Abstract
Staphylococcus aureus (S. aureus) is an important zoonotic bacteria and hazardous for the health of human beings and livestock globally. The characteristics like biofilm forming, facultative intracellular survival, and growing resistance of S. aureus pose a great challenge to its use in therapy. Nanoparticles are considered as a promising way to overcome the infections’ therapeutic problems caused by S. aureus. In this paper, the present progress and challenges of nanoparticles in the treatment of S. aureus infection are focused on stepwise. First, the survival and infection mechanism of S. aureus are analyzed. Second, the treatment challenges posed by S. aureus are provided, which is followed by the third step including the advantages of nanoparticles in improving the penetration and accumulation ability of their payload antibiotics into cell, inhibiting S. aureus biofilm formation, and enhancing the antibacterial activity against resistant isolates. Finally, the challenges and future perspective of nanoparticles for S. aureus infection therapy are introduced. This review will help the readers to realize that the nanosystems can effectively fight against the S. aureus infection by inhibiting biofilm formation, enhancing intracellular delivery, and improving activity against methicillin-resistant S. aureus and small colony variant phenotypes as well as aim to help researchers looking for more efficient nano-systems to combat the S. aureus infections.
Introduction
The infections caused by Staphylococcus aureus (S. aureus) seriously threaten human health and cause huge economic losses in farm. It is calculated that ~30% healthy people colonized by S. aureus do not exhibit any symptoms.Citation1–Citation4 S. aureus can cause many diseases such as skin infections, abscesses, impetigo, necrotizing pneumonia, septicemia, catheter-induced endocarditis, atherosclerosis, and osteomyelitis.Citation5–Citation7 Especially, the opportunistic infections in hospitals are extremely serious. It is reported that around 20% of surgical-site infections are caused by S. aureus.Citation8 The highly virulent methicillin-resistant S. aureus (MRSA) is a worrying public health threat in countries all across the world, and different popular strains have been isolated in communities and hospitals.Citation5 It was reported that the treatment cost of MRSA infections is $3,700 and more than those of methicillin-sensitive S. aureus infections. Moreover, the death rate is about threefold that of the latter.Citation9,Citation10
In the livestock breeding, the bovine mastitis caused by S. aureus has induced a number of economic losses including the decrease of milk production and quality, increase of culling and death rates, and so on.Citation11,Citation12 Staphylococcal subclinical mastitis accounts for 30% bovine mastitis.Citation13 It was reported that the S. aureus infections lead to a loss of about 380 tons of milk every year in the world.Citation14 The presence of S. aureus in raw milk is also a public health problem throughout the food chain. The presence of S. aureus in cells can establish reservoirs from which reinfection will occur,Citation15,Citation16 and then result in long-term and repeated infection.Citation17,Citation18 The intracellular survival strategies of S. aureus are associated with the subclinical and relapsing infection of bovine mastitis.
The facultative intracellular parasitism and biofilm of S. aureus protect them from host immune responses and the effect of antibiotics,Citation19 and thus present huge treatment challenges for the global medical community. In addition, the increasing resistance of S. aureus also leads to the treatment difficulty. Over decades, the nanoparticle carriers are reported to be one of the potential measures for improving their payload drug permeability across cell membrane, enhancing intracellular accumulation, increasing the antibacterial activity of antimicrobial agents against the resistant strains, offering multiple bactericidal mechanisms, and inhibiting the biofilm formation of S. aureus. We searched PubMed, Scopus, Web of Science, and Cochrane Central register of related publications about the application of nanoparticles in the treatment of intracellular infection using relevant keywords (nano, intracellular infections, intracellular delivery, S. aureus strategies, nanogel). About 3,625 records and 513 of closely related papers were screened for suitable studies. In this paper, the progress, challenges, and perspectives of nanomedicines for S. aureus infections are summarized according to the related publications to explore more efficient nanosystems to help human beings win the war against the S. aureus in the future.
Invasion strategies of S. aureus
S. aureus is a typical facultative intracellular bacterium. At the beginning of invasion, it first adheres to the surface of the body such as the skin and nasal cavity with the help of its secreted factors.Citation20 The process of host adhesion is the key step for the pathogenesis of S. aureus.Citation21,Citation22 S. aureus can secrete many kinds of factors () to resist the immune response of hosts and thus achieve successful colonization.Citation23,Citation24 Among these, fibronectin-binding protein A (Fnbp A), Fnbp B, and wall teichoic acid promote the colonization. In these processes, S. aureus secretes some factors to assist in resistance to the host immune defenses. For example, iron-regulated surface determinant A (Isd A) can enhance bacterial cellular hydrophobicity and thus help S. aureus resist bactericidal fatty acids.
Table 1 The function of various factors of Staphylococcus aureus
After host adhesion and colonization with the help of various factors, S. aureus invades cell and starts living within it. The bacteria can enter the cell and reside in special compartments using some smart mechanisms, leading to huge difficulties for their cleaning by host immune system and antimicrobials. The survival and proliferation of S. aureus within cells were via preventing combination of phagosome and lysosome, subversion autophagy, and others.Citation25 The toxin factors of S. aureus play a pivotal role () in the process of penetration into cell membrane and intracellular survival.Citation26 The β-toxin and δ-toxin are reported to relate to the penetration across cell membrane. β-toxin can hydrolyze sphingomyelin, which constitutes the membrane into hydrophilic phosphorylcholine and hydrophobic ceramide.Citation27 When the sphingomyelin is hydrolyzed by β-toxin, δ-toxin accumulates in the hydrophobic ceramide domains and the bacteria eventually permeabilize the cytomembrane ().Citation28 It was reported that α-toxin, a pore-forming toxin, can penetrate host cell membranes, and subsequently cause osmotic swelling, rupture, lysis, and cell death.Citation29,Citation30
Table 2 The functions of toxin factors of Staphylococcus aureus for intracellular survival
Figure 1 The schematic diagram of Staphylococcus aureus permeabilization into cell membrane via β-toxin and δ-toxin.
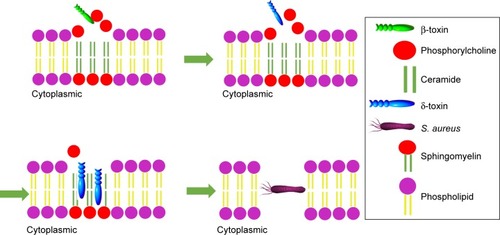
After endocytosis, some bacteria can inhibit the fusion of phagosome and lysosome or escape from phagosome by certain factors and mechanisms (). Grosz et al demonstrated that S. aureus 6850, MW2, and LAC can escape from the phagosomes of phagocytes by mediation of phenol-soluble modulin α (PSMα).Citation31 After cellular invasion, an intracellular niche served as a reservoir for the survival, and chronic carriage of S. aureus might be formed for the chronic and repeated infections.Citation32
Figure 2 The mechanism of Staphylococcus aureus infection cells.
Abbreviation: SCV, small colony variant.
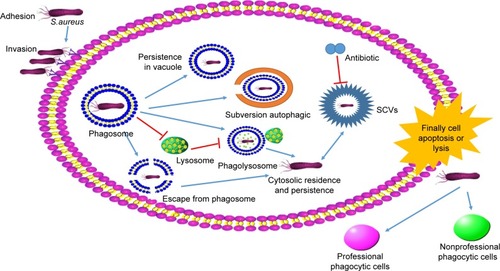
After successful infection, the small colony variants (SCVs) of S. aureus will be formulated.Citation33 There is a highly dynamic population between SCVs and normal phenotype. Tuchscherr et al reported that 25% S. aureus will transform to SCVs without any selective pressure.Citation35 Under the selective environmental stress, S. aureus will easily switch to SCVs. It can be induced by triclosan, cold stress, and high hydrostaticity. It is reported that the SCVs will arise when S. aureus is treated by antimicrobial agents in vitro culture.Citation34 The SCVs are related to the resistance of S. aureus, reinfection, and chronic infections.Citation36–Citation38 The SCVs are difficult to be detected by host innate immune system via decreasing of toxin and proinflammatory factor secretion when compared to normal phenotype.Citation39 It was reported that the intracellular infection of WCH-SK2 wild-type promoted the expression of much more factors (TLR2, tissue remodeling factors, and proinflammatory cytokines) than WCH-SK2SCV (only upregulated expression of TLR2).Citation40 In addition, the metabolism level and growth of SCVs will be slower than the normal phenotype due to the obstacle of tricarboxylic acid metabolism and energy production,Citation41–Citation44 because of dependence to hemin, menadione and thymidine.Citation1,Citation45
Treatment challenges of S. aureus infections
As mentioned earlier, S. aureus is able to escape the cleaning by the innate immune system and antimicrobial drugs with the help of various factors. The α-toxin, β-toxin, δ-toxin, PSMα, and others contribute to multiplication and spread of the intracellular S. aureus and maintain their intracellular lifestyle. Some factors contribute to form a biofilm, which is propitious to escape the stress of antimicrobial agents and immunity. In addition, the SCV phenotype is one of the difficulties that we have met in the treatment of S. aureus infections due to their low metabolism level and the virulence factors are expressed less than normal phenotype. A large number of antibiotics are devoid of the ability of cell membrane and bacterial biofilm penetration, and intracellular short time retention, which results in inadequate intracellular distribution and low intracellular concentration. These make the S. aureus infection treatment highly challenging.
S. aureus biofilms
As we are well aware, the biofilm formation is a process of quorum sensing (QS). The small-molecule signals named autoinducers (AIs) will be secreted and will accumulate in the extracellular media when the bacteria multiply. When enough bacteria are reached, the AIs will enable single bacterium to sense other bacteria in their surroundings and could form biofilms.Citation46 The formed biofilms exhibit higher virulence and resistance compared to planktonic S. aureus. Oyama et al reported that the thick biofilms of S. aureus showed higher virulence to mice livers than the thin biofilms. Moreover, the biofilms contribute to the intracellular survival of S. aureus and thus lead to chronic infections.Citation47 It is well known that most drugs have poor permeability across the biofilms and exhibit poor activity against biofilm-forming S. aureus than that of planktonic S. aureus. Currently, S. aureus biofilms are a serious conundrum and no effective treatment methods are available so far.
Intracellular survival
One of the key challenges of intracellular S. aureus infections treatment is how to deliver enough antibacterial drugs to the site where intracellular bacteria are located.Citation48 A multitude of antibiotics have low cell membrane penetration (β-lactams and aminoglycosides),Citation49 intracellular unabiding retention (fluoroquinolones and macrolides), inadequate intracellular distribution, and low intracellular concentration ().Citation50,Citation51 Therefore, intracellular S. aureus infection treatment is a great challenge for the global medical community. As mentioned earlier, S. aureus can easily switch to SCV phenotype and thus lower the metabolism level. Many antibiotics, especially the breeding-stage sterilization drugs (penicillin, cephalosporin), are invalid to them. As we know, membrane potential will be rapidly lowered when the flow of electrons in the electron transport chain is impaired. For aminoglycosides, its uptake by bacterial cells relies on the membrane potential. SCVs will occur when the electron transport chain breaks off, thereby reducing the membrane potential and hence limiting the uptake of aminoglycosides (eg, gentamicin).Citation52 Moreover, the process of wild phenotype switching to SCV phenotype is often linked with the reduction of the number of ATP,Citation53 which is necessary for drug molecules to enter into cytoplasm by active transport, macropinocytosis, or phagocytosis.
Table 3 The challenges of conventional antimicrobial agents against Staphylococcus aureus infections
Resistance
The increasing resistance is another obstacle in the treatment of S. aureus infection. Their multidrug resistance gives them the ability to escape the pharmacologic action of antibiotics. During the earlier periods, what we knew more about the resistant strains of S. aureus was resistance to β-lactams, but recent reports have indicated that S. aureus has already been resistant to daptomycinCitation54 and glycopeptide antibiotics (teicoplanin and vancomycin), which have been used to treat MRSA especially in severe infections.Citation55
There are several main resistance mechanisms for S. aureus. The development of resistance genes is a key resistance mechanism. The methicillin or cephalosporin resistance was conferred by the mecA gene and its homologous genes mec B and mec C.Citation56 The mecA gene encodes PBP2a or PBP2′, a kind of specific penicillin-binding protein. These proteins will degrade the β-lactam ring, which confers the activity of penicillin, cephalosporin, and methicillin. Besides, mecA can spread by the chromosome cassette mec genetic element of S. aureus.Citation57 Another resistance strategy of S. aureus is the efflux pump, which is able to actively effuse antimicrobial agents out of bacteria. The S. aureus biofilm also links with resistance.Citation58 It was reported that the resistance of S. aureus in the biofilm state was enhanced because of the reduced permeability of drugs.Citation59 Currently, the effective strategic measures involving alternative therapeutics that can reduce the resistance of S. aureus are imperative.
Enhanced activity of antibiotics against S. aureus by nanoparticles
As discussed earlier, many antibiotics are ineffective in the treatment of the infections caused by S. aureus due to the intelligent survival strategies and self-protection measures of S. aureus. The nanomedicines have been an emerging therapeutic approach to conquer the obstacles of treatment of S. aureus infections with their ability of inhibition of the formation of biofilm,Citation60 penetration of cell and biofilm membrane, enhanced intracellular retention,Citation61 and improved antibacterial activity of the loaded antimicrobial agents. Nanoparticles can passively accumulate in certain organs and infection site because of their special characteristics, such as nanosize, surface charge, and large specific surface area. The modified nanoparticles could further enhance the transmembrane performance of their payload drug by actively realizing the receptors of host cells and bacterial cells. Currently, many antimicrobial agents are incorporated into or conjugated with nanocarriers to enhance the pharmacologic activities against sensitive and resistant S. aureus in the SCVs and normal phenotype states and action times, and to reduce the side effects of the drug (). Therefore, nanoparticle drug delivery systems proved an ideal weapon to overcome the challenges of S. aureus infection that we faced.
Table 4 The examples of improving antibacterial effect against Staphylococcus aureus infections by nanoparticle delivery systems
Inhibition of biofilm formation
Because of the high permeability, nanoparticles can penetrate the thick biofilms. Bastari et al demonstrated that the nafcillin sodium and levofloxacin-loaded poly (lactide-co-glycolide) (PLGA) nanoparticles coated with calcium phosphate are able to inhibit the formation of S. aureus biofilm for 4 weeks.Citation62 Thomas et al demonstrated that ciprofloxacin-loaded PLGA nanoparticles were more effective against S. aureus biofilms than ciprofloxacin solution.Citation63 As reported earlier, tetracycline-loaded chitosan nanoparticles were more effective than free tetracycline in killing intracellular S. aureus.Citation64 The bacillus natto antimicrobial lipopeptide carboxymethyl–loaded chitosan nanoparticles exhibited a good inhibition and scavenging effect on the S. aureus biofilm formation and the surface-attached bacteria growth.Citation65
It is well known that glycocalyx, the main component of bacterial biofilm, is typically anionic.Citation66 Some cationic nanoparticles loaded with antimicrobial agents provide a new promising way to treat the infections of S. aureus that formed biofilm. It was reported that cationic liposomal ceftazidime could remarkably inhibit the biofilm formation of S. aureus compared with the ceftazidime solution.Citation67 The cell membrane of S. aureus will be disrupted when the positively charged ions of particle bind to negatively charged groups of the bacterial membrane. This process will create pores on the membrane and result in flowing out of cytoplasmic contents from the cell and dissipating the gradient of H+ across the cell membrane, which may lead to cell death.Citation68,Citation69
Some metal nanoparticles have been developed to effectively restrain the biofilm formation of S. aureus. For example, surface-adaptive gold nanoparticles exhibited enhanced photothermal ablation on MRSA biofilm under near-infrared light irradiation without injury to the healthy tissues.Citation70 Nanosized ZnO strengthened the antibacterial activity of their loaded antimicrobial agents, decreased the biofilm formation, and overcame attachment of MRSA on medical instruments in the implant-related infections.Citation71 The metal ions can lead to bacterial cell membrane disruption and then internalization into the bacterial cytosol. The reactive oxygen species form after internalization and then lead to DNA damage and cell death. It is also reported that the nanoparticles can effectively inhibit the biofilm formation via inhibiting the QS-regulated gene expression.Citation72
Enhanced intracellular delivery
The therapeutic effect of antimicrobial agents against intracellular S. aureus is dependent on the persistent time in the infected cell above the effective therapeutic levels. The drug concentration within the cell is decided by their ability of penetration into cell membrane and intracellular accumulation performance. As we all know, the nanoparticles can improve the permeability as well as accumulation of their payload drug within cells. Due to the direct effect between the particles and S. aureus in contact with them and the diffusion of the released drugs to S. aureus, the increased cellular uptake and subsequent controlled release of the nanoparticle entrapped/adsorbed antibiotics can effectively enhance their antibacterial effects, which make it more effective to treat intracellular infection. For instance, PLGA nanoparticles can increase the intracellular gentamicin and improve subcellular distribution, thus demonstrating stronger antibacterial effect against S. aureus.Citation73 The polymeric nanoparticles with ionic core and specific hydrophobic/hydrophilicity chemistry of the shell can also produce promising action against bacteria via interaction with cell membrane by hydrophobic segment of shell and stronger electrostatic interaction with the opposite surface charge of the core. It was reported that the modified nanoparticles with specific ligands of macrophage can enhance the phagocytosis efficiency for improving the intracellular concentration of antimicrobial agents.Citation74 Chakraborty et al discovered that vancomycin chitosan folic acid nanoparticles showed more effective performance across the epithelial and bacterial cell surfaces and stronger anti-S. aureus effect compared to the chitosan nanoparticles.Citation75
Liposomes are also viewed as a prospective carrier for intracel lular delivery of antimicrobial agents due to their phospho lipid bilayer structure being just like a cell membrane, meaning the phospho lipid bilayer structure can easily bind other groups so that it can be designed responsively to secreted bacterial toxins. Gupta et al demonstrated that levofloxacin liposomes exhibited prolonged, improved antibiofilm and antimicrobial efficacy in treating S. aureus infection.Citation76 Recently, it was confirmed that chloramphenicol-loaded deoxycholic acid liposomes can increase antibacterial effect on keratinocyte-infected MRSA and that the deformable liposomes hold excellent biocompatibility.Citation77 It was reported that the compound of vancomycin-loaded liposome surface bounded with chitosan-modified gold nanoparticles has the ability of responding to bacterial toxins.Citation78 The liposomes were also proved to enhance the intracellular gentamicin and antibacterial activity against S. aureus.Citation79 Ahani et al also indicated that polyhexamethylene biguanide chloride cationic liposome enables delivery of high concentrations of the antibacterial agent into the infectious cell and lower cytotoxicity.Citation80 Bas et al reported that the intracellular concentration of liposomal ofloxacin can reach up to 2.6-fold that of free ofloxacin.Citation81 A study reported that enrofloxacin load liposomes can inhibit the S. aureus in neutrophils for 60 minutes.Citation82 It was reported that the chitosan-decorated liposome containing alpha-lipoic acid and coenzyme Q10 also showed strong bactericidal effect with S. aureus. This new measure with multiple antimicrobial mechanisms will be a potential approach for reducing the resistance development of S. aureus.Citation83 In addition, aimed at the higher temperature of the infected site than the healthy tissue, a kind of antibiotic-loaded thermally sensitive liposome that can release completely at ≥39°C was designed, and it showed significant ability of killing S. aureus at 42°C than at 37°C.Citation84
Solid lipid nanoparticles (SLNs) might be another promising drug delivery system with the distinct advantages of biodegradability, good biocompatibility, and stability. Our previous work also showed that enrofloxacin-loaded docosanoic acid SLNs could effectively increase the accumulation and storage time of enrofloxacin within the cell.Citation85 The cellular uptake and accumulation of payload enrofloxacin are influenced by the zeta potential and diameter of the nanoparticles.
Some inorganic nanoparticles also showed huge potential for the S. aureus infection treatment. For example, β-tricalcium phosphate nanoparticles have made great achievements in osteomyelitis of S. aureus because of the stimulation bone regeneration function of β-tricalcium phosphate.Citation86,Citation87 The phagocytosed number of ciprofloxacin-loaded niosomes was much more than free ciprofloxacin and showed higher antimicrobial activity against intracellular S. aureus.Citation88
The antibacterial activity of nanoparticles is determined by its stability to the infected cells and the arrival in the target subcellular site in a predetermined way. The nanoparticles hold different transport routes into cells including phagocytosis (zipper-like and trigger-like) and nonphagocytosisCitation89 (). The different transport routes could influence the cellular uptake and intracellular distribution, thus influencing the therapy effects. Most uptake ways, that is, clathrin-mediated endocytosis, trigger-like phagocytosis, and macropinocytosis, mainly gather in the late endosomes and/or finally lysosomes to form an endolysosome (phagolysosome), and thus the internalized nanoparticles are often stored at acidic environments of endosomes and/or lysosomes and/or endolysosome (phagolysosome).Citation90 This transcellular way will be used to fight against S. aureus accumulated in phagosomes/phagolysosomes (endosomes/endolysosomes) and help to increase the antimicrobial effect of diffusible drug against cytoplasmic S. aureus or vesicles containing S. aureus. The endosomotropic/lysosomotropic trafficking might be highly effective against S. aureus because the main intracellular parasitic sites of S. aureus were phagosome and cytoplasm.Citation28 Besides, lysosomotropic or/and endosomotropic transports (ie, CvME, zipper-like phagocytosis) also might be an alternative way for intracellular antimicrobial agent delivery due to the ability to bypass lysosomes. Therefore, the non-lysosomotropic transports may be helpful for intracellular delivery of antibiotics that are hypersensitive to lysosomal enzymes and to kill the pathogen invaded in the similar pathways as bacteria.Citation91,Citation92
The transmembrane pathways and mechanism of nanoparticles depended on the size, zeta potential, surface hydrophilicity, and shape of nanoparticles. The clathrin-mediated endocytosis mainly aimed at the nanoparticles with size range from 100 to 200 nm. Therefore, 100–200 nm nanoparticles may have better intracellular colocalization with S. aureus by the clathrin-mediated endocytosis. For example, Sémiramoth et al proved that penicillin G self-assembled nanoparticles with a size of 140±10 nm displayed stronger penetration into cell through clathrin-dependent than free penicillin G and showed enhanced antibacterial activity against intracellular S. aureus.Citation93 In addition, the charge is also an important element that will influence the uptake of nanoparticle. The nanoparticles with either negative or positive charge appear to have more efficient endocytosis than the neutral nanoparticles.Citation94 The constituent of nanoparticle plays a key effect on uptake ways in that it determines the surface properties of nanoparticles. Generally, the hydrophilicity/hydrophobicity will influence the opsonization and phagocytosis, and thus determine the fate of exogenous nanoparticles in vivo. The surface hydrophobicity seems to be an important factor for enhanced uptake of nanoparticles. Couvreur et al showed that PEGylation of nanoparticles decreases the macrophage uptake.Citation95 Recently, the controlling phagocytosis via shape change of nanoparticle is more increasingly focused on. Beningo et al declared that rigid polyacrylamide nanoparticles were more easily uptaken than the soft ones because they were able to stimulate the assembly of actin filaments, which are required for the formation and closure of phagosomes.Citation96
Improved activity against MRSA
The resistance is one of the major barriers for fighting against the S. aureus infection, especially for MRSA. Facing the situation that the evolution of pathogens and resistance production of S. aureus are faster than the discovery and development of new drugs, the active recovery of existing antibiotics using the pharmaceutical technologies will be a potential strategy. Recently, nanomedicines were viewed as a promising measure to overcome the problem of MRSA.Citation97 It is reported that vancomycin HCl-SLNs displayed a more effective and longer effect on the resistant and sensitive S. aureus than vancomycin HCL.Citation98 Liposomes’ codelivery of clarithromycin and daptomycin with a mass ratio of 1:32 demonstrated an enhanced anti-MRSA activity and increased the survival of the infected host cells compared to the liposomal daptomycin and liposomal clarithromycin, respectively.Citation99 Antibiotics-loaded nanoparticles might hold high permeability to MRSA because of their small particle size. It is well known that nanoparticles have positive or negative surface charge itself or by decoration. The surface charge is convenient for the adsorbing of nanoparticles on the surface of MRSA and then contributed to express high antibacterial activity of antimicrobial agents. Besides the antimicrobial agents, the carrier materials (metal ion, lipids, and hydrogels) also have the antibacterial activity. The multiple bactericidal mechanisms (metal ion release, oxidative stress induction, DNA or ribonucleic acid damaging, and cell membrane disruption) require multiple gene mutations simultaneously, and therefore the resistance of bacteria is avoided or produced difficultly.
Some researchers focus on the modification of nanoparticles to further enhance the effects of antimicrobial agents against MRSA. For example, Cholsuc-VQWRIRVAVIRK-NH2 (DP7-C)-modified liposomes payload azithromycin showed higher anti-MRSA effect in mouse model compared to liposomal and free azithromycin via the upregulation of anti-inflammatory cytokines and chemokines.Citation100 It is more meaningful for pH-sensitive nanoparticles to combat the resistanceCitation101 because S. aureus can produce acidity at infection sites.Citation102 Some nanoparticle materials and modification of nanoparticles can make the nanoparticles pH sensitive. The nanoparticles will release drugs quickly in acid environment of the infection site of MRSA, thus achieving strong activity.Citation103
Metal nanoparticles are being tried to overcome the resistance of MRSA based on the different antibacterial activities of metals and the unique nature of nanoparticles. For example, Aurore et al found that nano-silver displayed excellent antibacterial activity against intracellular MRSA in osteoclasts at the level of nontoxic concentration and thus showed potential measures for bone infection treatment.Citation104 There are some controversies that the metal ion might enhance the resistance of bacteria. In the future, it will be possible for the combination of existing nanoparticles and modified technology to further improve the activity of antibiotics against resistant S. aureus and make them to be widely used in clinics.
Improved activity against SCV phenotypes
The typical characteristics of SCV phenotype are the lower metabolism level and growth rate than the normal phenotype, which makes the S. aureus difficult to detect by the immune system and difficult to destroy by antimicrobial agents. Some researchers are trying to enhance the activity of antibacterial agents against SCV phenotypes of S. aureus. Richter et al proved that protoporphyrin and defriprone can increase the activity of antibiotics against SCVs of S. aureus, because of their ability of increasing the cell metabolism level.Citation105 The agents that can increase cell metabolism will be a new approach for solving the therapy challenges of SCVs by using them to modify the nanoparticles.
Enhanced therapeutic efficiency in vivo
It will be easier to accumulate the nanoparticles in the infection foci in the body via the phagocytic cells of the mononuclear phagocytic system (MPS) and local impaired lymphatic drainage and enhanced capillary permeability due to the inflammation. In these processes, there are more opportunities that nanoparticles can meet the intracellular and extracellular S. aureus. Many studies demonstrated that the nanoparticles can enhance the treatment efficiency of antimicrobial agents against S. aureus infections in vivo. For example, tilmicosin-loaded hydrogenated castor oil SLNs with lower dosage showed better therapeutic efficacy than free tilmicosin for S. aureus mastitis infection in mice due to the enhanced bioavailability and sustained-release performance.Citation106 Xie et al proved that the gold nanoclusters showed excellent therapeutic effect on both the bacteremia model and the skin infection model induced by MRSA, due to the reasonable circulation time and the ultra-small size of the nanoclusters.Citation107 It was reported that the anti-MRSA activity of vancomycin pH-responsive lipid nanoparticles was 1.8-fold higher than vancomycin in vivo.Citation108 In addition, some inorganic materials with good biologic activities and low toxicity (vanadium dioxide)Citation109 also showed satisfactory effects on S. aureus infections in vivo. For example, the silica nanoprobe coated with vancomycin and decorated with polyelectrolyte–cypate complexes can selectively enable rapid (4 hours postinjection) near infrared fluorescence imaging with high sensitivity (105 colony-forming units) and achieve efficient photothermal therapy of MRSA infections in mice. Remarkably, the nanoprobes can afford a long-term tracking (16 days) of MRSA infection changes.Citation110 The bacteria-responsive functional nanomaterials will offer chance to combat the infections of bacterial resistance. The nanohydrogel systems were also considered as an effective medium to cope with the challenges of S. aureus because of the strong adhesiveness to infection site, sustained drug release, reduced frequency of dosage, and excellent inhibition of bacterial growth. Nimal et al found that chitosan gel containing tigecycline nanoparticle displayed significant activities against S. aureus using a Drosophila melanogaster infection model.Citation111
Current challenges
Nanoparticles armed with antimicrobial agents are used as a potential weapon against S. aureus infection due to their specific biologic performance, and have shown more advantages than the traditional preparations. But the studies of nanosystems against S. aureus infections are not complete and we still face the challenges from nanosystems, reasonable large-scale production, and so on. There are some contradictions between the absence of enhanced activity against intracellular pathogens and intracellular accumulation of some antibacterial drugs through nanoparticles because of the dormant or quiescent state of bacteriaCitation112,Citation113 and the drug inactivation in the intracellular unfavorable environment. Compared to the free rifampin, the activity against mycobacterium was not enhanced by the polyisobutyl cyanoacrylate nanoparticle, although the amount of intracellular rifampin was increased by the nanoparticles.Citation114 The SCV phenotype of S. aureus holds lower metabolism level and growth rate than the normal phenotype, because of which the antimicrobial agents hardly kill them. Currently, nanoparticles are scarcely developed to fight against the SCV phenotype of S. aureus. To eliminate the persisting SCV phenotype, establishing new approaches for development of nanoparticles is necessary. Combination treatment, either through the incorporation of multiple antibacterial agents that have synergistic effects, or the combined use of antibiotics and other intervention drugs with different antibacterial mechanisms, may be a promising way to combat the quiescence or dormancy of S. aureus. Another major challenge that we are facing is the premature release of nanoparticles. The key issue in the successful treatment of intracellular S. aureus with biofilm and drug resistance is the stability of the nanoparticles in transport, that is, it is important to ensure that the nanoparticles have not prematurely released the drug before reaching the nidus and that the drug inactivation on the way is avoided. Unfortunately, nanoparticles with an ideal ability to reach the target site without premature drug release are hardly achieved. Another insurmountable challenge is to make use of the nanoparticle payload antimicrobial agents to counteract MPS externally localized intracellular infections. As we all know, uptake by mononuclear phagocytes is highly beneficial in treating MPS infections. However, S. aureus can infect nonprofessional phagocytes, that is, enterocytes, hepatocytes, fibroblasts, and epithelial cells. The low phagocytic capacity of these cells prevents the S. aureus in the infected non-MPS tissues from being targeted. Therefore, the current antimicrobial agent nanoparticle systems should be endowed with the performance of distinguishing between the infected and the healthy cells and tissues, and have specific drug release according to the affected environment. Currently, the performance of antibacterial drug-loaded nanoparticles is evaluated in vitro and in vivo. Because of the transportation complexity and unpredictability of nanoparticles in vivo, the clinical efficacy of nanoparticles is still suspect, and their clinical evaluation should be strengthened.
As far as the nanoparticles are concerned, they should be nontoxic, highly loading, of low cost, and capable of reproducible manufacturing and validated characterization in order to achieve better clinical application. Unfortunately, current nanoparticle delivery systems seldom achieve these requirements (). For example, the poor drug loading capacity and instability of the liposomes remain important issues.Citation41 Low loading capacity of polymeric nanoparticles for the polar antimicrobial agents is always faced due to the mutual repulsion between the hydrophilic active molecules and the hydrophobic polymers. The lack of reasonable large-scale production is still another bottleneck for polymeric nanoparticles. Although SLNs overcome some disadvantages of liposomes and polymeric nanoparticles, the loading capacity and premature release are still the major challenges. There is no selectivity for metal ion nanoparticles to eukaryotic cells and bacterial cells, so effective transport of these nanoparticles to infected site must be a focus.Citation115 Other nanoparticles, that is, β-tricalcium phosphate nanoparticles, are also beset by these problems. The loading capacity as well as the stability of nanoparticles are being improved by the modification and the combination of various advantages of different nanoparticles. For example, a novel complex drug nanocarrier that combined the technologies of inorganic (hydroxyapatite) and organic nanomaterials (chitosan/konjac glucomannan) and liposomes offered high loading capacity, sustained release profiles, and strong activity against biofilms of S. aureus compared with free vancomycin.Citation116 The multifunctional nanoparticle delivery systems with definite clinical efficacy, affordability, and good compliance should be developed to avoid the disadvantages of current nanoparticles and to possess combined advantages of various nanosystems.
Table 5 The disadvantages of various nanoparticle drug delivery systems
Future perspectives
Facing the therapeutic challenges of S. aureus infections, we have to discover and develop more new nanosystem methods in order to effectively treat the S. aureus infections. As mentioned earlier, S. aureus can live both in extracellular and different subcellular structures. The intracellular efficacy of nanoparticles not only depends on the release of antimicrobial agents and high cell-associated drug levels but also the localization between drugs and intracellular bacteria.Citation90 Currently, the research focuses on whether the antimicrobials could penetrate membrane into cells but seldom on the drug delivery and release in the subcellular structure. The intracellular colocalization of different nanoparticles with S. aureus via the change in its physicochemical properties and proper modification should be strengthened to achieve the better colocalization between drugs and intracellular S. aureus and thus achieve satisfactory therapy.
It is well known that the pH of the infected site and the intracellular environment is lower than those of the healthy tissue and the extracellular environment, respectively. It is more promising for pH-sensitive nanoparticles to combat the infection of S. aureus.Citation117 Among various nanoparticles, the nanogel is pH-dependent and will be possible for targeted therapy of S. aureus infection. The loaded drug release of nanogel is more rapid in lower pH. It was reported that the nanogel was released in a small amount of their payload antimicrobial agents in the milk of the infected mammary gland with a pH of 7.0~7.4, while it was released quickly and completely in the lower pH value (5.0~5.5) of the intracellular endosomes and lysosomes where the S. aureus resided.Citation118,Citation119 In addition, nanogel can adhere to the mammary gland for a long time, because of the strong mucous adhesiveness, and penetrate into the mammary epithelial cell easily due to the small size, larger surface power, and stronger bioadhesion of gel material (eg, sodium alginate, chitosan). These merits will contribute to transporting of their payload drug into the infected sites and cells. Therefore, the nanogel will be an effective weapon to achieve the target treatment for S. aureus and should be given more attention (), especially for the nanogel combined with other nanoparticles.
Figure 4 The mechanism of nanogel releasing drug in the mammary.
Abbreviation: S. aureus, Staphylococcus aureus.
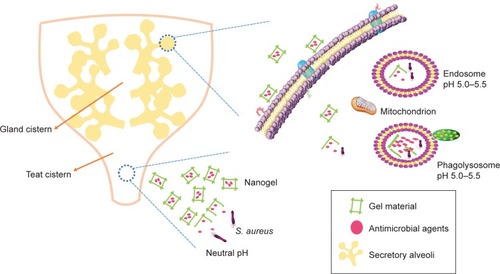
Facing the situation that the evolution of pathogens and resistance production of pathogenic bacteria are faster than the discovery and development of new drugs, the nanoparticle delivery systems with multiple bactericidal mechanisms, eg, coating or conjugation antimicrobial agents via antimicrobial peptides and antibacterial enzymes, should be put on a high level.Citation120,Citation121 In addition, conventional antimicrobial agents or treatment measures may not be able to completely remove all bacteria and thus lead to the bacterial persistence after treatment. The challenges from biofilms and SCVs of S. aureus are still difficult to cope with. In the future, the antimicrobial photodynamic therapy (APDT) and photon-induced photoacoustic streaming (PIPS) should be paid more attention. It is reported that APDT and PIPS combined with nanoparticles may destroy the functional integrity of bacterial cell walls, DNA, biofilm, and membrane proteins of bacteria.Citation122–Citation124 Shrestha et al found that APDT with chitosan-conjugated rose bengal nanoparticles (CSRBnps) achieved endotoxin inactivation and cleaned all the tested inflammatory factors from macrophages. The CSRBnps with APDT showed the ability to effectively inactivate endotoxins.Citation125
Nanoparticle-mediated antimicrobial drug delivery is a multiple and intricate dynamic process in vivo, including the absorption, distribution, metabolism, excretion, and drug release. The dynamic process can influence the uptake rate and fate of nanoparticles and their payload drugs. Therefore, the thorough studying of the dynamic process of nanoparticles in the cells and tissues as well as the invasion mechanism of S. aureus is a prerequisite to develop appropriate nanosystems for effective S. aureus infection therapy. In the future, the smart nanosystems with simulated invasion mechanism of S. aureus and simultaneously aimed at intracellular S. aureus, S. aureus with biofilm, and resistant S. aureus should be developed by studying the transformation mechanisms of nanoparticles at molecular, cellular, and animal levels to achieve the satisfactory effects. The smart nanoparticles aimed at the invasion process of S. aureus should be developed to improve the therapy effects of S. aureus infection disease. The erythrocyte membrane-coated nanogel (red erythrocyte membrane-coated [RBC]-nanogel) system using as a “trap” was discovered according to the features of penetration of cell membrane of S. aureus ().Citation126 The RBC-nanogel can use the loaded antimicrobial agents to kill S. aureus when the bacteria realize specific receptors of erythrocyte membrane and then enter into RBC-nanogel by secreting β-toxin and δ-toxin.
Acknowledgments
This work was supported by the National Natural Science Foundation of China (grant no 31772797).
Disclosure
The authors report no conflicts of interest in this work.
References
- von EiffCBeckerKMachkaKStammerHPetersGNasal carriage as a source of Staphylococcus aureus bacteremia. Study GroupN Engl J Med20013441111611136954
- ChambersHFThe changing epidemiology of Staphylococcus aureus?Emerg Infect Dis20017217818211294701
- RasmussenGMoneckeSBrusOEhrichtRSöderquistBLong term molecular epidemiology of methicillin-susceptible Staphylococcus aureus bacteremia isolates in SwedenPLoS One2014912e11427625479442
- PosadowskaUBrzychczy-WłochMPamułaEPerlTMCullenJJWenzelRPGentamicin loaded PLGA nanoparticles as local drug delivery system for the osteomyelitis treatmentActa Bioeng Biomech2015173414826687562
- GordonRJLowyFDPathogenesis of methicillin-resistant Staphylococcus aureus infectionClin Infect Dis200846Suppl 5S350S35918462090
- ConlonBPStaphylococcus aureus chronic and relapsing infections: evidence of a role for persister cellsBioessays2014361099199625100240
- ZhouJFangTWangYThe controlled release of vancomycin in gelatin/β-TCP composite scaffoldsJ Biomed Mater Res2012100A922952301
- BanburyMKExperience in prevention of sternal wound infections in nasal carriers of Staphylococcus aureusSurgery20031345 SupplS18S2214647029
- MichaelIOGabrielOEPattern of renal diseases in children in mid-western zone of NigeriaSaudi J Kidney Dis Transpl200314453954417657132
- BoucherHWTalbotGHBenjaminDK10 x ′20 Progress– development of new drugs active against gram-negative bacilli: an update from the Infectious Diseases Society of AmericaClin Infect Dis201356121685169423599308
- JagielskiTPuaczELisowskiAShort communication: antimicrobial susceptibility profiling and genotyping of Staphylococcus aureus isolates from bovine mastitis in PolandJ Dairy Sci201497106122612825087034
- MelchiorMBVaarkampHFink-GremmelsJBiofilms: a role in recurrent mastitis infections?Vet J2006171339840716624706
- HalasaTHuijpsKØsteråsOHogeveenHEconomic effects of bovine mastitis and mastitis management: a reviewVet Q2007291183117471788
- LoiselleMCSterCTalbotBGImpact of postpartum milking frequency on the immune system and the blood metabolite concentration of dairy cowsJ Dairy Sci20099251900191219389948
- ClementSVaudauxPFrancoisPEvidence of an intracellular reservoir in the nasal mucosa of patients with recurrent Staphylococcus aureus rhinosinusitisJ Infect Dis200519261023102816107955
- KerrnMBStruveCBlomJFrimodt-MøllerNKrogfeltKAIntracellular persistence of Escherichia coli in urinary bladders from mecillinam-treated miceJ Antimicrob Chemother200555338338615681580
- MonackDMMuellerAFalkowSPersistent bacterial infections: the interface of the pathogen and the host immune systemNat Rev Microbiol20042974776515372085
- FreemanAFHollandSMPersistent bacterial infections and primary immune disordersCurr Opin Microbiol2007101707517208513
- PrajsnarTKCunliffeVTFosterSJRenshawSAA novel vertebrate model of Staphylococcus aureus infection reveals phagocyte-dependent resistance of zebrafish to non-host specialized pathogensCell Microbiol200810112312232518715285
- LöfflerBTuchscherrLNiemannSPetersGStaphylococcus aureus persistence in non-professional phagocytesInt J Med Microbiol2014304217017624365645
- SpezialePPietrocolaGRindiSStructural and functional role of Staphylococcus aureus surface components recognizing adhesive matrix molecules of the hostFuture Microbiol20094101337135219995192
- ZecconiAScaliFStaphylococcus aureus virulence factors in evasion from innate immune defenses in human and animal diseasesImmunol Lett20131501–2122223376548
- ClarkeSRBrummellKJHorsburghMJIdentification of in vivo-expressed antigens of Staphylococcus aureus and their use in vaccinations for protection against nasal carriageJ Infect Dis200619381098110816544250
- GarzoniCKelleyWLStaphylococcus aureus: new evidence for intracellular persistenceTrends Microbiol2009172596519208480
- FosterTJGeogheganJAGaneshVKHöökMAdhesion, invasion and evasion: the many functions of the surface proteins of Staphylococcus aureusNat Rev Microbiol2014121496224336184
- BankTLDosenAGieseRFHaaseEMSojarHTAtomic force spectroscopy evidence of non-specific adhesion of Aggregatibacter actino-mycetemcomitansJ Nanosci Nanotechnol201111108450845622400208
- HarraghyNHussainMHaggarAThe adhesive and immunomodulating properties of the multifunctional Staphylococcus aureus protein EapMicrobiology2003149Pt 102701270714523103
- FraunholzMSinhaBIntracellular Staphylococcus aureus: live-in and let dieFront Cell Infect Microbiol201224322919634
- TorresVJPishchanyGHumayunMSchneewindOSkaarEPStaphylococcus aureus IsdB is a hemoglobin receptor required for heme iron utilizationJ Bacteriol2006188248421842917041042
- RasmussenGMoneckeSBrusOEhrichtRSöderquistBLong term molecular epidemiology of methicillin-susceptible Staphylococcus aureus bacteremia isolates in SwedenPLoS One2014912e11427625479442
- WilkeGABubeck WardenburgJWardenburgJBRole of a dis-integrin and metalloprotease 10 in Staphylococcus aureus alpha-hemolysin-mediated cellular injuryProc Natl Acad Sci U S A201010730134731347820624979
- GroszMKolterJPaprotkaKCytoplasmic replication of Staphylococcus aureus upon phagosomal escape triggered by phenol-soluble modulin αCell Microbiol201416445146524164701
- GarzoniCKelleyWLStaphylococcus aureus: new evidence for intracellular persistenceTrends Microbiol2009172596519208480
- KahlBCSmall colony variants (SCVs) of Staphylococcus aureus – a bacterial survival strategyInfect Genet Evol20142151552223722021
- TuchscherrLMedinaEHussainMStaphylococcus aureus phenotype switching: an effective bacterial strategy to escape host immune response and establish a chronic infectionEMBO Mol Med20113312914121268281
- TuchscherrLGeraciJLöfflerBStaphylococcus aureus regulator Sigma B is important to develop chronic infections in hematogenous murine osteomyelitis modelPathogens201763E3128714889
- ProctorRABalwitJMVesgaOVariant subpopulations of Staphylococcus aureus as cause of persistent and recurrent infectionsInfect Agents Dis1994363023127889317
- SendiPProctorRAStaphylococcus aureus as an intracellular pathogen: the role of small colony variantsTrends Microbiol2009172545819162480
- BuiLMGKiddSPA full genomic characterization of the development of a stable small colony variant cell-type by a clinical Staphylococcus aureus strainInfect Genet Evol20153634535526458527
- TuchscherrLHeitmannVHussainMStaphylococcus aureus small-colony variants are adapted phenotypes for intracellular persistenceJ Infect Dis201020271031104020715929
- OuJJDrillingAJCooksleyCReduced innate immune response to a Staphylococcus aureus small colony variant compared to its wild-type parent strainFront Cell Infect Microbiol2016618728083514
- SerraRGrandeRBuffoneGExtracellular matrix assessment of infected chronic venous leg ulcers: role of metalloproteinases and inflammatory cytokinesInt Wound J2016131535824618232
- LooneyWJSmall-colony variants of Staphylococcus aureusBr J Biomed Sci200057431732211204864
- BesierSLudwigAOhlsenKBradeVWichelhausTAMolecular analysis of the thymidine-auxotrophic small colony variant phenotype of Staphylococcus aureusInt J Med Microbiol2007297421722517412637
- AmatoSMFazenCHHenryTCThe role of metabolism in bacterial persistenceFront Microbiol201457024624123
- BassegodaAIvanovaKRamonETzanovTStrategies to prevent the occurrence of resistance against antibiotics by using advanced materialsAppl Microbiol Biotechnol201810252075208929392390
- OyamaTMiyazakiMYoshimuraMTakataTOhjimiHJimiSBiofilm-forming methicillin-resistant Staphylococcus aureus survive in kupffer cells and exhibit high virulence in miceToxins201687198
- ArmsteadALLiBNanomedicine as an emerging approach against intracellular pathogensInt J Nanomedicine201163281329322228996
- LimbertMIsertDKleselNAntibacterial activities in vitro and in vivo and pharmacokinetics of cefquinome (HR 111V), a new broad-spectrum cephalosporinAntimicrob Agents Chemother199135114192014969
- BrionesEColinoCILanaoJMDelivery systems to increase the selectivity of antibiotics in phagocytic cellsJ Control Release2008125321022718077047
- Pinto-AlphandaryHAndremontACouvreurPTargeted delivery of antibiotics using liposomes and nanoparticles: research and applicationsInt J Antimicrob Agents200013315516810724019
- TaberWHMuellerJPMillerPFBacterial uptake of aminoglycoside antibioticsMicro Rev1987514439457
- BuiLMConlonBPKiddSPAntibiotic tolerance and the alternative lifestyles of Staphylococcus aureusEssays Biochem2017611717928258231
- Gómez CasanovaNSiller RuizMMuñoz BellidoJLMechanisms of resistance to daptomycin in Staphylococcus aureusRev Esp Quimioter201730639139629082727
- GardeteSTomaszAMechanisms of vancomycin resistance in Staphylococcus aureusJ Clin Invest201412472836284024983424
- BeckerKBallhausenBKöckRKriegeskorteAMethicillin resistance in Staphylococcus isolates: the “mec alphabet” with specific consideration of mecC, a mec homolog associated with zoonotic S. aureus lineagesInt J Med Microbiol2014304779480425034857
- DeurenbergRHStobberinghEEThe molecular evolution of hospital-and community-associated methicillin-resistant Staphylococcus aureusCurr Mol Med20099210011519275621
- Hall-StoodleyLStoodleyPEvolving concepts in biofilm infectionsCell Microbiol20091171034104319374653
- SinghRRayPDasASharmaMRole of persisters and small-colony variants in antibiotic resistance of planktonic and biofilm-associated Staphylococcus aureus: an in vitro studyJ Med Microbiol200958Pt 81067107319528167
- ParkSBSteadmanCSChaudhariAAProteomic analysis of antimicrobial effects of pegylated silver coated carbon nanotubes in Salmonella enterica serovar TyphimuriumJ Nanobiotechnology20181613129587743
- YazarEBasALBirdaneYOYaparKElmasMTrasBDetermination of intracellular (neutrophil and monocyte) concentrations of free and liposome encapsulated ampicillin in sheepVeterinární Medicína20125125154
- BastariKArshathMNgZHA controlled release of antibiotics from calcium phosphate-coated poly(lactic-co-glycolic acid) particles and their in vitro efficacy against Staphylococcus aureus biofilmJ Mater Sci Mater Med201425374775724370968
- ThomasNThornCRichterKThierryBPrestidgeCEfficacy of poly-lactic-co-glycolic acid micro- and nanoparticles of ciprofloxacin against bacterial biofilmsJ Pharm Sci2016105103115312227519649
- MayaSIndulekhaSSukhithasriVEfficacy of tetracycline encapsulated O-carboxymethyl chitosan nanoparticles against intracellular infections of Staphylococcus aureusInt J Biol Macromol201251439239922705573
- JiangXHZhouWMHeYZWangYLvBWangXMEffects of lipopeptide carboxymethyl chitosan nanoparticles on Staphylococcus aureus biofilmJ Biol Regul Homeost Agents201731373774328956426
- SutherlandIWThe biofilm matrix – an immobilized but dynamic microbial environmentTrends Microbiol20019522222711336839
- ZhouTHSuMShangBCNano-hydroxyapatite/β-tricalcium phosphate ceramics scaffolds loaded with cationic liposomal ceftazidime: preparation, release characteristics in vitro and inhibition to Staphylococcus aureus biofilmsDrug Dev Ind Pharm201238111298130422257380
- RanjanAPothayeeNSeleemMNAntibacterial efficacy of core-shell nanostructures encapsulating gentamicin against an in vivo intracellular Salmonella modelInt J Nanomed20094289297
- RanjanAPothayeeNVadalaTPEfficacy of amphiphilic core-shell nanostructures encapsulating gentamicin in an in vitro salmonella and listeria intracellular infection modelAntimicrob Agents Chemother20105483524352620516284
- HuDLiHWangBSurface-adaptive gold nanoparticles with effective adherence and enhanced photothermal ablation of methicillin-resistant Staphylococcus aureus biofilmACS Nano20171199330933928806528
- AlvesMMBouchamiOTavaresANew insights into antibiofilm effect of a nanosized ZnO coating against the pathogenic methicillin resistant Staphylococcus aureusACS Appl Mater Interfaces2017934281572816728782933
- MillerKPWangLChenYPPellechiaPJBenicewiczBCDechoAWEngineering nanoparticles to silence bacterial communicationFront Microbiol2015618925806030
- ImbuluzquetaEDrug delivery systems for potential treatment of intracellular bacterial infectionsFront Biosci2010151397417
- HuaLHilliardJJShiYAssessment of an anti-alpha-toxin monoclonal antibody for prevention and treatment of Staphylococcus aureus-induced pneumoniaAntimicrob Agents Chemother20145821108111724295977
- ChakrabortySPSahuSKMahapatraSKNanoconjugated vancomycin: new opportunities for the development of anti-VRSA agentsNanotechnology2010211010510320154376
- GuptaPVNirwaneAMBelubbiTNagarsenkerMSPulmonary delivery of synergistic combination of fluoroquinolone antibiotic complemented with proteolytic enzyme: a novel antimicrobial and antibiofilm strategyNanomedicine20171372371238428648640
- HsuCYYangSCSungCTWengYHFangJYAnti-MRSA malleable liposomes carrying chloramphenicol for ameliorating hair follicle targetingInt J Nanomedicine2017128227823829184410
- PornpattananangkulDZhangLOlsonSBacterial toxin-triggered drug release from gold nanoparticle-stabilized liposomes for the treatment of bacterial infectionJ Am Chem Soc2011133114132413921344925
- DeesCSchultzRDThe mechanism of enhanced intraphagocytic killing of bacteria by liposomes containing antibioticsVet Immunol Immunopathol19902421351462336788
- AhaniEMontazerMToliyatTMahmoudi RadMHarifiTPreparation of nano cationic liposome as carrier membrane for polyhexam-ethylene biguanide chloride through various methods utilizing higher antibacterial activities with low cell toxicityJ Microencapsul201734212113128609225
- BaşALSimşekAErganişOCorluMBasALSimsekAEfficacies of liposome-encapsulated enrofloxacin against Staphylococcus aureus infection in Anatolian shepherd dog monocytes in vitroDtsch Tierarztl Wochenschr2005112621922316028487
- BaşLASimşekACorluMYazarEElmasMDeğimZGDetermination of intracellular concentrations of free and two types of liposome-encapsulated enrofloxacin in anatolian shepherd dog monocytesJ Vet Med B Infect Dis Vet Public Health200249628929312241030
- ZhaoGHuCXueYIn vitro evaluation of chitosan-coated liposome containing both coenzyme Q10 and alpha-lipoic acid: cytotoxicity, antioxidant activity, and antimicrobial activityJ Cosmet Dermatol201817225826228722258
- NigatuASAsharHSethuramanSNElastin-like polypeptide incorporated thermally sensitive liposome improve antibiotic therapy against musculoskeletal bacterial pathogensInt J Hyperthermia201834220120829278945
- XieSYangFTaoYEnhanced intracellular delivery and antibacterial efficacy of enrofloxacin-loaded docosanoic acid solid lipid nanoparticles against intracellular SalmonellaSci Rep201771411028646211
- UskokovićVDesaiTASimultaneous bactericidal and osteogenic effect of nanoparticulate calcium phosphate powders loaded with clindamycin on osteoblasts infected with Staphylococcus aureusMater Sci Eng C Mater Biol Appl20143721022224582242
- ChouJValenzuelaSGreenDWAntibiotic delivery potential of nano- and micro-porous marine structure-derived β-tricalcium phosphate spheres for medical applicationsNanomedicine2014981131113924471502
- AkbariVAbediDPardakhtyASadeghi-AliabadiHCiprofloxacin nano-niosomes for targeting intracellular infections: an in vitro evaluationJ Nanopart Res2013154114
- GeiserMUpdate on macrophage clearance of inhaled micro- and nanoparticlesJ Aerosol Med Pulm Drug Deliv201023420721720109124
- XieSTaoYPanYBiodegradable nanoparticles for intracellular delivery of antimicrobial agentsJ Control Release201418710111724878179
- GenestetCBernard-BarretFHodilleEAntituberculous drugs modulate bacterial phagolysosome avoidance and autophagy in Myco-bacterium tuberculosis-infected macrophagesTuberculosis (Edinb)2018111677030029917
- SahayGAlakhovaDYKabanovAVEndocytosis of nanomedicinesJ Control Release2010145318219520226220
- SémiramothNdi MeoCZouhiriFSelf-assembled squalenoylated penicillin bioconjugates: an original approach for the treatment of intracellular infectionsACS Nano2012653820383122482704
- AllenTMAustinGAChonnALinLLeeKCUptake of liposomes by cultured mouse bone marrow macrophages: influence of lipo-some composition and sizeBiochim Biophys Acta19911061156641995057
- CouvreurPVauthierCNanotechnology: intelligent design to treat complex diseasePharm Res20062371417145016779701
- BeningoKAWangYFc-receptor-mediated phagocytosis is regulated by mechanical properties of the targetJ Cell Sci2012115849856
- KalhapureRSSonawaneSJSikwalDRSolid lipid nanoparticles of clotrimazole silver complex: an efficient nano antibacterial against Staphylococcus aureus and MRSAColloids Surf B Biointerfaces201513665165826492156
- KalhapureRSMocktarCSikwalDRIon pairing with linoleic acid simultaneously enhances encapsulation efficiency and antibacterial activity of vancomycin in solid lipid nanoparticlesColloids Surf B Biointerfaces201411730331124667076
- LiYSuTZhangYHuangXLiJLiCLiposomal co-delivery of daptomycin and clarithromycin at an optimized ratio for treatment of methicillin-resistant Staphylococcus aureus infectionDrug Deliv201522562763724471983
- LiuXLiZWangXNovel antimicrobial peptide-modified azithromycin-loaded liposomes against methicillin-resistant Staphylococcus aureusInt J Nanomedicine2016116781679428008253
- ZhangDKongYYSunJHCo-delivery nanoparticles with characteristics of intracellular precision release drugs for overcoming multidrug resistanceInt J Nanomedicine2017122081210828356731
- KalhapureRSJadhavMRambharoseSpH-responsive chitosan nanoparticles from a novel twin-chain anionic amphiphile for controlled and targeted delivery of vancomycinColloids Surf B Biointerfaces201715865065728763772
- KalhapureRSSikwalDRRambharoseSEnhancing targeted antibiotic therapy via pH responsive solid lipid nanoparticles from an acid cleavable lipidNanomedicine20171362067207728434930
- AuroreVCaldanaFBlanchardMSilver-nanoparticles increase bactericidal activity and radical oxygen responses against bacterial pathogens in human osteoclastsNanomedicine201814260160729155361
- RichterKThomasNZhangGDeferiprone and gallium- protoporphyrin have the capacity to potentiate the activity of antibiotics in Staphylococcus aureus small colony variantsFront Cell Infect Microbiol2017728028690982
- WangXFZhangSLZhuLYEnhancement of antibacterial activity of tilmicosin against Staphylococcus aureus by solid lipid nanoparticles in vitro and in vivoVet J2012191111512021900026
- XieYLiuYYangJGold nanoclusters for targeting MRSA in vivoAngew Chem Int Ed Eng2018571539583962
- JadhavMKalhapureRSRambharoseSNovel lipids with three C18-fatty acid chains and an amino acid head group for pH-responsive and sustained antibiotic deliveryChem Phys Lipids2018212122529305156
- GuoJZhouHWangJNano vanadium dioxide films deposited on biomedical titanium: a novel approach for simultaneously enhanced osteogenic and antibacterial effectsArtif Cells Nanomed Biotechnol201821117
- ZhaoZYanRYiXBacteria-activated theranostic nanoprobes against methicillin-resistant Staphylococcus aureus infectionACS Nano20171154428443828350437
- NimalTRBaranwalGBavyaMCBiswasRJayakumarRAnti-staphylococcal activity of injectable nano tigecycline/chitosan-PRP composite hydrogel using Drosophila melanogaster model for infectious woundsACS Appl Mater Interfaces2016834220742208327508491
- DengYKizerMRadaMIntracellular delivery of nano-materials via an inertial microfluidic cell hydroporatorNano Lett20181842705271029569926
- KaprelyantsASGottschalJCKellDBDormancy in non-sporulating bacteriaFEMS Microbiol Rev1993103–42712868318260
- AnisimovaYVGelperinaSIPeloquinCAHeifetsLBNanoparticles as antituberculosis drugs carriers: effect on activity against myco-bacterium tuberculosis in human monocyte-derived macrophagesJ Nanopart Res200022165171
- LinPLFlynnJLUnderstanding latent tuberculosis: a moving targetJ Immunol20101851152220562268
- MaTShangBCTangHNano-hydroxyapatite/chitosan/konjac glucomannan scaffolds loaded with cationic liposomal vancomycin: preparation, in vitro release and activity against Staphylococcus aureus biofilmsJ Biomater Sci Polym Ed201122121669168121605505
- PeiYMohamedMFSeleemMNYeoYParticle engineering for intracellular delivery of vancomycin to methicillin-resistant Staphylococcus aureus (MRSA)-infected macrophagesJ Control Release201726713314328797580
- MaoCXieXLiuXThe controlled drug release by pH-sensitive molecularly imprinted nanospheres for enhanced antibacterial activityMater Sci Eng C Mater Biol Appl201777849128532100
- XueYXiaXYuBA green and facile method for the preparation of a pH-responsive alginate nanogel for subcellular delivery of doxorubicinRSC Adv20155907341673423
- MurakamiMCabralHMatsumotoYImproving drug potency and efficacy by nanocarrier-mediated subcellular targetingSci Transl Med2011364ra2
- AshbyMPetkovaAHilpertKCationic antimicrobial peptides as potential new therapeutic agents in neonates and children: a reviewCurr Opin Infect Dis201427325826724722240
- Lopez CascalesJJGarroAPorassoRDEnrizRDThe dynamic action mechanism of small cationic antimicrobial peptidesPhys Chem Chem Phys20141639216942170525198294
- GeorgeSKishenAAugmenting the antibiofilm efficacy of advanced noninvasive light activated disinfection with emulsified oxidizer and oxygen carrierJ Endod20083491119112318718378
- GeorgeSKishenAPhotophysical, photochemical, and photobiological characterization of methylene blue formulations for light-activated root canal disinfectionJ Biomed Opt200712303402917614737
- ShresthaACordovaMKishenAPhotoactivated polycationic bioactive chitosan nanoparticles inactivate bacterial endotoxinsJ Endod201541568669125637193
- ZhangYZhangJChenWErythrocyte membrane-coated nanogel for combinatorial antivirulence and responsive antimicrobial delivery against Staphylococcus aureus infectionJ Control Release201726318519128087406