, ultraviolet.
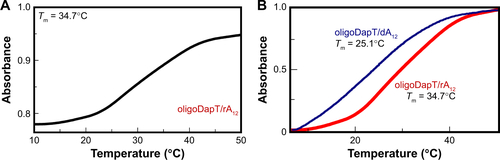
Disclosure
The authors report no conflicts of interest in this work.
References
- RovielloGNGaetanoSDCapassoDFrancoSCrescenzoCBucciEMPedone C RNA-binding and viral reverse transcriptase inhibitory activity of a novel cationic diamino acid-based peptideJ Med Chem2011542095210121391685
- WeckenmannNMNachtsheimBJEnantioselective synthesis of D-α-(Uracil-5-yl) glycine derivatives and their racemization-free incorporation into a model peptideEur J Org Chem20153066246630
- RovielloGNRicciABucciEMPedoneCSynthesis, biological evaluation and supramolecular assembly of novel analogues of peptidyl nucleosidesMol Biosyst2011751773177821431179
- ScognamiglioPLDi NataleCPerrettaGMarascoDFrom peptides to small molecules: an intriguing but intricated way to new drugsCurr Med Chem201320313803381723895692
- ShiNQGaoWXiangBQiXREnhancing cellular uptake of activable cell-penetrating peptide-doxorubicin conjugate by enzymatic cleavageInt J Nanomedicine201271613162122619516
- DolmanNPMoreJCAltAStructure-activity relationship studies on N3-substituted willardiine derivatives acting as AMPA or kainate receptor antagonistsJ Med Chem20064982579259216610801
- MizutaniMJitsukawaKMasudaHEinagaHSynthesis and X-ray structure of a unique multinuclear complex constructed by a nucleobase-amino acid DL-willardiineChem Comm1996111113891390
- RovielloGNMusumeciDSynthetic approaches to nucleopeptides containing all four nucleobases, and nucleic acid-binding studies on a mixed-sequence nucleo-oligolysineRSC Adv2016668635786358528496969
- RovielloGNRovielloGMusumeciDBucciEMPedoneCDakin-West reaction on 1-thyminyl acetic acid for the synthesis of 1,3-bis(1-thyminyl)-2-propanone, a heteroaromatic compound with nucleopeptide-binding propertiesAmino Acids20124341615162322349760
- RovielloGNRovielloVAutieroISavianoMSolid phase synthesis of TyrT, a thymine–tyrosine conjugate with poly(A) RNA-binding abilityRSC Adv20166276072761329057072
- RovielloGNMusumeciDPedoneCBucciEMSynthesis, characterization and hybridization studies of an alternate nucleo-epsilon/gammapeptide: complexes formation with natural nucleic acidsAmino Acids201038110311119067107
- RovielloGNMusumeciDMocciaMdabPNA: design, synthesis, and DNA binding studiesNucleos Nucleot Nucl Acids20072613071310
- RovielloGNMusumeciDBucciEMPedoneCSynthesis and characterization of a novel ester-based nucleoamino acid for the assembly of aromatic nucleopeptides for biomedical applicationsInt J Pharm20114151–220621021689737
- NielsenPEEgholmMBergRHBuchardtOSequence-selective recognition of DNA by strand displacement with a thymine-substituted polyamideScience19912545037149715001962210
- MilanoGMusumeciDGaglioneMMessereAAn alternative strategy to synthesize PNA and DNA magnetic conjugates forming nanoparticle assembly based on PNA/DNA duplexesMol Biosyst20106355356120174683
- RovielloGNMusumeciDDe CristofaroAAlternate dab-aegPNAs: synthesis, nucleic acid binding studies and biological activityMol Biosyst20096119920520024081
- AmatoFTomaiuoloRBorboneNDesign, synthesis and biochemical investigation, by in vitro luciferase reporter system, of peptide nucleic acids as new inhibitors of miR-509-3p involved in the regulation of cystic fibrosis disease-gene expressionMed Chem Commun201456871
- AmatoFTomaiuoloRNiciFExploitation of a very small peptide nucleic acid as a new inhibitor of miR-509-3p involved in the regulation of cystic fibrosis disease-gene expressionBiomed Res Int2014201461071824829907
- PintoBRuscianoGD’ErricoSSynthesis and label free characterization of a bimolecular PNA homo quadruplexBiochim Biophys Acta201718615 Pt B1222122827913190
- RovielloGNMusumeciDBucciEMEvidences for complex formation between L-dabPNA and aegPNABioorg Med Chem Lett200818174757476018707882
- RovielloGNDi GaetanoSCapassoDCesaraniABucciEMPedoneCSynthesis, spectroscopic studies and biological activity of a novel nucleopeptide with Moloney murine leukemia virus reverse transcriptase inhibitory activityAmino Acids20103851489149619813074
- RovielloGNVicidominiCGaetanoSDCapassoDMusumeciDRovielloVSolid phase synthesis and RNA-binding activity of an arginine-containing nucleopeptideRSC Adv2016617141401414829057071
- RovielloGNCrescenzoCCapassoDSynthesis of a novel Fmoc-protected nucleoaminoacid for the solid phase assembly of 4-piperidyl glycine/L-arginine-containing nucleopeptides and preliminary RNA: interaction studiesAmino Acids201039379580020204432
- MusumeciDRiccardiCMontesarchioDG-quadruplex forming oligonucleotides as anti-HIV agentsMolecules2015209175111753226402662
- WatanabeSTomizakiKYTakahashiTUsuiKKajikawaKMiharaHInteractions between peptides containing nucleobase amino acids and T7 phages displaying S. cerevisiae proteinsPept Sci2007882131140
- DuXZhouJLiXXuBSelf-assembly of nucleopeptides to interact with DNAsInterface Focus2017762016011629147550
- SahuBSacuiIRapireddySSynthesis and characterization of conformationally preorganized, (R)-diethylene glycol-containing γ-peptide nucleic acids with superior hybridization properties and water solubilityJ Org Chem2011765614562721619025
- Di NataleCScognamiglioPLCascellaRNucleophosmin contains amyloidogenic regions that are able to form toxic aggregates under physiological conditionsFASEB J20152993689370125977257
- AdinolfiMDe NapoliLDi FabioGIadonisiAMontesarchioDModulating the activity of oligonucleotides by carbohydrate conjugation: solid phase synthesis of sucrose-oligonucleotide hybridsOrg Biomol Chem20042131879188615227540
- MusumeciDRozzaLMerlinoAInteraction of anticancer Ru(III) complexes with single stranded and duplex DNA model systemsDalton Trans20154431139141392526154188
- RovielloGNVicidominiCCostanzoVRovielloVNucleic acid binding and other biomedical properties of artificial oligolysinesInt J Nanomedicine2016115897590428115843
- ZhangSHolmesTCDi PersioCMHynesROSuXRichASelf-complementary oligopeptide matrices support mammalian cell attachmentBiomaterials19951618138513938590765
- YuanDShiJDuXZhouNXuBSupramolecular glycosylation accelerates proteolytic degradation of peptide nanofibrilsJ Am Chem Soc201513732100921009526237170
- SalehTBolhassaniAShojaosadatiSAHosseinkhaniSEvaluation of cell penetrating peptide delivery system on HPV16E7 expression in three types of cell lineIran J Biotechnol2015131556228959282
- GourNKedrackiDSafirINgoKXVebert-NardinCSelf-assembling DNA-peptide hybrids: morphological consequences of oligonucleotide grafting to a pathogenic amyloid fibrils forming dipeptideChem Commun2012484454405442
- RovielloGNMusumeciDD’AlessandroCPedoneCBinding ability of a thymine-functionalized oligolysine towards nucleic acidsBioorg Med Chem2014223997100224411200
- SongGRenJRecognition and regulation of unique nucleic acid structures by small moleculesChem Commun2010463972837294
- RovielloGNMusumeciDRovielloVPirtskhalavaMEgoyanAMirtskhulavaMNatural and artificial binders of polyriboadenylic acid and their effect on RNA structureBeilstein J Nanotechnol201561338134726199837
- SahaBSuresh KumarGSpectroscopic and calorimetric investigations on the binding of phenazinium dyes safranine-O and phenosafranine to double stranded RNA polynucleotidesJ Photochem Photobiol B201616112914027236048
- KabirASuresh KumarGTargeting double-stranded RNA with sper-mine, 1-naphthylacetyl spermine and spermidine: a comparative biophysical investigationJ Phys Chem B201411838110501106425184857
- DasASuresh KumarGProbing the binding of two sugar bearing anticancer agents aristololactam-β-(D)-glucoside and daunomycin to double stranded RNA polynucleotides: a combined spectroscopic and calorimetric studyMol Biosyst201281958196922596256
- HaraRIMaedaYSakamotoTWadaTDouble-stranded RNA-binding artificial cationic oligosaccharides stabilizing siRNAs with a low N/P ratioOrg Biomol Chem20171571710171728138666
- ChenBYooKXuWPanRHanXXChenPCharacterization and evaluation of a peptide-based siRNA delivery system in vitroDrug Deliv Transl Res20177450751528349343
- LinYHHuangJHLiuYBellesXLeeHJOral delivery of dsRNA lipoplexes to German cockroach protects dsRNA from degradation and induces RNAi responsePest Manag Sci201773596096627470169
- SanittPApiratikulNNiyomthamNCholesterol-based cationic liposome increases dsRNA protection of yellow head virus infection in Penaeus vannameiJ Biotechnol20162289510227140871
- AttasartPBoonmaSSunintaboonPTanwilaiDPothikasikornJNoonpakdeeWTInhibition of Plasmodium falciparum proliferation in vitro by double-stranded RNA nanoparticle against malaria topoisomerase IIExp Parasitol2016164849026953249
- RovielloGNMusumeciDCastiglioneMBucciEMPedoneCBenedettiESolid phase synthesis and RNA-binding studies of a serum-resistant nucleo-epsilon-peptideJ Pept Sci200915315516018985708
- YuanDDuXShiJZhouNZhouJXuBMixing biomimetic het-erodimers of nucleopeptides to generate biocompatible and biostable supramolecular hydrogelsAngew Chem Int Ed Engl201554195705570825783774