Abstract
Introduction
Macrocrystalline oxides of alkaline earth metals (Mg and Ca) or light metals (Al and Ti) can respond to standard warfare agents such as sulfur mustard, soman, or agent VX. In this paper, we compared the decontamination ability of sodium hydroxide (NaOH) and sodium hypochlorite (NaClO) for nitrogen mustards (cyclophosphamide [CP] and ifosfamide [IFOS]) with a new procedure using a destructive sorbent based on nanocrystalline and nanodispersive titanium dioxide (TiO2) as a new efficient and cheap material for complete decontamination of surfaces.
Methods
Titanium (IV) dioxide nanoparticles were prepared by the homogeneous hydrolysis of titanium(IV) oxysulfate (TiOSO4) with urea. The as-prepared TiO2 nanoparticles were used for the fast and safe decontamination of cytostatics from the nitrogen mustard family (CP and IFOS) in water. The adsorption–degradation process of cytostatics in the presence of TiO2 was compared with decontamination agents (0.01 M solution of sodium hydroxide and 5% solution of sodium hypochlorite). The mechanism of the decontamination process and the degradation efficiency were determined by high-performance liquid chromatography with mass spectrometry.
Results
It was demonstrated that a 0.01 M solution of sodium hydroxide (NaOH) decomposes CP to 3-((amino(bis(2-chloroethyl)amino)phosphoryl)oxy)propanoic acid and sodium hypochlorite formed two reaction products, namely, IFOS and 4-hydroxy-cyclophosphamide. IFOS is cytotoxic, and 4-hydroxy-cyclophosphamide is a known metabolite of CP after its partial metabolism by CYP/CYP450. IFOS degrades in the pres¬ence of NaOH to toxic IFOS mustard. Titanium(IV) dioxide nanoparticles adsorbed on its surface CP after 5 minutes and on IFOS after 10 minutes. The adsorption–degradation process of CP in water and in the presence of TiO2 led to 4-hydroxy-cyclophosphamide and IFOS, respectively, which decayed to oxidation product 4-hydroxy-ifosfamide.
Conclusion
Nanodispersive TiO2 is an effective degradation agent for decontamination of surfaces from cytostatics in medical facilities.
Introduction
Macrocrystalline oxides of alkaline earth metals (Mg and Ca) or light metals (Al and Ti) can respond to standard warfare agents such as sulfur mustard, soman, or agent VX.Citation1 Reactions with the macrocrystalline oxides are slow and unusable for military decontamination practices. However, if the size of the individual oxide particles decreases on the order of 1–10 nm, it is possible to observe an increase in their specific reactivity. Observed acceleration heterogeneous reactions, which take place on the surface of the nanocrystals, have been explained in particular by increasing the proportion of highly reactive sites, such as the edges and corners of nanocrystals in which the amount of dislocations or defects increase.Citation2
The sulfur mustards family includes eight useful agents, including sulfur mustard bis(2-chloroethyl)sulfide (yperite) used as a chemical warfare agent, which is cytotoxic and acts as a nonspecific DNA alkylating agent. After World War I, there was research into its effect on tumor cells. Cytostatics are first derived from the sulfur mustard, and with a substitution of nitrogen instead of sulfur, we get the nitrogen mustards. In 1942, they were classified to begin human clinical trials of nitrogen mustards for the treatment of lymphoma.Citation3 There was an incident during an air raid on Bari (Bari) in World War II, where a release of mustard gas affected hundreds of soldiers and civilians.Citation4 On the basis of these past studies, bis(2-chlorethyl)ethylamine, called Mustin, was the first prototype of an anticancer chemotherapeutic.Citation4
The nitrogen mustards used as warfare agents include HN1 – bis(2-chlorethyl)ethylamine, HN2 – bis(2-chlorethyl) methylamine, and HN3 – tris(2-chlorethyl)amine, and derivatives from uracil mustard (5-[bis(2-chloroethyl) amino]-1H-pyrimidine-2,4-dione) that are used as cytotoxic chemotherapy agents include cyclophosphamide (CP) (N,N-bis(2-chlor)), chlorambucil(4-[bis(2-chlorethyl) amino]benzenebutanoic acid), ifosfamide (IFOS) (N,3-bis(2-chloroethyl)-1,3,2-oxazaphosphinan-2-amide 2-oxide), melphalan (4-[bis(2-chloroethyl)amino]-l-phenylalanine), and bendamustine (4-[5-[bis(2-chloroethyl)amino]-1-methylbenzimidazol-2-yl]butanoic acid).
CP is the most common and most versatile cytostatic. It is a unique alkylating cytostatic agent for the following reasons: CP alone is ineffective; the cytostatic effect is due to its metabolite formed in the liver – 4-hydroxy-cyclofosfamide. The treatment affects the microsomal enzyme system and CYP/CYP450. This metabolite is found in two tautomer forms, namely, 4-hydroxy-cyclophosphamide and aldophosphamide, which are mutually balanced. In the cells, aldophosphamide decays to acrolein and the cytotoxic agent phosphorus-amino mustard, which is a bifunctional alkylating agent. IFOS, as well as CP, is inactive in vitro and is most likely activated in vivo in the liver by microsomal enzymes to 4-hydroxy-ifosfamide, which is in equilibrium with its tautomer aldoifosfamide. Aldo-phosfamide spontaneously decomposes to acrolein and the alkylation metabolite isofosfamide mustard.
Stenglova Netikova et alCitation5 presented new data, which demonstrated the possibility of using titanium(IV) dioxide as a new, efficient, and cheap material for complete decontamination of anthracycline antibiotics. This pioneering study makes it attractive to scientists and a promising alternative way to perform cytostatics decontamination.
In this paper, we compared the previously used decontamination methodology of sodium hydroxide (NaOH) and sodium hypochlorite (NaClO) for CP and IFOS with the new procedure using a destructive sorbent base on nanocrystalline and nanodispersive titanium dioxide (TiO2).
Experimental
All of the chemicals used, including titanium oxo-sulfate (TiOSO4), urea (CO(NH2)2), NaOH, NaClO, and sulfuric acid (H2SO4), were obtained from Sigma-Aldrich (St Louis, MO, USA), and CP and IFOS were obtained as drugs from the pharmaceutical company Sandoz (Holzkirchen, Germany). All the reagents used were analytical grade and employed without further purification; CP and IFOS were in pharmacological purity.
Titania TIT15 sample preparation
Homogeneous hydrolysis of titanium oxo-sulfate with urea was used to obtain titanium(IV) dioxide. This method is based on thermal decomposition of urea at a temperature higher than 60°C. In a typical synthesis,Citation5,Citation6 15 g of TiOSO4 was dissolved in 100 mL of hot distilled water acidified with 98% H2SO4. The pellucid liquid was diluted into 4 L of distilled water and supplemented with 200 g of urea. The mixture was heated to 98°C with stirring for 6 hours until it reached a pH of 7.2. The formed precipitate was washed using decantation until the conductivity of 10 S was reached, filtered off, and dried at 105°C.
Characterization methods
Preparation of stock solutions
For the stability testing, we prepared stock solutions of drugs in a variety of physiological solutions. The appropriate amount of drug injection solution in physiological solution was transferred to a 50 mL volumetric flask and filled to volume with physiological saline solution 0.9% sodium chloride (NaCl) at a final concentration of 20 µg/mL.
Chromatographic conditions
The concentrations of CP and IFOS were measured after adding NaOH (0.1 M) and NaClO (5%) by an isocratic high-performance liquid chromatography (HPLC) system of DIONEX UltiMate 3000 (Thermo Fisher Scientific, Waltham, MA, USA) consisting of a pump with a column of YMC Co. (Allentown, PA, USA; ODS Hydrosphere C18 150×4.6 mm ID) and a DAD detector with data-processing Chromeleon station software (Thermo Fisher Scientific). The UV detection of 5-FU was set at 230 nm. Mobile phase A consisted of 0.1% formic acid in 100% methanol, and mobile phase B consisted of 0.1% formic acid in Milli-Q water (5:95 v/v) at a flow rate of 0.500 mL/min. The column temperature was maintained at 30°C. The injected volume of each sample was 20 µL.
All samples after their degradation were analyzed by mass spectrometry (MS). The analysis of drugs was performed using an LTQ Orbitrap mass spectrometer (Thermo Fisher Scientific) following chromatographic separation using an HPLC system consisting of an autosampler and dual pumps. An HPLC system Thermo Finnigan (Thermo Fisher Scientific) was used. An LTQ Orbitrap MS equipped with an atmospheric pressure interface and an ESI ion source was used. The source voltage was set to 3.5 kV. The heated capillary temperature was maintained at 275°C. Analyses were run using full MS (50–1,000 m/z range) in the negative ion mode, with a resolution of 30,000 in ITMS mode.
The method was developed and validated with respect to linearity, accuracy, recovery, precision, system suitability, selectivity, and robustness, and forced degradation studies proved the stability indicating ability of the method.
Selectivity might include degradants and matrix. Decontamination studies were performed for cytotoxic drugs to provide an indication of the stability indicating property and selectivity of the proposed method. Intentional decomposition was attempted to stress conditions that were exposed to it with alkali (0.01 M NaOH) and sodium hypochlorite (5% NaClO) to evaluate the ability of the proposed method for a decontamination strategy of cytotoxic drugs.
Sampling procedure and analysis of the transformation products
Decontamination tests were performed by using CP as internal standards. In this procedure, standards were mixed into the decontamination solution in a series of glass vials (Supelco [Bellefonte, PA, USA], 20 mL). The vials were sealed with caps and covered with aluminum foil to protect the reaction mixture from sunlight. At predetermined time intervals (0, 5, 15, 20, 30, 50, 70, 90, and 120 minutes), the reaction was terminated by addition of formic acid (0.1%). The solution was transferred to a smaller vial (Supelco, 2.0 mL) and analyzed immediately by HPLC to determine oxidation or hydrolysis of the transformation products.
HPLC method for the decomposition of cytostatics
The decomposition of CP and IFOS on the reactive sorbents in water was measured at the University JE Purkyně in Ústí nad Labem using the methodology developed at the Institute of Inorganic Chemistry AVCR.Citation5
Fourier transform infrared spectroscopy analysis of cytostatics degradation
The CP and IFOS degradation processes were studied on a Nicolet Impact 400D FTIR spectrometer (Nicolet CZ, s.r.o., Prague, Czech Republic) equipped with the Praying Mantis™ (Harrick, Pleasantville, NY, USA) for diffuse reflection measurements (DRIFTS).Citation7
Results and discussion
As has already been discussed, nanocrystalline TiO2 prepared by homogeneous hydrolysis of oxo-titanium sulfate with urea was used for the degradation of CP and IFOS. According to the reaction conditions, TiO2 can be prepared either as an excellent photocatalytic pigmentCitation8–Citation10 or as a destructive sorbent for stoichiometric decomposition of chemical warfare agents,Citation6,Citation11 pesticides,Citation12 and some of the anthracycline antibiotic cytostatics.Citation5
CP is the most commonly used alkylating agent (adding an alkyl group [CnH2n+1] to the DNA) in tumor therapy and represents a great contribution to stopping the proliferation of tumor cells, but there is also a health risk for medical staff. Cyclophosphamide is a “prodrug” in which the active chemotherapeutic is created in the liver.
LC–MS chromatogram and mass spectra of CP are presented in ; the chromatographic peak at retention time tR =3.40 minutes with [M+H]+ =261.2 (ESI+) corresponds to pure CP.Citation13 The two reaction products are formed by the reaction of CP with NaClO; after 5 minutes of reaction, it produced 4-hydroxy-cyclophosphamide (tR =8.4 minutes, [M+H]+ =277.02), and after 15 minutes, it was identified as 3-(2-chloroethyl)–2-((2-chloroethyl)amino)–1,3,2-oxaza-phosphinane 2-oxide, (tR =6.50 minutes, [M+H]+ =261.09), also known under the name IFOS. LC chromatograms and the mass spectra of these reaction products are shown in . The chromatographic behavior demonstrated that the 4-hydroxy-CP formed during the reaction with NaClO has a higher polarity than IFOS, which is indicated by a longer retention time. The reaction pathway of CP conversion in the presence of sodium hypochlorite is shown in .
Figure 1 The pathway of conversion of CP in the presence of sodium hypochlorite (5% solution).
Abbreviations: CP, cyclophosphamide; CP-TP1-OCl, 4-hydroxy-cyclophosphamide; CP-TP2-OCl, ifosfamide.
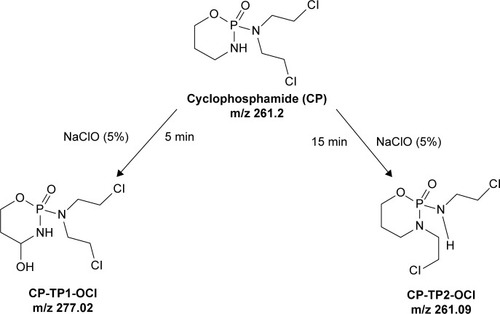
IFOS is similar to CP and is hydroxylated in cells to its active metabolite 4-hydroxy-ifosfamide and its tau-tomers form aldo-ifosfamide; by cleavage of acrolein, it changes from aldo-iphosphamide to iso-phosphorus-amino mustard.
The main active human metabolite of CP is 4-hydroxy-cyclophosphamide, and its formation occurs through hydroxylation.Citation14 IFOS is a chemotherapeutic agent chemically related to the nitrogen mustards and a synthetic analog of CP. TrisselCitation15 reported that IFOS is chemically stable in NaCl (0.9%). We expect that it will also be stable in sodium hypochlorite NaClO (5%), because no other reaction product has been detected.
The appearance of new peaks during the decontamination process of CP in 5% NaClO aqueous solution is indicative of the formation of decay products.
CP reacts with aqueous solutions of 0.01 M NaOH, and after 15 minutes, the reaction produces 3-((amino(bis(2-chloroethyl)amino)phosphoryl)oxy) propanoic acid (tR =7.49 minutes, [M+H]+ =293.02), which is also the end product. It is formed by the opening cycle of the CP backbone leading to the formation of carboxyl (–COOH) and amino (–NH2) groups. The scheme of reaction of 0.01 M NaOH with CP is presented in . HPLC–MS chromatogram and the mass spectra of transformation product 3-((amino(bis (2-chloroethyl)amino)phosphoryl)oxy) propanoic acid of CP are presented in .
Figure 2 Scheme of degradation CP after reaction with a solution of 0.01 M NaOH.
Abbreviations: CP, cyclophosphamide; CP-TP1-OH, 3-((amino(bis(2-chloroethyl) amino)phosphoryl)oxy) propanoic acid.
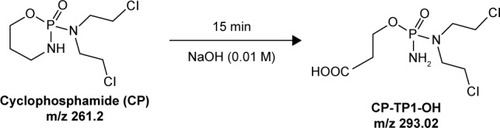
The results of the adsorption/degradation experiments in the presence of TiO2 indicated that no adsorption product occurred throughout the 60-minute reaction time in an aqueous medium. In the case of adsorption/degradation of CP on the surface of TiO2, we can explain that based on the knowledge of the stoichiometric degradation of organophosphates, which are decomposed bimolecular nucleophilic substitution SNCitation2 mechanisms, where surface –OH groups, in this case, act as a strong nucleophile, as we have reported in our previous studies.Citation16,Citation17 The electrophilic center nitrogen atom in the drug molecule will probably play a crucial role in the adsorption process. In the first part, the electron from the center nitrogen atom travels to the inside of the molecule. The mechanism is known as “inner sphere electron transfer”Citation18,Citation19 with elimination of a molecule of HCl. The mechanism is next followed by a substitution reaction to “temporarily” affect the electrophilic nitrogen atom (denoted as +). The final step is the creation of a stable surface complex. This can be explained by an increased number of catalyst active sites that are available for the adsorption reaction.Citation20,Citation21 The proposed mechanism of adsorption of CP on a titania surface is shown in . Kinetics of the reaction of CP with 5% solution NaClO and 0.01 M NaOH are presented in (blue curve). The origin and degradation of transformation products denoted as CP-TP1 (4-hydroxy-cyclophosphamide) shows a red curve and transformation products denoted as CP-TP2 (IFOS) in a green curve. It is clear that CP is not completely degraded after 120 minutes, and after reaction with sodium hydroxide, the reaction product is another cytotoxic agent, IFOS. CP in the presence of nanostructured TiO2 is adsorbed and gradually degraded without the formation of reaction products. The kinetics of CP degradation were calculated as first-order kinetics [odkaz], and are k=0.0602 min−1 and the degree of conversion is 89.7% at 120 minutes ().
Figure 3 The proposed mechanism of adsorption of CP on a titania surface.
Abbreviation: CP, cyclophosphamide.
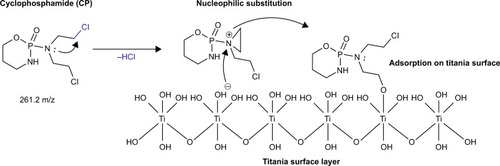
Figure 4 Transformation products formed during CP degradation plotted as a function of the normalized concentration (C/C0) of cyclophosphamide decay.
Note: Transformation products formed during the decontamination time (minutes) in the presence of (A) 5% NaClO agent, (B) 0.01 M NaOH agent, and (C) TiO2.
Abbreviations: CP, cyclophosphamide; CP-TP1-OCl, 4-hydroxy-cyclophosphamide; CP-TP2-OCl, ifosfamide.
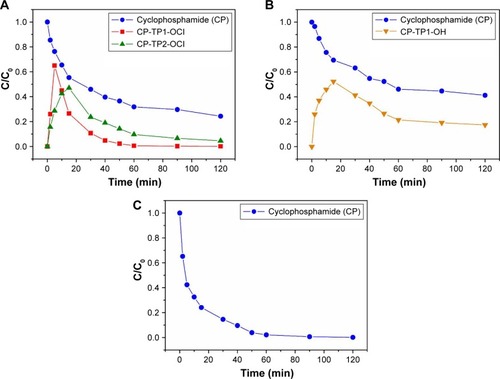
Since IFOS (m/z 261.09) was identified as one of the transformation products (CP-TP2-ClO) of CP in the NaClO (5%) agent, it was also in this case tested for its stability in both decontamination agents (NaClO and NaOH). The typi cal LC–MS chromatogram and mass spectra of IFOS (tR =6.50 minutes, [M+H]+ =261.09) are presented in . After the reaction with sodium hydroxide (NaOH, 0.01 M) the agent has had identified only one transformation product formed by alkylation of IFOS after 30 minutes as ifosforamide mustard (known as palifosfamide).Citation22 This transformation product is labeled IFOS-TP1-OH (tR =2.49 minutes, [M+H]+ =219.99). The LC–MS chromatogram and mass spectra of the transformation product IFOS-TP1-OH of IFOS in sodium hydroxide (NaOH 0.01 M agent) are shown in .
Kaijser et alCitation23 described a sensitive and selective method for the analysis of ifosforamide mustard in plasma. The method consists of direct derivatization of ifosforamide mustard in plasma with diethyldithiocarbamate and subsequent solid-phase extraction of the resulting derivative. The analysis of the derivatization product was performed by HPLC with UV detection (HPLC-UV).Citation23 Ifosforamide mustard probably represents the most important cytotoxic compound able to produce irreversible cross-links between DNA strands. In tumor cells, ifosforamide mustard reacts with DNA, causing cell death.Citation24 The scheme of IFOS degradation in the presence of 0.01 M NaOH is shown in . The sodium hypochlorite agent (5%) did not result in any identified transformation product.
Figure 5 The proposed pathway of conversion of IFOS in the presence of a sodium hydroxide (0.01 M) agent.
Abbreviations: IFOS, ifosfamide; IFOS-TP1-OH, ifosforamide mustard.
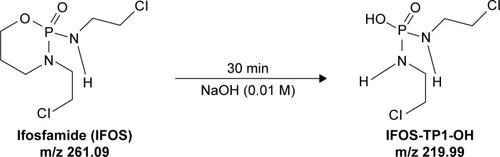
In the case of IFOS degradation on the surface of TiO2, only one oxidation product, 4-hydroxyifosfamide (C7H15Cl2N2O3P), was identified after 15 minutes of reaction time ([M+H]+ =276.09). presents the proposed pathway of adsorption of IFOS on a titania surface.
Figure 6 The proposed pathway of adsorption of IFOS on a titania surface.
Abbreviation: IFOS, ifosfamide.
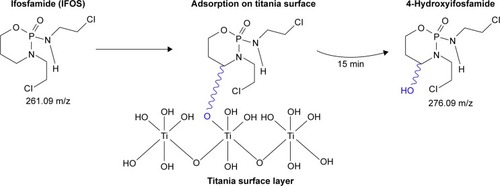
This degradation product is a metabolic product of IFOS, which is formed by hydroxylation at the ring carbon atom 4 to form the unstable intermediate 4-hydroxyifosfamide. This metabolite then rapidly degrades to the stable urinary metabolite 4-ketoifosfamide. The stable urinary metabolite, 4-carboxyifosfamide, is formed upon opening of the ring. These metabolites have not been found in obtained solutions after the adsorption reaction with TiO2.Citation25 The kinetics of the reaction of IFOS (violet curve) with the solution of 5% NaClO and 0.01 M NaOH are presented in . The green curve in shows the origin of the transformation products named IFOS-TP1-OH and ifosforamide mustard. Titanium dioxide mineralized IFOS and transformed 4-hydroxyifosmamide into phosphoric acid, carbon dioxide, and water. The rate constant k and degree of conversion d are 0.0746 min−1 and 96.5%, respectively ().
Figure 7 Transformation products formed during IFOS degradation plotted as a function of the normalized concentration (C/C0) of IFOS decay.
Note: Transformation products formed during the decontamination time (minutes) in the presence of (A) 5% NaClO agent, (B) 0.01 M NaOH agent, and (C) TiO2.
Abbreviations: IFOS, ifosfamide; IFOS-TP1-OH, ifosforamide mustard.
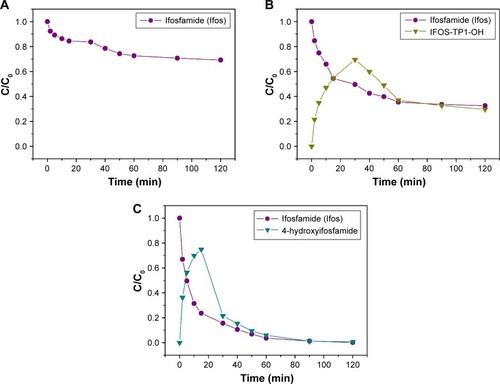
Significant degradation pathways of both agents, CP and IFOS, derived from mustard gasCitation26 as well as their degradation products were detected on the surface by in situ sensing DRIFT spectra in reaction time.Citation27 DRIFT spectral data provide evidence of the chemical interactions with a titania surface. The results of the degradation experiments in the heterogeneous CP–water–TiO2 system indicated that no degradation product occurred throughout the 120 minutes reaction time in an aqueous medium.
However, from the DRIFT spectra obtained in the reaction time for the CP–TiO2 system there are some important bands recognizable from surface-adsorbed CP. The surface degradation of CP is realized through binding of the electrophilic phosphoryl oxygen ν(P=O) to the surface hydroxyl groups by hydrogen-bond formation. The P=O bond (~1,280– 1,305 cm−1) is shifted to a lower wave number (~1,215 cm−1), which may indicate the decay of this group during stable surface complex formation with surface hydroxyls.Citation7,Citation27 As can be further seen, the vibrational band around a wave number of 1,170 cm−1 corresponding to ν(P–O) vibration absorption is lost over time. It has been demonstrated that the ring in the molecules was completely opened by cleavage of the P–O bond. On the other hand, vibration bands at 1,030–1,058 cm−1, which can be assigned to a specific vibration ν(P–O–C), remain unchanged, only showing a slight deformation due to the separation of the electronegative oxygen from the phosphorus in the ring.Citation28 Therefore, it is apparent that other vibration bands will be influenced. The peaks at 922 and 1,455–1,460 cm−1 are the absorptions of ν(P–N), and the peaks at 968, 2,850, and 2,960 cm−1 are the absorptions of ν(C–H) stretching vibrations.Citation29,Citation30 In the DRIFT spectra of CP and IFOS are the characteristic peaks at 785 and 884 cm−1, respectively, corresponding to the ν(C–Cl) bond, which slowly decreases due to the dehydrohalogenation pathway, where the Ti(IV) centers act as Lewis acids and promote the cleavage of the labile C–Cl bond in the drug molecule (the mechanism is known as “inner sphere electron transfer”) accompanied by elimination of a molecule of HCl.Citation31
In the case of IFOS degradation, we assume two different reaction pathways in aqueous solution (heterogeneous IFOS–water–TiO2 system) and the surface interaction of pure IFOS (IFOS–TiO2 system). In the first step, IFOS is superficially oxidized to 4-hydroxyifosfamide (4-H-IFOS) as the major degradation product identified after 5 minutes in the retention time of 5.213 minutes with m/z [M+H]+ =276.09 (faster process) followed by the opening of the agent cycle by cleavage of the P–O bond similarly to what occurs with CP (a slower process). This metabolite has not yet been found in the obtained solution after the adsorption reaction with TiO2.Citation32 Other DRIFT band characteristics remain similar to CP. Nevertheless, in all cases, the belt vibration intensity of the bands is reduced due to further cleavage of the molecules into smaller fragments, which apparently remain on the surface. In the presence of gaseous CO2(g), absorption bands appear at 1,706, 1,775, and 1,900 cm−1, corresponding to the carbonyl group ν(C=O). The band at 2,361 cm−1 is assigned to adsorbed CO2 with a Ti-O-C-O adsorption configuration.Citation33
The examples of the fingerprint DRIFT spectra (700–4,000 cm−1) obtained at preselected time intervals (0, 15, 30, 50, 90, and 120 minutes) after reactive adsorption on the titanium oxide denoted TIT15 are presented in and . The proposed mechanism pathways of adsorption of CP and IFOS on the titania surface are shown in and .
Figure 8 The DRIFTS fingerprint spectra of CP destructive adsorption on the titanium oxide.
Abbreviations: CP, cyclophosphamide; DRIFTS, diffuse reflectance infrared Fourier transform spectroscopy.
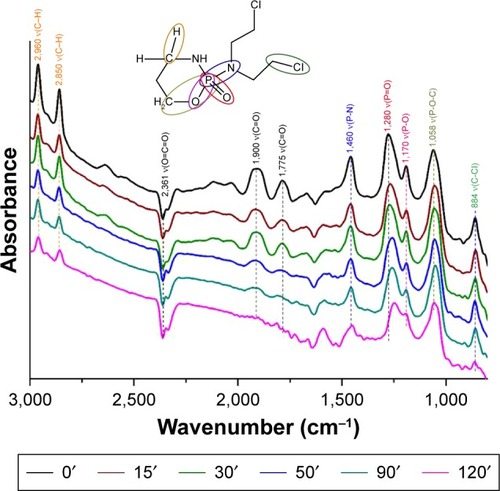
Figure 9 The DRIFTS fingerprint spectra of IFOS destructive adsorption on the titanium oxide.
Abbreviations: IFOS, ifosfamide; DRIFTS, diffuse reflectance infrared Fourier transform spectroscopy.
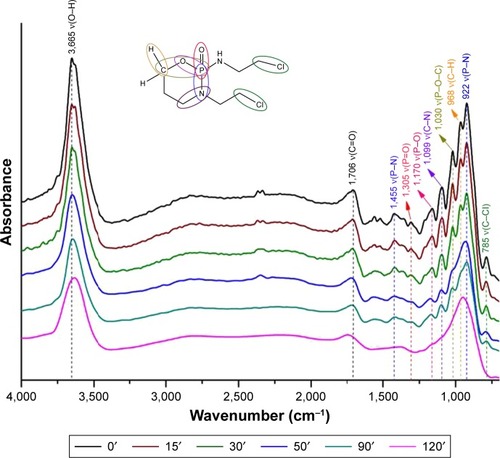
Conclusion
Aqueous solutions of sodium hydroxide and sodium hypochlorite have been used as cleaner simulants in medical facilities for the remediation of cytostatics from the family of nitrogen mustards. Sodium hydroxide decomposes CP to 3-((amino(bis(2-chloroethyl)amino)phosphoryl)oxy) propanoic acid and sodium hypochlorite forms two reaction products, namely, IFOS and 4-hydroxy-cyclophosphamide. IFOS is stable in a solution of sodium hypochlorite; IFOS in the presence of sodium hydroxide is converted to toxic IFOS mustard. In contrast, a destructive sorbent based on nanocrystalline and nanodispersive TiO2 can on its surface stoichiometrically decompose CP and IFOS respectively, into a nontoxic reaction product (fragments) and then mineralize them to water and carbon dioxide. Nanodispersive TiO2 is an effective degradation agent for decontamination of surfaces from cytostatics in medical facilities.
Supplementary materials
Figure S1 HPLC–MS chromatogram (A) and mass spectra (B) of CP.
Note: Adduction is represented by [M+H]+, formed by the interaction of a molecule with a proton (hydron).
Abbreviations: CP, cyclophosphamide; HPLC–MS, high-performance liquid chromatography–mass spectrometry; NL, intensity of the signal; RT, retention time.
![Figure S1 HPLC–MS chromatogram (A) and mass spectra (B) of CP.Note: Adduction is represented by [M+H]+, formed by the interaction of a molecule with a proton (hydron).Abbreviations: CP, cyclophosphamide; HPLC–MS, high-performance liquid chromatography–mass spectrometry; NL, intensity of the signal; RT, retention time.](/cms/asset/a25029ae-1422-4f83-a77a-6782fe0d9e08/dijn_a_12194008_sf0001_b.jpg)
Figure S2 HPLC–MS chromatogram (A) and mass spectra of the transformation product of CP (B) 4-hydroxycyclophosphamide and (C) 3-(2-chloroethyl)-2-((2-chloroethyl) amino)-1,3,2-oxazaphosphinane 2-oxide (IFOS) from CP in sodium hypochlorite 5% solution (NaClO).
Note: Adduction is represented by [M+H]+, formed by the interaction of a molecule with a proton (hydron).
Abbreviations: CP, cyclophosphamide; HPLC–MS, high-performance liquid chromatography–mass spectrometry; NL, intensity of the signal; RT, retention time.
![Figure S2 HPLC–MS chromatogram (A) and mass spectra of the transformation product of CP (B) 4-hydroxycyclophosphamide and (C) 3-(2-chloroethyl)-2-((2-chloroethyl) amino)-1,3,2-oxazaphosphinane 2-oxide (IFOS) from CP in sodium hypochlorite 5% solution (NaClO).Note: Adduction is represented by [M+H]+, formed by the interaction of a molecule with a proton (hydron).Abbreviations: CP, cyclophosphamide; HPLC–MS, high-performance liquid chromatography–mass spectrometry; NL, intensity of the signal; RT, retention time.](/cms/asset/3235e46c-26ac-4e6f-80be-322ff9afa262/dijn_a_12194008_sf0002_b.jpg)
Figure S3 HPLC–MS chromatogram (A) and mass spectra (B) of transformation product 3-((amino(bis(2-chloroethyl)amino)phosphoryl)oxy) propanoic acid from CP in sodium hydroxide 0.01 M (NaOH) solution.
Note: Adduction is represented by [M+H]+, formed by the interaction of a molecule with a proton (hydron).
Abbreviations: CP, cyclophosphamide; HPLC–MS, high-performance liquid chromatography–mass spectrometry; NL, intensity of the signal; RT, retention time.
![Figure S3 HPLC–MS chromatogram (A) and mass spectra (B) of transformation product 3-((amino(bis(2-chloroethyl)amino)phosphoryl)oxy) propanoic acid from CP in sodium hydroxide 0.01 M (NaOH) solution.Note: Adduction is represented by [M+H]+, formed by the interaction of a molecule with a proton (hydron).Abbreviations: CP, cyclophosphamide; HPLC–MS, high-performance liquid chromatography–mass spectrometry; NL, intensity of the signal; RT, retention time.](/cms/asset/c4497bbe-fc61-4ee3-b5f4-9355f3c2f2b2/dijn_a_12194008_sf0003_b.jpg)
Figure S4 Typical LC–MS chromatogram (A) and mass spectra (B) of IFOS.
Note: Adduction is represented by [M+H]+, formed by the interaction of a molecule with a proton (hydron).
Abbreviations: IFOS, ifosfamide; LC–MS, liquid chromatography–mass spectrometry; NL, intensity of the signal; RT, retention time.
![Figure S4 Typical LC–MS chromatogram (A) and mass spectra (B) of IFOS.Note: Adduction is represented by [M+H]+, formed by the interaction of a molecule with a proton (hydron).Abbreviations: IFOS, ifosfamide; LC–MS, liquid chromatography–mass spectrometry; NL, intensity of the signal; RT, retention time.](/cms/asset/35551bda-f24d-469f-a975-1dc7c678a4f7/dijn_a_12194008_sf0004_b.jpg)
Figure S5 LC–MS chromatogram (A) and mass spectra (B) of the transformation product IFOS-TP1-OH of IFOS in sodium hydroxide 0.01 M (NaOH) solution.
Note: Adduction is represented by [M+H]+, formed by the interaction of a molecule with a proton (hydron).
Abbreviations: IFOS, ifosfamide; IFOS-TPI-OH, 3-((amino(bis(2-chloroethyl)amino)phosphoryl)oxy) propanoic acid; LC–MS, liquid chromatography–mass spectrometry; NL, intensity of the signal; RT, retention time.
![Figure S5 LC–MS chromatogram (A) and mass spectra (B) of the transformation product IFOS-TP1-OH of IFOS in sodium hydroxide 0.01 M (NaOH) solution.Note: Adduction is represented by [M+H]+, formed by the interaction of a molecule with a proton (hydron).Abbreviations: IFOS, ifosfamide; IFOS-TPI-OH, 3-((amino(bis(2-chloroethyl)amino)phosphoryl)oxy) propanoic acid; LC–MS, liquid chromatography–mass spectrometry; NL, intensity of the signal; RT, retention time.](/cms/asset/9e0b2718-34d8-48f2-a11c-f8b05a6b666a/dijn_a_12194008_sf0005_b.jpg)
Disclosure
The authors report no conflicts of interest in this work.
References
- WagnerGWBartramPWVxKKJGD and HD reactions with nanosize particlesAbstracts of Papers of the American Chemical Society199921732253228
- StenglVHenychJJanosPSkoumalMNanostructured metal oxides for stoichiometric degradation of chemical warfare agentsDeVoogtPReviews of Environmental Contamination and Toxicology236BerlinSpringer2016239258
- GilmanAThe initial clinical trial of nitrogen mustardAm J Surg1963105557457813947966
- HirschJAn anniversary for cancer chemotherapyJAMA2006296121518152017003400
- Stenglova NetikovaIRSlusnaMTolaszJSt’astnyMPopelkaSStenglVA new possible way of anthracycline cytostatics decontaminationNew J Chem20174139753985
- ŠtenglVMaříkováMBakardjievaSŠubrtJOpluštilFOlšanskáMReaction of sulfur mustard gas, soman and agent VX with nanosized anatase TiO2 and ferrihydriteJ Chem Technol Biotechnol2005807754758
- ŠtenglVHenychJGrygarTPérezRChemical degradation of trimethyl phosphate as surrogate for organo-phosporus pesticides on nanostructured metal oxidesMater Res Bull201561259269
- BakardjievaSŠubrtJŠtenglVDianezMJSayaguesMJPhoto-activity of anatase–rutile TiO2 nanocrystalline mixtures obtained by heat treatment of homogeneously precipitated anataseAppl Catal B2005583–4193202
- ŠtenglVBakardjievaSMurafaNHouškováVLangKVisible-light photocatalytic activity of TiO2/ZnS nanocomposites prepared by homogeneous hydrolysisMicroporous and Mesoporous Materials20081102–3370378
- ŠtenglVBakardjievaSMurafaNPreparation and photocatalytic activity of rare earth doped TiO2 nanoparticlesMater Chem Phys20091141217226
- StenglVGrygarTMOpluštilFNěmecTGe4+ doped TiO2 for stoichiometric degradation of warfare agentsJ Hazard Mater20122272286267
- HenychJŠtenglVSlušnáMDegradation of organophosphorus pesticide parathion methyl on nanostructured titania-iron mixed oxidesAppl Surf Sci2015344916
- Santana-VieraSGuedes-AlonsoRSosa-FerreraZSantana-RodríguezJJKabirAFurtonKGOptimization and application of fabric phase sorptive extraction coupled to ultra-high performance liquid chromatography tandem mass spectrometry for the determination of cytostatic drug residues in environmental watersJ Chromatogr A20171529394929132820
- BaumannFPreissRCyclophosphamide and related anticancer drugsJ Chromatogr B Biomed Sci Appl20017641–217319211817027
- TrisselLAThe Handbook on Injectable Drugs: Single User VersionBethesda, MDAmerican Society of Health-System Pharmacists2011
- JanosPKuranPKormundaMCerium dioxide as a new reactive sorbent for fast degradation of parathion methyl and some other organophosphatesJ Rare Earths2014324360370
- ŠťastnýMŠtenglVHenychJTolaszJVomáčkaPEdererJMesoporous manganese oxide for the degradation of organophosphates pesticidesJ Mater Sci201651526342642
- MatyjaszewskiKInner sphere and outer sphere electron transfer reactions in atom transfer radical polymerizationMacromol Symp19981341105118
- JeonJGoddardWAKimHInner-sphere electron-transfer single iodide mechanism for dye regeneration in dye-sensitized solar cellsJ Am Chem Soc201313572431243423384053
- MaierWFTransition Metal Oxides: Surface Chemistry and Catalysis. (Reihe: Studies in Surface Science and Catalysis, Vol. 45). Von H. H. Kung. Elsevier, Amsterdam 1989. X, 282 S., geb. HFl. 215.00. – ISBN 0-444-87394-5Angew Chem Int Ed Engl19901028965966
- GqLLinsebiglerAYatesJTTi3+ Defect Sites on TiO2 (110) – Production and Chemical Detection of Aactive SitesJ Phys Chem199498451173311738
- NicolaoPGiomettoBNeurological toxicity of ifosfamideOncology200365Suppl 2111614586141
- KaijserGPBeijnenJHRozendomEBultAUnderbergWJAnalysis of ifosforamide mustard, the active metabolite of ifosfamide, in plasmaJ Chromatogr B Biomed Appl199668622492558971607
- FurlanutMFranceschiLPharmacology of ifosfamideOncology200365Suppl 22614586139
- FlemingRAAn overview of cyclophosphamide and ifosfamide pharmacologyPharmacotherapy1997175S146S154
- GoodmanLSWintrobeMMDameshekWGoodmanMJGilmanAMclennanMTNitrogen-mustard therapy – use of methyl-bis(beta-chloroethyl)amine hydrochloride and tris(beta-chloroethyl)amine hydrochloride for hodgkins-disease, lymphosarcoma, leukemia and certain allied and miscellaneous disorders (Reprinted)JAMA198425117225522616368885
- HenychJJanošPKormundaMTolaszJStenglVReactive adsorption of toxic organophosphates parathion methyl and DMMP on nanostruc tured Ti/Ce oxides and their compositesArabian Journal of Chemistry Epub1162016
- PaciAMartensTRoyerJAnodic oxidation of ifosfamide and cyclophosphamide: a biomimetic metabolism model of the oxaza-phosphorinane anticancer drugsBioorg Med Chem Lett200111101347134911392552
- WenZJinchengWPreparation and Characterization of a Cyclo-phosphamide-Core PAMAM Dendritic MontmorilloniteSilicon2018102483493
- JamesCRavikumarCSundiusTFT-Raman and FTIR spectra, normal coordinate analysis and ab initio computations of (2-methylphenoxy)acetic acid dimerVib Spectrosc20084711020
- Arcibar-OrozcoJAPanettieriSBandoszTJReactive adsorption of CEES on iron oxyhydroxide/(N-)graphite oxide composites under visible light exposureJ Mater Chem A Mater20153331708017090
- FlemingRAAn overview of cyclophosphamide and ifosfamide pharmacologyPharmacotherapy1997175 Pt 2146s154s9322882
- LiaoL-FLienC-FShiehD-LChenM-TLinJ-LFTIR Study of Adsorption and Photoassisted Oxygen Isotopic Exchange of Carbon Monoxide, Carbon Dioxide, Carbonate, and Formate on TiO2J Phys Chem B2002106431124011245