Abstract
Background
Cardiovascular and kidney diseases are a global public health problem and impose a huge economic burden on health care services. Homocysteine, an amino acid, is associated with coronary heart disease, while urea is a harmful metabolic substance which can be used to reflect kidney function. Monitoring of these two substances is therefore very important. This in vitro study aimed to determine whether homocysteine is extractable transdermally and noninvasively, and whether homocysteine and urea can be extracted simultaneously by reverse iontophoresis.
Methods
A diffusion cell incorporated with porcine skin was used for all experiments with the application of a direct current (dc) and four different symmetrical biphasic direct currents (SBdc) for 12 minutes via Ag/AgCl electrodes. The dc and the SBdc had a current density of 0.3 mA/cm2.
Results
The SBdc has four different phase durations of 15 sec, 30 sec, 60 sec, and 180 sec. It was found that homocysteine can be transdermally extracted by reverse iontophoresis. Simultaneous extraction of homocysteine and urea by reverse iontophoresis is also possible.
Conclusion
These results suggest that extraction of homocysteine and urea by SBdc are phase duration-dependent, and the optimum mode for simultaneous homocysteine and urea extraction is the SBdc with the phase duration of 60 sec.
Introduction
Cardiovascular disease is a challenging global public health problem, causing about 16.7 million deaths annually worldwide.Citation1 Meanwhile, kidney disease is reportedly increasing in developed countries, including the US, Canada, Australia, and Europe.Citation2,Citation3 Cardiovascular disease and kidney disease impose a substantial economic burden, not just on health care services but also on industry and commerce. Taiwan also faces these challenges, with heart disease and kidney disease being, respectively, the second and eighth leading causes of death in 2007.Citation4 Therefore, there is a need to reduce the occurrence and death rate by means of frequent monitoring of patients who are at high risk of developing cardiovascular disease or kidney disease. On the other hand, a large clinical study has shown that the increased risk of heart disease across the spectrum of kidney disease has been recently appreciated by the medical community.Citation5,Citation6 Therefore, simultaneous monitoring of cardiovascular disease and kidney disease is essential.
Homocysteine is an amino acid. Highly elevated homocysteine levels were associated with a more than three-fold increase in the risk of heart attack over a five-year period.Citation5 Therefore, the blood homocysteine level is a good index for heart attack monitoring. Conversely, urea in the plasma increases with the degree of renal failure and accumulates in kidney disease.Citation6 Therefore, the clinical diagnosis of chronic kidney disease is principally based on the measurement of increased levels of urea.
Blood sampling is the current routine clinical method to determine blood homocysteine and urea levels. However, this method is invasive, painful, aesthetically unpleasant, and inconvenient. This provides considerable impetus for the development of noninvasive methods for the simultaneous monitoring of blood homocysteine and urea levels. So far, no research has been reported on investigation of transdermal extraction of homocysteine by reverse iontophoresis. Several papers have reported on the use of reverse iontophoresis to enhance the extraction of urea,Citation7–Citation9 but no papers have been found for the simultaneous transdermal extraction of urea and homocysteine by this method.
Reverse iontophoresis refers to the passage of a low voltage (≤10 V) and low level of current (≤0.5 mA/cm2) through the skin to promote the extraction of both charged and neutral molecules.Citation10 The most successful reverse iontophoresis application for patient monitoring to date, as mentioned, has been in noninvasive and continuous blood glucose detection utilizing the GlucoWatch®.Citation11,Citation12
The aim of this study was to find out whether homocysteine is extractable transdermally and noninvasively, and whether homocysteine and urea can be extracted simultaneously by reverse iontophoresis.
Materials and methods
Reagents and solutions
All reagents used in this study were commercially available and used without further purification. Phosphate-buffered saline, urea, and homocysteine were purchased from Sigma Chemical Company (St Louis, MO). The urea assay kit was purchased from BioVision (Mountain View, CA). The homocysteine assay kit was purchased from IBL-America (Minneapolis, MN). Deionized water, purified by a Millipore Milli-Q UFplus System (Bedford, MA) was used to prepare all solutions.
Equipment
The diffusion cell used for the in vitro studies was the same as that previously described ().Citation13 A reverse iontophoresis device was homemade, with an accuracy of ±0.01 μA for delivering current and ±0.01 msec for timing. All colorimetric analysis was performed using a Bio-Rad 680 microplate reader (Bio-Rad, Hemel Hempstead, UK).
In vitro studies
The diffusion cell was used for all experiments. It consisted of two upper electrode chambers and a lower chamber. The electrode chambers were separated from the lower chamber by porcine ear skin with a thickness of 250 μm, obtained by Padgett Dermatome (Integra LifeSciences Corporation, Plainsboro, NJ). The thickness of the skin used in this study was the same as that reported in other researchers’ diffusion studies.Citation17,Citation18 All chambers were filled with 0.1 M phosphate buffer (pH 7.0), except that the lower chamber additionally contained 5 mM urea and 15 μM homocysteine, which attempted to simulate the normal physiological levels of urea and homocysteine in human blood. Each upper electrode chamber contained 300 μL phosphate buffer (0.1 M, pH 7.0) while the lower chamber contained the necessary solution of about 25 mL. A Ag/AgCl electrode was positioned inside each electrode chamber, and its fabrication procedure was the same as previously described.Citation13 The surface area of the porcine skin exposed to the electrode in each chamber was 0.2 cm2 and the electrode chambers were 11 mm apart.
A direct current (dc, cathodal dc abbreviated to dc-cathode and anodal dc abbreviated to dc-anode) and four symmetrical biphasic direct currents (SBdc) were applied for 12 minutes and 36 minutes via the Ag/AgCl electrodes. The dc and the SBdc had a current density of 0.3 mA/cm2. The four SBdc had four different phase durations of 15 sec (15s PDSBdc), 30 sec (30s PDSBdc), 60 sec (60s PDSBdc), and 180 sec (180s PDSBdc). At the end of each experiment, the entire contents of the electrode chambers were removed to determine the amount of urea and homocysteine extracted.
For the control experiments, all the experimental arrangements and procedures were the same, except no current was applied.
Determination of extracted urea and homocysteine
The amount of urea (determined by the urea assay kit) and homocysteine (determined by the homocysteine assay kit) extracted through the porcine skin were quantified by a colorimetric clinical chemistry assay method adapted for use with the Bio-Rad 680 microplate reader. To perform the urea and homocysteine quantifications, we followed their corresponding assay procedures mentioned in the user manuals of their assay kits,Citation19,Citation20 except that the amount of sample used was 100 μL rather than the recommended amount. An excellent linear relationship (r2 > 0.95) between urea concentration and its relative absorbance was found, and the relationship between homocysteine concentration and its relative absorbance was also found to be linearly excellent (r2 > 0.9). This allowed the urea and homocysteine concentrations to be calculated simply by linear regression.
Statistical analysis
One-way analysis of variance was used to determine whether there were significant differences between the dc and SBdc for urea and homocysteine extraction. Post hoc comparisons were made with the least significant difference (LSD) test. All statistical analyses were carried out using SPSS 10.0 software (SPSS, Chicago, IL), with the level of statistical significance set at P < 0.05.
Results and discussion
An in vitro model was used in this study because of its simplicity. We were able to create a normal (ie, healthy) model, a cardiovascular disease model, and a kidney disease model, by just simply adjusting the solution stored inside the lower chamber of the diffusion cell. For example, the kidney disease model could be created by preparing the lower chamber solution with a high urea concentration, ie, 20 mM urea. On the other hand, it is relatively difficult and expensive to create an in vivo model. Hence, an in vitro model was used in this study. We simply used the normal model (5 mM urea and 15 μM homocysteine) for this investigation in order to find out whether homocysteine was extractable, as well as whether homocysteine and urea could be extracted simultaneously. In a future study, cardiovascular disease and kidney disease models will be used.
Skin consists of three layers, ie, the epidermis, dermis, and subcutis. The epidermis (about 100 μm in thickness) is the topmost layer of the skin, forming a barrier for many substances going in and out. The in vitro model used in this study also had these three layers. The diffusion cell used in this study had a lower chamber, and its function was to simulate the dermis and subcutis. For the epidermis, a porcine ear skin was used, and its thickness (250 μm) was the same as the thickness reported in diffusion studies done by other researchers.Citation17,Citation18
There are three mechanisms for reverse iontophoresis, ie, diffusion (almost negligible), electromigration, and electro-osmosis. The major mechanisms for transport of ions and molecules are electromigration of charged species to the electrode of opposite polarity, electro-osmosis of neutral molecules to the cathode or anode, or a combination of these two processes.
Homocysteine, an uncharged molecule with a molecular weight of about 135 Da, is a hydrophilic molecule (partition coefficient −2.2). Because of its small size and hydrophilic properties, homocysteine is expected to be transdermally extractable by electro-osmosis. Urea is a negatively charged molecule and its molecular weight is about 60.06 Da. As a consequence of its small size and negative charge, electromigration is the dominant physical factor for urea extraction.
As expected, reverse iontophoresis facilitated the transdermal extraction of homocysteine and urea (see ). shows that there was a significant difference between the dc and the four SBdc on extraction of homocysteine (one-way analysis of variance, P < 0.001). It was found that there was a significantly higher homocysteine extraction for the dc and the four SBdc than for the control sample which utilized diffusion-only extraction (LSD test, P < 0.001 in most cases and P < 0.05 in the remaining cases). This suggests that the use of physical force, like reverse iontophoresis, can promote more homocysteine extraction than diffusion alone. Because homocysteine is an uncharged molecule, it theoretically should be extracted to the electrode chamber of the diffusion cell with the negative polarity (ie, the cathode). As shown in , although slightly higher homocysteine extraction was observed at the cathode than at the anode, the difference was not significant. This might be because prolonged dc passage can cause skin polarization, which results in the reduction of electro-osmotic flow. Homocysteine extractions at the dc-cathode and the dc-anode were found to be significantly lower than that at the four SBdc (LSD test, P < 0.001 in most cases and P < 0.05 in the remaining cases). A possible explanation is that the use of dc for reverse iontophoresis causes skin polarization. Polarization effects turn the skin into what is effectively a charged capacitor, and this reduces the flow of current and charged species during reverse iontophoresis. However, the use of SBdc has been suggested as a means to overcome skin polarization.Citation14,Citation15 Therefore, SBdc promoted more homocysteine extraction than dc. Also, homocysteine extraction using 60s PDSBdc was found to be significantly higher than that with protocols utilizing 15s PDSBdc (LSD test, P < 0.001) and 30s PDSBdc (LSD test, P < 0.05) but there was no significant difference when using 180s PDSBdc (LSD test, P = 0.838). This result suggests that extraction of homocysteine by SBdc is phase duration-dependent. Because a plateau was observed at 60s PDSBdc and 180s PDSBdc for homocysteine extraction, the optimum mode for homocysteine extraction is therefore the 60s SBdc. Moreover, it was found that a longer extraction time resulted in relatively more homocysteine extraction. However, a three-fold increase in extraction time did not result in a three-fold increase in homocysteine extraction (see ). More importantly, the homocysteine extraction flux (see ) and even effective homocysteine extraction (see ) decreased with increased extraction time. This indicates the presence of a homocysteine reservoir in the skin.
Table 1 Effect of the direct current and the symmetrical biphasic direct currents on effective urea and homocysteine extraction (n ≥ 3)
Figure 2 The transdermal extraction of A) homocysteine and B) urea (mean ± standard deviation; n ≥ 3 for each bar) by reverse iontophoresis. The extraction period was 12 minutes and 36 minutes. The current density of the extraction was 0.3 mA/cm2; 15s, 30s, 60s, and 180s PDSBdc corresponds to the symmetrical biphasic direct current with the phase duration of 15 seconds, 30 seconds, 60 seconds, and 180 seconds, respectively. The dc-cathode and dc-anode correspond to a cathode of direct current and an anode of direct current, respectively.
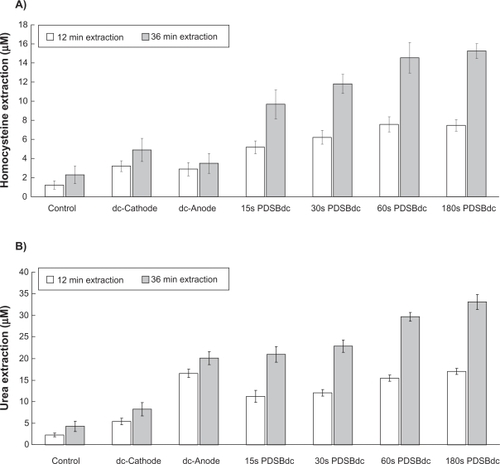
Figure 3 A) Homocysteine and B) urea extraction fluxes as a function of time (mean ± standard deviation; n ≥ 3). The current density of the extraction was 0.3 mA/cm2; 15s, 30s, 60s, and 180s. PDSBdc corresponds to the symmetrical biphasic direct current with the phase duration of 15 seconds, 30 seconds, 60 seconds, and 180 seconds, respectively. The dc-cathode and dc-anode corresponds to a cathode of direct current and an anode of direct current, respectively.
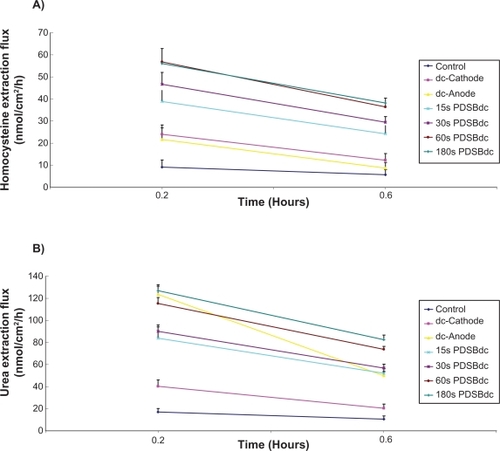
On the other hand, as shown in , a significant difference was found between dc and the four SBdc for the extraction of urea (one-way analysis of variance, P < 0.001). Again, the dc and the four SBdc were found to promote more urea extraction than diffusion alone (LSD test, P < 0.001 in most cases and P < 0.05 in the remaining cases). Because urea carries a negative charge, urea should theoretically be extracted to the electrode chamber of the diffusion cell with the positive polarity. As shown in , our results agreed with this theoretical phenomenon that the dc-anode facilitates significantly (P < 0.001) more urea extraction than the dc-cathode. Urea extraction using the dc-anode was found to be significantly higher than that of protocols utilizing 15s PDSBdc and 30s PDSBdc (LSD test, P < 0.001 in all cases) but there was no significant difference using 60s PDSBdc (LSD test, P = 0.139) and 180s PDSBdc (LSD test, P = 0.540). However, as shown in , it could be argued that because the dc and SBdc have different effective extraction times (defined as the total number of hours for the passage of current at the same polarity during the whole experimental time), a standardization technique should be employed to investigate the rate of transdermal extraction of urea. Therefore, the extraction rate for urea was recalculated in nmol/hour, where time was set as the actual time of the applied current at the electrode chamber collecting the molecule. This is based on the assumption that, at any time in the cycle, the anode dominates the collection of urea. As shown in , calculating the effective urea extraction amount in this format shows that urea extraction using the dc-anode was significantly lower than when using SBdc (LSD test, P < 0.001 in all cases). On the other hand, urea extraction using 60s PDSBdc was found to be significantly higher than when using 15s PDSBdc and 30s PDSBdc (LSD test, P < 0.001 in all cases) but lower than that when using 180s PDSBdc (LSD test, P < 0.05). This result suggests that extraction of urea by SBdc is also phase duration-dependent. Although 15s, 30s, 60s, and 180s PDSBdc showed the same effective urea extraction time, it was found that a long PDSBdc results in higher urea extraction. Because skin has a thickness dimension, urea takes some time to pass through the skin. For a short PDSBdc, some urea molecules may not have enough time to pass through and may stay inside the skin. These urea molecules will not move forward into the collection chamber, and may even move backwards to the lower chamber of the diffusion cell when the phase of the SBdc is reversed. This might explain the phase duration-dependent phenomenon. Because urea extraction using 60s PDSBdc was slightly lower than that using 180s PDSBdc (LSD test, P = 0.045), the optimum mode for urea extraction is 60s SBdc in order to achieve simultaneous extraction of urea and homocysteine. On the other hand, it was found that a longer extraction time caused comparatively more urea extraction, although a threefold increase in extraction time could not cause a three-fold increase in urea extraction (see ). Moreover, the urea extraction flux (see ) and even the effective urea extraction (see ) decreased with increased extraction time. This also indicates the presence of a urea reservoir in the skin. Conversely, as shown in , the result of dc-anode urea extraction flux in 36 minutes (about 0.05 μmole/cm2/hour ≈ 0.01 μmole/hour) was found to be in agreement with the findings reported by Wascotte et al in 2007.Citation7 They found that the urea extraction flux in 30 minutes was about 0.015 μmole/hour. However, our finding of PDSBdc urea extraction flux in 36 minutes (see ) was higher than that of Wascotte et al. This means that PDSBdc resulted in a higher urea extraction flux as compared with dc alone.
Based on our findings, reverse iontophoresis potentially provides a promising technique for simultaneous, noninvasive, and transdermal extraction of urea and homocysteine. However, it does not mean that this is applicable in humans. Therefore, ethic approval has been applied for to do further research. Once the human study is approved, experiments will be conducted to test our hypothesis that homocysteine is transdermally extractable, and that homocysteine and urea can be simultaneously extracted in humans. If our hypothesis is correct, a homocysteine-urea Glucowatch may be able to be developed in the future. This could help potential users to have a screening test inhouse. Of course, a homocysteine-urea watch may face the same reproducibility problems as those found with the Glucowatch. Therefore, intensive research will be necessary to overcome this reproducibility problem.
Conclusion
A novel method for noninvasive transdermal extraction of homocysteine by reverse iontophoresis has been demonstrated. Reverse iontophoresis facilitates transdermal extraction of homocysteine and urea at the same time. The optimum mode for simultaneous homocysteine and urea extraction appears to be SBdc with a phase duration of 60 seconds. Once this hypothesist is proven in humans in the future, this new method for urea and homocysteine monitoring would be beneficial to patients, such as those with kidney disease, or obese individuals who are at high risk of developing coronary artery disease.
Acknowledgements
This work was supported by a grant (98-2221-E-260-002-) from the National Science Council, Taiwan, Republic of China. It was also partially supported by a grant (99T8888-6) from the National Chi Nan University, Taiwan, Republic of China, and another grant (98-2221-E-260-024-MY3) from the National Science Council, Taiwan, Republic of China.
Disclosure
The authors report no conflicts of interest in this work.
References
- WHOCardiovascular Disease Prevention and Control2003 Available at: http://www.who.int. Accessed November 17, 2010.
- GoereeRManalichJGrootendorstPBeecroftMLChurchillDNCost analysis of dialysis treatments for end-stage renal diseasesClin Invest Med1996184554648714789
- RaineAEGMargreiterRBrunnerFPReport on management of renal failure in Europe, XXII, 1991Nephrol Dial Transplant199271Suppl 27351338121
- Department of Health, Executive YuanHealth statistical reports, 2008 Available at: http://www.doh.gov.tw. Accessed November 17, 2010.
- StampferMJMalinowMRWillettWCA prospective study of plasma homocyst(e)ine and risk of myocardial infarction in US physiciansJAMA19922688778811640615
- ManNKTouamMJungersPCauses et consequences de l’uremie chronique, indications de la dialyse de suppleanceL’Hemodialyse de SuppleanceFlammarion, ParisMedecine-Sciences2003
- WascotteVRozetESalvaterraANon-invasive diagnosis and monitoring of chronic kidney disease by reverse iontophoresis of urea in vivoEur J Pharm Biopharm2008691077108218406585
- WascotteVDelgado-CharroMBRozetEMonitoring of urea and potassium by reverse iontophoresis in vitroPharm Res2007241131113717380270
- DegimITIlbasmisSDundarozRReverse iontophoresis: A non-invasive technique for measuring blood urea levelPediatr Nephrol2003181032103712898373
- MerinoVKaliaYNGuyRHTransdermal therapy and diagnosis by iontophoresisTrends Biotechnol1997152882909263476
- PitzerKRDesaiSDunnTDetection of hypoglycaemia with the GlucoWatch biographerDiabetes Care20012488188511347748
- TierneyMJTamadaJAPottsROClinical evaluation of the GlucoWatch® biographer: A continual, non-invasive glucose monitor for patients with diabetesBiosens Bioelectron20011662162911679237
- ChingTSConnollyPSimultaneous transdermal extraction of glucose and lactate from human subjects by reverse iontophoresisInt J Nanomedicine2008321122318686780
- HowardJPDrakeTRKelloggDLEffects of alternating current iontophoresis on drug deliveryArch Phys Med Rehabil1995764634667741619
- TomohiraYMachidaYOnishiHIontophoretic transdermal absorption of insulin and calcitonin in rats with newly-devised switching technique and addition of ureaInt J Pharm1997155231239
- MannJFGersteinHCPogueJRenal insufficiency as a predictor of cardiovascular outcomes and the impact of ramipril: The HOPE randomized trialAnn Intern Med200113462963611304102
- ChenJHammellDCSpryMIn vitro skin diffusion study of pure forskolin versus a forskolin-containing Plectranthus barbatus root extractJ Nat Prod20097276977119281221
- BaynesREBrooksJDMumtazMEffect of chemical interactions in pentachlorophenol mixtures on skin and membrane transportToxicol Sci20026929530512377978
- Homocysteine enzymatic assay kit Available at: http://www.ibl-america.com/pdf/newIBLelisa/IB4610.1.pdf. Accessed November 17, 2010.
- Urea assay kit Available at: http://www.biovision.com/manuals/K375-100.pdf?osCsid=j6kit0eqdved7ebtnsjv5g0010. Accessed November 17, 2010.