Abstract
Being a non-invasive and relatively safe technique, photothermal therapy has attracted a lot of interest in the cancer treatment field. Recently, nanostructure technology has entered the forefront of cancer therapy owing to its ability to absorb near-infrared radiation as well as efficient light to heat conversion. In this study, key nanostructures for cancer therapy including gold nanoparticles, magnetite iron oxide nanoparticles, organic nanomaterials, and novel two-dimensional nanoagents such as MXenes are discussed. Furthermore, we briefly discuss the characteristics of the nanostructures of these photothermal nanomaterial agents, while focusing on how nanostructures hold potential as cancer therapies. Finally, this review offers promising insight into new cancer therapy approaches, particularly in vivo and in vitro cancer treatments.
Introduction
Globally, cancer as an invasive and malignant disease is considered the second leading cause of mortality. As per the American Cancer Society, the estimated number of cancer incidence in 2017 is 1,688,780 new cancer cases and 600,920 cancer deaths in USA, which implies a potential increase of 70% over the next 2 decades.Citation1,Citation2 Current cancer therapies, such as surgical excision, chemotherapy, radiation therapy, or even combination therapy, have severe limitations. Surgery could result in hurt or injury in the adjacent healthy critical tissues, and both chemotherapy and radiotherapy exhibit violent side effects.Citation3
Recently, photothermal therapy (PTT) has gained a great deal of attention due to its excellent performance in cancer treatment. PTT depends on heat generation upon exposure of tumor cells to near-infrared radiation (NIR).Citation4–Citation6 PTT agents absorb light energy, which causes electron excitation and subsequent non-radiative relaxation. This will lead to gain kinetic energy, which in turn results in heat generation in the medium surrounding the PTT agents.Citation7–Citation9 This PTT approach is considered as non-invasive thermotherapy in which the induced thermal energy develops cell membrane disruption or protein denaturation of the surrounding tumor cells.Citation10
Coupled with that, a major breakthrough in nanotechnology has been developed resulting in the production of various nanomaterials for many applications. Due to their unique optical properties, nano-scaled materials, especially noble metal nanoparticles (NPs), are promising in the nanomedicine and biomedical fields.Citation11–Citation14 The noble metal NPs such as gold (Au) and silver (Ag) have a unique photo-physical phenomenon, called localized surface plasmon resonance (LSPR), which enhances their photo-absorption.Citation15–Citation18 Consequently, they are considered as PTT because of their ability to stimulate hyperthermia in the cancerous cells, when exposed to NIR.Citation19–Citation21 This phenomenon is due to the special optical properties of noble metal NPs, which are able to absorb the light energy in NIR region (ie, 650–900 nm). Then, the absorbed light energy is converted to heat leading to tumor cell damage and tumor tissue necrosis.Citation3
To illustrate, the surface plasmon resonance (SRP) effect means that the metal-free electrons that exist in the conduction band oscillate around the particle surface when exposed to the electromagnetic field of light. Subsequently, this oscillation leads to charge separation and formation of dipole oscillation (). At a certain frequency, the oscillation will reach its maximum which is known as SPR, which supports the light absorption of the NPs, especially the noble metals (ie, Au and Ag).Citation22–Citation25
Figure 1 (A) Schematic description of localized surface plasmon resonance in metal NPs. (B) Dark-field image of NPs. (C) Scattering spectrum of a single NP.
Notes: Reproduced from Xie T, Jing C, Long YT. Single plasmonic nanoparticles as ultrasensi tive sensors. Analyst. 2017;142(3):409–420,Citation25 http://pubs.rsc.org/en/Content/ArticleLanding/2017/AN/C6AN01852A#!divAbstract, with permission of The Royal Society of Chemistry.
Abbreviations: LSPR, localized surface plasmon resonance; NP, nanoparticle.
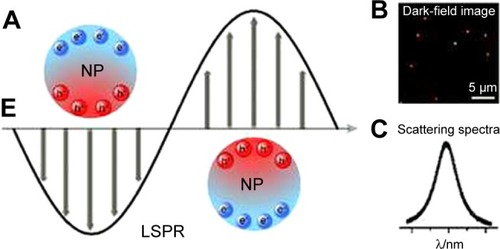
The conversion of the absorbed light to heat proceeds through three main photo-physical processes. The first and second processes allow for the formation of a hot metallic lattice in the metal NPs, and the last one allows for a cooling off, which means heat dissipation from the NPs to the surrounding environment. Accordingly, the dissipated energy will heat up the cancerous cells around the Au nanoparticles (AuNPs) causing cell destruction. However, such energy conversion and dissipation occur when the frequency of the incident light is overlapped with the SPR absorption band.Citation24–Citation27 shows the schematic presentation of the three processes included in the light to heat conversion mechanism.
Figure 2 Schematic illustration of light to heat conversion by plasmonic nanostructures.
Notes: Step 1 is excitation of metal nanoparticles by the absorbed light photons, which results in particle oscillation and charge separation. Step 2 is conversion of the absorbed light to heat through electron–electron relaxation and electron–phonon relaxation processes, which result in the formation of hot metallic lattice. Step 3 is cooling off the metal structure through electron–phonon coupling and phonon–phonon relaxation, which cause heat dissipation. Reproduced from Webb JA, Bardhan R. Emerging advances in nanomedicine with engineered gold nanostructures. Nanoscale. 2014;6(5):2502,Citation27 http://pubs.rsc.org/en/content/articlelanding/2014/nr/c3nr05112a#!divAbstract, with permission of The Royal Society of Chemistry.
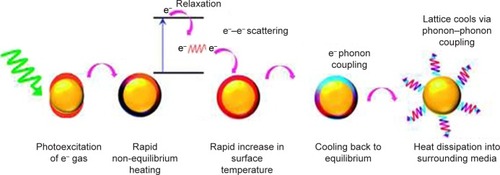
On the other hand, exposure of the healthy cells to electromagnetic radiation of light raises the concern about the safety of this type of therapy. With respect to the LSPR frequency, the incident laser light is generally in the visible region or in the NIR region. In vivo, the radiation can be normally absorbed in the tissue by water, hemoglobin, and melanin.Citation3 However, NIR laser light is weakly absorbed by these absorbents; hence, it can penetrate the skin without causing damage to normal tissues.Citation24,Citation28 Under such circumstances, many researchers have opened the door for the application of several nanomaterials, which strongly absorb the NIR and, as a result of SPR effect, generate sufficient heat to cause tumor cell death.
Functional nanostructures as cancer PTT
In this study, we introduce a recent literature review about nanomaterials used as a cancer PTT over the past 5 years. Different nanostructures developed even from the same material or combination of two or three PTT agents were reported to enhance the efficiency or to decrease the toxicity. For simplicity, this review covers mainly the application of diverse nano-scaled materials, either single or hybrid nanostructures, but does not cover the implication of any therapeutic agent or anticancer drug of the nanomaterials.
Au-based nanomaterials
One of the most promising PTT nanomaterials for cancer treatment is Au nanostructures, as they can be synthesized in different forms such as nanorods, nanoshells, nanocages, or nanospheres. Beyond their plasmonic effect, they have an enhanced permeability to tumor vasculature, resulting in higher retention in tumor tissue. Because the tumor vascular system possesses gaps ranging in size from 100 nm to 2 µm, AuNPs can penetrate easily; these NPs are retained due to lymphatic clearance deficiency in the cancerous cells.Citation18 Although AuNPs can successfully pass into the tumor tissue, they are subjected to phagocytosis by macrophages. Hence, AuNPs are sometimes coated with biocompatible polymers to inhibit reuptake by phagocytic cells.Citation29 In addition, AuNPs were reported as good biocompatible NPs with low cytotoxicity.Citation30 Nanomaterials are unable to differentiate between cancerous cells and normal cells. In some studies, however, AuNPs were conjugated with a specific antibody-targeted cancer receptor to achieve minimal binding with healthy tissues.Citation31–Citation33
The optical properties of different Au nanostructures are greatly affected by altering their shapes and sizes. Research studies used these properties to tune the resonance in the NIR region according to the desired applications.Citation27 In detail, more complex shapes such as Au nanorods (AuNRs), nanoshells, and nanostars exhibited more enhanced LSPR effect than simple AuNPs.Citation34,Citation35 Kelly et alCitation36 demonstrated that complex geometrical shapes improved the polarizability of Au nanostructures due to additional bumps and protrusions. To illustrate, polarizability of AuNPs arises from their diameter only, while polarizability of Au-nanostar was enhanced by their projections, which increase the overall polarizability resulting in red shift in the plasmon resonance wavelength.Citation37 In addition to shape, increasing the size of Au nanostructures enhances the electron oscillation around Au nanostructure leading to increasing the oscillation time and decreasing the frequency. This phenomenon is called phase retardation, which results in prolonged lifetime of plasmon and red shift of the resonance wavelength as well.Citation27,Citation38
Findings from recent research support the application of Au-based nanomaterials as PTT. Ali et alCitation39 studied the effect of AuNRs on human oral squamous cell carcinoma (HSC-3) by analyzing the data from Raman spectroscopy, metabolomics, and proteomics mass spectrometry. They claimed that the AuNR-treated cancer cells exhibited high levels of phenylalanine resulting in cell apoptosis. Similarly, a long-term in vivo study was carried out in mouse tumor tissues to discover the efficiency of AuNRs as PTT agents.Citation40 The authors compared the apoptotic effect induced by gold nanorods conjugated to rifampicin (AuNRs@RF) to the apoptotic effect induced by gold nanorods conjugated to polyethelyne glycole (AuNRs@ PEG). By using proteomics analysis, it was found that AuNRs@ RF has more efficient endocytosis and easier cellular uptake resulting in increased neutrophil cell death (NETosis).
Moreover, AuNRs were found to minimize metastasis via inhibition of cancer cell migration.Citation41 This occurs because AuNR surface, when conjugated to specific peptides, induces changes in migration-related proteins (integrins), which result in controlling cancer metastasis. Another key point is the effect of Au nanomaterial concentration on NIR-induced hyperthermia. Singh et alCitation42 investigated the optimal concentration of AuNRs to be an efficient PTT in vitro using human esophagogastric tissues. They studied different concentrations of AuNRs and variable power densities. Their results showed a linear relationship between temperature elevation and laser power and AuNR concentration.
During treatment with a PTT agent, excessive reactive oxygen species (ROS) could be produced as a result of heat shock.Citation43–Citation45 Thus, ROS can cause protein damage leading to cell death. Sometimes, ROS levels increased in degenerative diseases such as Alzheimer or even in progressive disease such as cancer.Citation46–Citation49 Interestingly, platinum-coated Au nanorods were proved to be used in cancer therapy as a PTT with simultaneous ROS scavenging.Citation50 Platinum shells of varying thickness were examined and showed no interference with the photothermal effect of AuNRs, as well as no impairment of the thermal response to NIR laser waves.
In addition, a new strategy was reported by Wu et alCitation51 to synthesize nanotubes of Au-attapulgite composite, where attapulgite is a natural and biocompatible clay. The nanocomposite was prepared by the assembly of AuNPs on the surface of attapulgite. The authors stated that the synthesized tube-like nanocomposite could act as an efficient PTT when tested using A549 cells (human adenocarcinoma cell). Furthermore, a new Au nanostructure (Au nanobipyramids) was reported to be applied for breast cancer detection and treatment in which Au nano-bipyramids were bio-conjugated with 2-naphthalenethiol and folic acid to target and kill human breast (adenocarcinoma) cells.Citation52 The authors revealed that the conjugated Au nano-bipyramids are capable of quantitative detection of the MCF-7 cells as well as enhancement of photothermal effect in vivo and in vitro.
On the contrary, there are some challenges in the clinical application of Au nanostructures as PTT agents. For instance, lack of stability inside the body and agglomeration of Au nanostructures is the most challenging factor upon in vivo application.Citation27 Some modifications, such as attaching a surface ligand and dispersion in phosphate buffer with suitable pH, could be carried out during synthesis to increase the in vivo stability and decrease the aggregation, respectively.Citation53 However, the use of natural surface ligands results in lower yield from Au nanostructures,Citation54 and the use of high salt or basic buffer can cause surface instability and aggregation of the nanostructures.Citation55
Iron oxide (Fe3O4) NPs
Due to unique magnetism, non-toxicity, biodegradability, and biocompatibility, magnetite Fe3O4 NPs have been widely reported in biomedical applications.Citation56–Citation61 For instance, Fe3O4 NPs were extensively used in drug delivery systems, cell targeting, and in magnetic resonance imaging (MRI) as contrast agents. In addition, they possess a unique surface, which is able to carry targeting molecules for cancer cells and go deeply into tumor tissues.Citation62 Furthermore, Fe3O4 NPs have been proved useful for cancer treatment due to their magnetic hyperthermia in which induced high temperatures were achieved and controlled by alternating magnetic field (AFM).Citation63 The generated heat is sufficient to destroy cancerous cells but may affect healthy tissues. For this reason, the use of NIR laser radiation (widely used in therapy and diagnosis) to induce hyperthermia has an added safety advantage compared to AFM.Citation64
Fe3O4 nanomaterials of different shapes (ie, spherical, hexagonal, and wire-like particles) were investigated for photothermal effect on human esophageal cancer cells (Eca-109). It was found that NIR-induced hyperthermia by spherical Fe3O4 NPs exhibited significant cytotoxicity and suppression of tumor growth.Citation64 Shen et alCitation65 have synthesized both individual and clustered Fe3O4 NPs and investigated their thermo-therapeutic effect in vivo and in vitro. They used human lung adenocarcinoma epithelial (A549) cells for in vitro testing and flow cytometry analysis to investigate the cell apoptosis effect. The clustered Fe3O4 NPs were shown to have higher NIR-induced hyperthermia and increased cell death compared to individual one.
The effect of coating Fe3O4 NPs with an anti-biofouling polymer was studied and compared to commercial magnetic NPs (FeREX, BioPAL) for their anticancer activity.Citation66 The study showed that highly crystallized iron oxide nanoparticles (HCIONPs) offered more potential photothermal effect in vivo and in vitro due to their preferred lattice orientations. Consequently, this approach was suggested for clinical use in cancer treatment. Furthermore, Zhou et alCitation67 developed iron/iron oxide core–shell nanoparticles (Fe@Fe3O4 NPs) that have advantages of NIR photothermal therapeutic effect, selective magnetic targeting, and MRI for complete tumor treatment. Another study investigated the efficiency of FePt NPs functionalized with folates to target breast cancer cells EMT-6.Citation68 The findings revealed that the FePt NP exhibited intracellular explosions accompanied by rupture of cancerous cell membranes.
The main limitation of most studies is the use of highly intense laser irradiation, which might exceed the safe limit for cutaneous tissues (0.33 W/cm2). However, Espinosa et alCitation69 mitigated this drawback and reported the dual magnetic and photothermal activity of Fe3O4 nanocubes. The authors applied a magnetic field in alternating patterns and NIR laser of reasonable irradiation density (0.3 W/cm2). Such dual approach showed complete cell death and tumor ablation even at low iron doses.
Organic nanomaterials
Owing to their enhanced biocompatibility and low toxicity, organic nanomaterials have recently been reported for a wide variety of biomedical applications.Citation70 In particular, they can be used as PTT agents because they exhibit an efficient light-to-heat conversion. Moreover, organic materials have a strong NIR absorption resulting from their diffused bi-electrons.Citation71 Therefore, reduced graphene oxide (rGO) nanosheets as an example have a high extension coefficient (3.6 L g−1cm−1).Citation72 Carbon-based nanomaterials (ie, carbon nanotubes and graphene), organic dyes, and polymer nanocarriers are the most widely reported organic-based photothermal nanoagents.
Liang et alCitation73 have synthesized PEGylated single-walled carbon nanotubes (SWCNTs) and demonstrated their efficiency as a PTT. They investigated the therapeutic effect of PEGylated SWCNTs on metastasis of primary 4T1 murine breast tumor to lymph node. The findings showed the ability of PEGylated SWCNTs to suppress the primary tumor and the pulmonary metastasis. Similarly, a novel SWCNT-peptide composite showed an effective photothermal effect on colon 26 and HepG2 cells after 3 minutes of NIR radiation.Citation74
Graphene, as a carbon-based nanomaterial, has biomedical applications such as biosensors, drug carriers, and a very recent application as a PTT due to its strong NIR absorption.Citation75–Citation77 However, graphene is still considered to exhibit some biological toxicity because of poor dispersibility in physiological fluids. Consequently, most of the graphene-based PTT agents were modified with surface functionalization to mitigate the bio-toxicity limitation.Citation77–Citation79 To figure out photothermal efficiency of graphene and graphene oxide (GO), their ability to produce heat after laser irradiation was investigated and compared to AuNRs using an integrating sphere method and the time constant method.Citation80
Graphene or GO as two-dimensional (2D) nanosheets with large surface area can be mixed with certain functional NPs for diagnostic and therapeutic purposes.Citation81 For example, BaGdF5 NPs attached to the PEG-coated GO surface were fabricated by Zhang et alCitation82 for both MRI and photothermal cancer therapy. The synthesized nanocomposites were injected into mice bearing HeLa tumor and irradiated for 10 minutes with 808 nm laser (power density of 0.5 W/cm2). When the tumor tissue samples were collected for histological examination, the results showed that the treated group acquired thermonecrosis and cell shrinkage. Chen et alCitation83 developed radiolabeled reduced GO coated with PEG (131I-rGO-PEG) for combined radiotherapy and PTT. The results showed high synergistic effect on 4T1 murine breast cancer therapy due to the combination between radioactive 131I and strong NIR absorption of PEG-coated rGO as well as no apparent animal toxicity.
The literature revealed many articles concerned with the use of organic dyes such as cyanine and porphyrin derivatives as efficient photothermal agents. The most common cyanine derivative indocyanine green (ICG) dye, which was approved by the US Food and Drug Administration for clinical use, was reported in combination with nanocarriers as a PTT with a good efficiency in vitro and in vivo for tumor ablation.Citation84–Citation86 The nanocarriers containing ICG dye showed enhanced aqueous stability and better cellular uptake compared to the direct dyes. However, most of these studies have poor photostability at high-power laser irradiation, which means limited use to preclinical studies.Citation70 Similarly, porphyrin-based nanomaterials showed its activity as PTT for the treatment of different tumors as well as their biodegradability and biocompatibility.Citation87 However, it is important to mention that porphyrin nanoagents exhibit short absorption wavelength (600–700 nm), which restricts their in vivo application.Citation70
In the last decade, NPs of conductive polymers have been reported for biomedical applications due to their high stability and biocompatibility. In particular, polypyrrole (PPy) and poly-(3,4-ethylenedioxythiophene):poly(4-styrenesulfonate) (PEDOT:PSS) have strong NIR absorbance, making them suitable as photothermal agents for cancer therapy. Both PPy and PEDOT:PSS were examined for tumor ablation in vivo and in vitro, showing high efficiency without undesired effects.Citation88–Citation91 However, the clinical use of polymer NPs is still limited due to lack of safety data.Citation70
Combined nanomaterials
In general, the combination between two PTT agents to synthesize nanocomposites or core–shell NPs provides novel physical and chemical properties.Citation92 In addition, these nanocomposites act as multi-function nanomaterials with tunable size and composition. The optical properties were enhanced while using two or more nanostructures to synthesize a hybrid PTT.Citation93 Therefore, strong plasmonic effect and surface-enhanced Raman scattering were reported for many combined PTT.
Hybrid NPs consisting of Au and Fe3O4 were synthesized to get new and unique physical and chemical properties. The plasmonic effect was investigated by using optical absorption measurements such as UV-visCitation92 and surface-enhanced Raman spectra.Citation93 Owing to their potential application as PTT, the optical properties of Fe3O4@Au core–shell were simulated by using the extended Mie theory.Citation94–Citation96 Investigations of absorption cross-section spectra as well as the relationship between the resonance wavelength and the elevated temperature were conducted.Citation97 In addition, Ahmed et alCitation98 studied the in vitro cell viability of Fe3O4@AuNPs by using the MTT assay. They found 50 µg/mL of Fe3O4@AuNPs to be non-toxic and biocompatible.
Another example of hybrid therapy is the combination between ICG organic dye and Fe3O4 NPs investigated by Niu et al.Citation99 The authors used the two synergistic PTT agents encapsulated in perfluoropentane-based polylactide-co-glycolide (Fe3O4/ICG@PLGA/PFP NPs) and evaluated their efficiency toward tumor ablation. When Fe3O4/ICG@PLGA/PFP NPs were incubated with MCF-7 human breast cancer cells and irradiated with 808 nm laser for 5 minutes, they showed tissue deformation and significant cell death. Furthermore, effectiveness of this dual NIR therapy was assessed in vivo by using mice bearing MCF-7 cells. The results revealed reduced tumor size and inhibition of tumor growth with no significant toxicity in vital organs such as liver, kidney, heart, spleen, and brain.
Meng et alCitation100 developed golden SWCNT hybrids with enhanced NIR absorption, excellent water dispersibility, and better biocompatibility. The novel hybrids were synthesized by layer-by-layer self-assembly of two different polysaccharides on the SWCNT skeleton, followed by growing AuNPs on the surface. Highly effective photothermal activity was achieved, when the golden SWCNTs were incubated overnight with HeLa cells and irradiated for 15 minutes with 808 nm laser diode.
In addition, graphene-based hybrid PTT has been recently reported to have the advantages of carbon-based materials and metal NPs. For example, combination of GO with AuNPs revealed an enhanced tumor ablation in vivo and in vitro at lower laser irradiation (0.75 W/cm2).Citation101 Similarly, reduced GO/AuPd NPs/PEG-coated nanocomposites were evaluated for the suppression of HeLa cells in vitro showing good results after laser irradiation for 10 minutes.Citation102 For the purpose of improving light-to-heat conversion as well as targeting the tumor tissues, combined GO/magnetic iron NP hybrids were also reported in the literature.Citation81,Citation103 However, laser-induced hyperthermia in these articles were substituted by magnetic hyperthermia, which is induced by AFM to mitigate the limited penetration of NIR into the tissues.Citation104
Nanocomposites of AuNRs coated with pyrrole conductive polymer have gained a great interest due to high photostability and biocompatibility in addition to improved photothermal activity.Citation105 Likewise, Au/pyrrole NPs with Fe3O4 NPs deposited on the surface were synthesized by Feng et alCitation106 for cancer therapy. The Au/pyrrole@Fe3O4 nanocomposites were considered to be multifunctional nanoagents with enhanced photothermal conversion, superparamagnetic properties, and good biocompatibility.
Novel 2D materials
New family of 2D nanosheets have been recently reported in the literature for many biomedical applications. Owing to high biocompatibility, excellent physicochemical performance as well as unique photothermal conversion efficiency, various 2D nanostructures are considered to be promising PTT agents for cancer therapy.Citation107 MXenes are recently reported but widely spread new 2D materials, having a general formula of Mn+1Xn, where M represents a transition metal and X represents carbon or nitrogen.Citation108,Citation109 One of the most powerful 2D PTT nanoagents is Ti3C2 (MXene), which shows strong NIR absorption and high light-to-heat conversion property. To explain, the exfoliated ultrathin MXene nanosheets demonstrate semimetal-like band energy structure; consequently, they have the LSPR effect similar to metal NPs.Citation110 The same condition is applied for the transition of metal dichalcogenides such as tungsten sulfide (WS2), molybdenum sulfide (MoS2), and titanium sulfide (TiS2) as well as black phosphorus.Citation111,Citation112
Lin et alCitation110 synthesized phospholipid-stabilized ultrathin Ti3C2 nanosheets (MXene) by prolonged etching of Ti3AlC2 using hydrofluoric acid (HF), followed by intercalation with tetrapropylammonium hydroxide (TPAOH) and surface modification with soybean phospholipids (SP). They evaluated the cytotoxicity of Ti3C2-SP nanoflakes and the tumor ablation ability in vivo and in vitro by using 4T1 tumor-bearing mice and murine 4T1 breast cancer cells, respectively. The results showed that the groups treated with the stabilized MXenes revealed high percentage of 4T1 cancer cell death in vitro and significant decrease of tumor size in vivo after exposure to NIR laser irradiation for few minutes. Similarly, by using the same mouse model, MXene-based hybrids such as MnOx-Ti3C2 composites were prepared and investigated for tumor ablation and MRI by Dai et al.Citation113
summarizes different nano-scaled PTT agents applied for different types of cancer cells. From , it is clearly observed that the combination between two or more PTT agents contributed in reducing the power densities of laser irradiation near to the safety limit in addition to decreasing the exposure radiation time.
Table 1 Summary of different nanomaterials reported as PTT agents applied on various types of tumor cells
Conclusion and future perspective
Throughout this review, new insights for cancer treatment have been addressed, focusing on the promising benefits of employing the PTT types. It is evident from ineffective conventional treatments that there is a desperate necessity for distinct and unique treatments. As extensively explained, nanostructure technology has the potential to play critical functions in cancer therapy, through directly acting as PTT. Of note, nanostructures with various shapes were revealed as PTT due to their strong NIR absorption and efficient light-to-heat conversion. Investigation through various nanostructure studies revealed that the mentioned concepts attempt to strengthen their overall photothermal therapeutic behavior. As represented by numerous reports, therapies including AuNPs, Fe3O4 NPs, organic nanomaterials, and novel 2D nanoagents have the potential to directly enhance the in vivo and in vitro cancer therapies. In addition, hybrid nanomaterials with two or more PTT agents have superior advantages of synergism, which leads to enhanced efficiency and reduced toxicological effects of every single therapy. To sum up, nanostructures are considered promising candidates for tumor ablation as PTT agents due to their unique optical properties. However, in vivo stability and more detailed toxicity studies should be covered in the future for the PTT nanoagents to be clinically approved.
Acknowledgments
The authors gratefully acknowledge Qatar University for Graduate Assistantship and they would also like to show gratitude to Dr Mary Newsome (Office of Graduate Studies, Qatar University) for discussions and useful suggestions of writing this paper. The authors would also like to acknowledge Qatar University for funding the project: GCC Co-Fund Program Grant GCC-2017-001.
Disclosure
The authors report no conflicts of interest in this work.
References
- SiegelRLMillerKDJemalACancer statistics, 2017CA Cancer J Clin201767173028055103
- Cancer Fact SheetWorld Health Organization2017 Available from: http://www.who.int/mediacentre/factsheets/fs297/en/Accessed December 12, 2017
- DayESMortonJGWestJLNanoparticles for thermal cancer therapyJ Biomech Eng2009131774001
- MelanconMPZhouMLiCCancer theranostics with near-infrared light-activatable multimodal nanoparticlesAcc Chem Res2011441094795621848277
- KennedyLCBickfordLRLewinskiNAA new era for cancer treatment: Gold-nanoparticle-mediated thermal therapiesSmall20117216918321213377
- YangKFengLShiXLiuZNano-graphene in biomedicine: theranostic applicationsChem Soc Rev201342253054723059655
- CamerinMRelloSVillanuevaAPhotothermal sensitisation as a novel therapeutic approach for tumours: studies at the cellular and animal levelEur J Cancer20054181203121215911245
- CamerinMRodgersMAJKenneyMEJoriGPhotothermal sensitization: evidence for the lack of oxygen effect on the photosensitizing activityPhotochem Photobiol Sci20054325125315738991
- HeXBischofJCQuantification of temperature and injury response in thermal therapy and cryosurgeryCrit Rev Biomed Eng2003315–635542215139301
- van der ZeeJHeating the patient: a promising approach?Ann Oncol20021381173118412181239
- El-SayedMASome interesting properties of metals confined in time and nanometer space of different shapesAcc Chem Res200134425726411308299
- NiemeyerCMNanoparticles, proteins, and nucleic acids: biotechnology meets materials scienceAngew Chem200140224128415829712109
- WestJLHalasNJEngineered nanomaterials for biophotonics applications: improving sensing, imaging, and therapeuticsAnnu Rev Biomed Eng20035128529214527314
- KatzEWillnerIIntegrated nanoparticle-biomolecule hybrid systems: synthesis, properties, and applicationsAngew Chem200443456042610815538757
- DreadenECAlkilanyAMHuangXMurphyCJEl-SayedMAThe golden age: gold nanoparticles for biomedicineChem Soc Rev20124172740277922109657
- DreadenECMackeyMAHuangXKangBEl-SayedMABeating cancer in multiple ways using nanogoldChem Soc Rev2011407339121629885
- HuangXNeretinaSEl-SayedMAGold nanorods: from synthesis and properties to biological and biomedical applicationsAdv Mater200921484880491025378252
- LeeJChatterjeeDKLeeMHKrishnanSGold nanoparticles in breast cancer treatment: promise and potential pitfallsCancer Lett20143471465324556077
- JainPKHuangXEl-SayedIHEl-SayedMANoble metals on the nanoscale: optical and photothermal properties and some applications in imaging, sensing, biology, and medicineAcc Chem Res200841121578158618447366
- HuangXJainPKEl-SayedIHEl-SayedMAGold nanoparticles: interesting optical properties and recent applications in cancer diagnostics and therapyNanomedicine20072568169317976030
- HuangXJainPKEl-SayedIHEl-SayedMAPlasmonic photothermal therapy (PPTT) using gold nanoparticlesLasers Med Sci200823321722817674122
- JainPKLeeKSEl-SayedIHEl-SayedMACalculated absorption and scattering properties of gold nanoparticles of different size, shape, and composition: applications in biological imaging and biomedicineJ Phys Chem B2006110147238724816599493
- HuangXEl-SayedMAGold nanoparticles: optical properties and implementations in cancer diagnosis and photothermal therapyJ Adv Res2010111328
- HuangXEl-SayedMAPlasmonic photo-thermal therapy (PPTT)Alexandria J Med201147119
- XieTJingCLongYTSingle plasmonic nanoparticles as ultrasensitive sensorsAnalyst2017142340942028004043
- LinkSEl-SayedMAOptical properties and ultrafast dynamics of metallic nanocrystalsAnnu Rev Phys Chem200354133136612626731
- WebbJABardhanREmerging advances in nanomedicine with engineered gold nanostructuresNanoscale201465250224445488
- KimJParkSLeeJEDesigned fabrication of multifunctional magnetic gold nanoshells and their application to magnetic resonance imaging and photothermal therapyAngew Chem20061184679187922
- KahJCYWongKYNeohKGCritical parameters in the pegylation of gold nanoshells for biomedical applications: an in vitro macrophage studyJ Drug Target200917318119319016072
- HuMChenJLiZ-YGold nanostructures: engineering their plasmonic properties for biomedical applicationsChem Soc Rev200635111084109417057837
- LoweryARGobinAMDayESHalasNJWestJLImmunonanoshells for targeted photothermal ablation of tumor cellsInt J Nanomedicine20061214915417722530
- WuP-CShiehD-BChengF-YNanomaterial-mediated photothermal cancer treatment: the pivotal role of cellular uptake on photothermal therapeutic efficacyRSC Adv20144955329753306
- LooCLoweryAHalasNWestJDrezekRImmunotargeted nanoshells for integrated cancer imaging and therapyNano Lett20055470971115826113
- YuanHKhouryCGWilsonCMGrantGABennettAJVo-DinhTIn vivo particle tracking and photothermal ablation using plasmon-resonant gold nanostarsNanomedicine2012881355136322370335
- YeXGaoYChenJRelfsnyderDCZhengCMurrayCBSeeded growth of monodisperse gold nanorods using bromide-free surfactant mixturesNano Lett20131352163217123547734
- KellyKLCoronadoEZhaoLLSchatzGCThe optical properties of metal nanoparticles: the influence of size, shape, and dielectric environmentJ Phys Chem B20031073668677
- BurdaCChenXNarayananREl-SayedMAChemistry and properties of nanocrystals of different shapesChem Rev200510541025110215826010
- RingeELangilleMRSohnKPlasmon length: a universal parameter to describe size effects in gold nanoparticlesJ Phys Chem Lett20123111479148326285624
- AliMRKWuYHanTSimultaneous time-dependent surface-enhanced Raman spectroscopy, metabolomics, and proteomics reveal cancer cell death mechanisms associated with gold nanorod photothermal therapyJ Am Chem Soc201613847154341544227809520
- AliMRKRahmanMAWuYEfficacy, long-term toxicity, and mechanistic studies of gold nanorods photothermal therapy of cancer in xenograft miceProc Natl Acad Sci U S A201711415E3110E311828356516
- AliMRKWuYTangYTargeting cancer cell integrins using gold nanorods in photothermal therapy inhibits migration through affecting cytoskeletal proteinsProc Natl Acad Sci U S A201711428E5655E566328652358
- SinghMHarris-BirtillDCCZhouYApplication of gold nanorods for photothermal therapy in ex vivo human oesophagogastric adenocarcinomaJ Biomed Nanotechnol201612348149027280246
- SlimenIBNajarTGhramADabbebiHBen MradMAbdrabbahMReactive oxygen species, heat stress and oxidative-induced mitochondrial damage. A reviewInt J Hyperth2014307513523
- FedyaevaAVStepanovAVLyubushkinaIVPobezhimovaTPRikhvanovEGHeat shock induces production of reactive oxygen species and increases inner mitochondrial membrane potential in winter wheat cellsBiochem201479111202121025540005
- GaoLLiuRGaoFWangYJiangXGaoXPlasmon-mediated generation of reactive oxygen species from near-infrared light excited gold nanocages for photodynamic therapy in vitroACS Nano2014877260727124992260
- BenzCCYauCAgeing, oxidative stress and cancer: paradigms in parallaxNat Rev Cancer2009811875879
- UttaraBSinghAZamboniPMahajanROxidative stress and neurodegenerative diseases: a review of upstream and downstream antioxidant therapeutic optionsCurr Neuropharmacol200971657419721819
- SchieberMChandelNSROS function in redox signaling and oxidative stressCurr Biol20142410R453R46224845678
- CervellatiFCervellatiCRomaniAHypoxia induces cell damage via oxidative stress in retinal epithelial cellsFree Radic Res201448330331224286355
- AioubMPanikkanvalappilSREl-SayedMAPlatinum-coated gold nanorods: efficient reactive oxygen scavengers that prevent oxidative damage toward healthy, untreated cells during plasmonic photothermal therapyACS Nano201711157958628029783
- WuPDengDGaoJCaiCTube-like gold sphere-attapulgite nanocomposites with a high photothermal conversion ability in the near-infrared region for enhanced cancer photothermal therapyACS Appl Mater Interfaces2016816102431025227054373
- FengJChenLXiaYBioconjugation of gold nanobipyramids for SERS detection and targeted photothermal therapy in breast cancerACS Biomater Sci Eng201734608618
- NiidomeTYamagataMOkamotoYPEG-modified gold nanorods with a stealth character for in vivo applicationsJ Control Release2006114334334716876898
- MaYChechikVAging of gold nanoparticles: ligand exchange with disulfidesLangmuir20112723144321443721985439
- WangZLeeJHLuYLabel-free colorimetric detection of lead ions with a nanomolar detection limit and tunable dynamic range by using gold nanoparticles and DNAzymeAdv Mater2008201732633267
- LaurentSMahmoudiMSuperparamagnetic iron oxide nanoparticles: promises for diagnosis and treatment of cancerInt J Mol Epidemiol Genet20112436739022199999
- Maier-HauffKUlrichFNestlerDEfficacy and safety of intratumoral thermotherapy using magnetic iron-oxide nanoparticles combined with external beam radiotherapy on patients with recurrent glioblastoma multiformeJ Neurooncol2011103231732420845061
- AnselmoACMitragotriSA review of clinical translation of inorganic nanoparticlesAAPS J20151751041105425956384
- LartigueLAlloyeauDKolosnjaj-TabiJBiodegradation of iron oxide nanocubes: high-resolution in situ monitoringACS Nano2013753939395223634880
- LeeJ-HJangJChoiJExchange-coupled magnetic nanoparticles for efficient heat inductionNat Nanotechnol20116741842221706024
- YangHWHuaMYLiuHLSelf-protecting core-shell magnetic nanoparticles for targeted, traceable, long half-life delivery of BCNU to gliomasBiomaterials201132276523653221645920
- SohailAAhmadZBégOAArshadSSherinLA review on hyperthermia via nanoparticle-mediated therapyBull Cancer2017104545246128385267
- RosensweigREHeating magnetic fluid with alternating magnetic fieldJ Magn Magn Mater20022521–3 SPEC. ISS370374
- ChuMShaoYPengJNear-infrared laser light mediated cancer therapy by photothermal effect of Fe3O4magnetic nanoparticlesBiomaterials201334164078408823465836
- ShenSWangSZhengRMagnetic nanoparticle clusters for photothermal therapy with near-infrared irradiationBiomaterials201539677425477173
- ChenHBurnettJZhangFZhangJPaholakHSunDHighly crystallized iron oxide nanoparticles as effective and biodegradable mediators for photothermal cancer therapyJ Mater Chem B201427757765
- ZhouZSunYShenJIron/iron oxide core/shell nanoparticles for magnetic targeting MRI and near-infrared photothermal therapyBiomaterials201435267470747824881997
- ChenCLKuoLRLeeSYPhotothermal cancer therapy via femtosecond-laser-excited FePt nanoparticlesBiomaterials20133441128113423137396
- EspinosaADi CoratoRKolosnjaj-TabiJFlaudPPellegrinoTWilhelmCDuality of iron oxide nanoparticles in cancer therapy: amplification of heating efficiency by magnetic hyperthermia and photothermal bimodal treatmentACS Nano20161022436244626766814
- WangJQiuJA review of organic nanomaterials in photothermal cancer therapyCancer Res Front2016216784
- YangKXuHChengLSunCWangJLiuZIn vitro and in vivo near-infrared photothermal therapy of cancer using polypyrrole organic nanoparticlesAdv Mater201224415586559222907876
- RobinsonJTTabakmanSMLiangYUltrasmall reduced graphene oxide with high near-infrared absorbance for photothermal therapyJ Am Chem Soc2011133176825683121476500
- LiangCDiaoSWangCTumor metastasis inhibition by imaging-guided photothermal therapy with single-walled carbon nanotubesAdv Mater201426325646565224924258
- HashidaYTanakaHZhouSPhotothermal ablation of tumor cells using a single-walled carbon nanotube-peptide compositeJ Control Release201417315866
- JinYWangJKeHWangSDaiZGraphene oxide modified PLA microcapsules containing gold nanoparticles for ultrasonic/CT bimodal imaging guided photothermal tumor therapyBiomaterials201334204794480223557859
- ChenYWChenPJHuSHChenIWChenSYNIR-triggered synergic photo-chemothermal therapy delivered by reduced graphene oxide/carbon/mesoporous silica nanocookiesAdv Funct Mater2014244451459
- GonçalvesGGraphene-Based Materials in Health and EnvironmentVila GGPMMNew YorkSpringer2016
- BussyCAli-BoucettaHKostarelosKSafety considerations for graphene: lessons learnt from carbon nanotubesAcc Chem Res201346369270123163827
- KostarelosKNovoselovKSExploring the interface of graphene and biologyScience2014344618126126324744363
- SavchukOACarvajalJJMassonsJAguilóMDíazFDetermination of photothermal conversion efficiency of graphene and graphene oxide through an integrating sphere methodCarbon2016103134141
- YangKHuLMaXMultimodal imaging guided photothermal therapy using functionalized graphene nanosheets anchored with magnetic nanoparticlesAdv Mater201224141868187222378564
- ZhangHWuHWangJGraphene oxide-BaGdF5 nanocomposites for multi-modal imaging and photothermal therapyBiomaterials201542667725542794
- ChenLZhongXYiXRadionuclide 131I labeled reduced graphene oxide for nuclear imaging guided combined radio- and photothermal therapy of cancerBiomaterials201566212826188609
- GongHDongZLiuYEngineering of multifunctional nano-micelles for combined photothermal and photodynamic therapy under the guidance of multimodal imagingAdv Funct Mater20142464926502
- JianWYuTChenCIndocyanine green-encapsulated hybrid polymeric nanomicelles for photothermal cancer therapyLangmuir2015316202621025985856
- KumarPSrivastavaRIR 820 dye encapsulated in polycaprolactone glycol chitosan: Poloxamer blend nanoparticles for photo immunotherapy for breast cancerMater Sci Eng C201557321327
- JinCSLovellJFChenJZhengGAblation of hypoxic tumors with not photodynamic, therapy using a nanostructured porphyrin assemblyACS Nano2013732541255023394589
- ChengLYangKChenQLiuZOrganic stealth nanoparticles for highly effective in vivo near-infrared photothermal therapy of cancerACS Nano2012665605561322616847
- ChenMFangXTangSZhengNPolypyrrole nanoparticles for high-performance in vivo near-infrared photothermal cancer therapyChem Commun201248718934
- ZhaZWangJQuEPolypyrrole hollow microspheres as echo-genic photothermal agent for ultrasound imaging guided tumor ablationSci Rep2013318
- LiangXLiYLiXPEGylated polypyrrole nanoparticles conjugating gadolinium chelates for dual-modal MRI/photoacoustic imaging guided photothermal therapy of cancerAdv Funct Mater20152514511462
- YuHChenMRicePMWangSXWhiteRLSunSDumbbell-like bifunctional Au−Fe3O4 nanoparticlesNano Lett20055237938215794629
- MezniABaltiIMlayahAHybrid Au-Fe3O4 nanoparticles: Plasmonic, surface enhanced Raman scattering, and phase transition propertiesJ Phys Chem C2013117311616616174
- BrullotWValevVKVerbiestTMagnetic-plasmonic nanoparticles for the life sciences: calculated optical properties of hybrid structuresNanomedicine20128555956821945901
- ChaffinEABhanaSO’ConnorRTHuangXWangYImpact of core dielectric properties on the localized surface plasmonic spectra of gold-coated magnetic core-shell nanoparticlesJ Phys Chem B201411849140761408425010347
- LiQZhangWZhaoDQiuMPhotothermal enhancement in core-shell structured plasmonic nanoparticlesPlasmonics201493623630
- EncinaERCoronadoEASize optimization of iron oxide@noble metal core-shell nanohybrids for photothermal applicationsJ Phys Chem C20161201056305639
- AhmedOSMohamedMBZekriARNImamHKhaledHMAbdel-kaderMHSynthesis of the newly developed core-shell Au/Fe3O4 magnato-plasmonic nanocomposite in cancer cellsLife Sci J20141110182187
- NiuCXuYAnSNear-infrared induced phase-shifted ICG/Fe3O4 loaded PLGA nanoparticles for photothermal tumor ablationSci Rep20177111028127051
- MengLXiaWLiuLNiuLLuQGolden single-walled carbon nanotubes prepared using double layer polysaccharides bridge for photo-thermal therapyACS Appl Mater Interfaces2014674989499624606763
- GaoSZhangLWangGHybrid graphene/Au activatable theranostic agent for multimodalities imaging guided enhanced photothermal therapyBiomaterials201679364526691399
- DarabdharaGDasMRTurcheniukVReduced graphene oxide nanosheets decorated with AuPd bimetallic nanoparticles: a multifunctional material for photothermal therapy of cancer cellsJ Mater Chem B201534283668374
- AkhavanOMeidanchiAKhoeiSZinc ferrite spinel-graphene in magneto-photothermal therapy of cancerJ Mater Chem B201422133063314
- CriadoAPratoMRecent advances of graphene-based hybrids with magnetic nanoparticles for biomedical applicationsCurr Med Chem201724552953627993110
- DuCWangAFeiJZhaoJLiJPolypyrrole-stabilized gold nanorods with enhanced photothermal effect towards two-photon photothermal therapyJ Mater Chem B201532245394545
- FengWZhouXNieWAu/polypyrrole @ Fe3O4 nanocomposites for MR/CT dual-modal imaging guided-photothermal therapy: an in vitro studyACS Appl Mater Interfaces2015774354436725664659
- ChenYWangLShiJTwo-dimensional non-carbonaceous materials- enabled efficient photothermal cancer therapyNano Today2016113292308
- NaguibMKurtogluMPresserVTwo-dimensional nanocrystals produced by exfoliation of Ti 3AlC 2Adv Mater201123374248425321861270
- KarlssonLHBirchJHalimJBarsoumMWPerssonPOAtomically resolved structural and chemical investigation of single MXene sheetsNano Lett20151584955496026177010
- LinHWangXYuLChenYShiJTwo-dimensional ultrathin MXene ceramic nanosheets for photothermal conversionNano Lett201717138439128026960
- ChengLLiuJGuXPEGylated WS2 nanosheets as a multifunctional theranostic agent for in vivo dual-modal CT/photoacoustic imaging guided photothermal therapyAdv Mater201426121886189324375758
- ZhuZZouYHuWNear-infrared plasmonic 2D semimetals for applications in communication and biologyAdv Funct Mater2016261117931802
- DaiCLinHXuGLiuZWuRChenYBiocompatible 2D titanium carbide (MXenes) composite nanosheets for pH-responsive MRI-guided tumor hyperthermiaChem Mater2017292086378652
- LiWRongPYangKHuangPSunKChenXSemimetal nanomaterials of antimony as highly efficient agent for photoacoustic imaging and photothermal therapyBiomaterials201545182625662491
- YuJJuYZhaoLMultistimuli-regulated photochemothermal cancer therapy remotely controlled via Fe5C2nanoparticlesACS Nano201610115916926602632
- ZhouZKongBYuCTungsten oxide nanorods: An efficient nanoplatform for tumor CT imaging and photothermal therapySci Rep20144110