Abstract
Preparation of poly (ethylene glycol) (PEG)-grafted chitosan is essential for improving the biocompatibility and water solubility of chitosan. Presently available methods for this have limitations. This article describes a new method for preparing PEGylated chitosan nanoparticles. For this chitosan was chemoselectively modified using a novel scheme at the C6 position of its repeating units by PEG. The amine groups at the C2 position of the chitosan were protected using phthalic anhydride. Sodium hydride was used to catalyze the etherification reaction between chlorinated chitosan and methyl-PEG, and PEG-grafted chitosan was successfully synthesized. Each step was characterized using 13C nuclear magnetic resonance and Fourier transform infrared. After PEGylation the phthaloylated chitosan was successfully deprotected using hydrazine monohydrate. The synthetic scheme proposed demonstrates a new method for grafting PEG onto chitosan with a moderate degree of substitution. The potential of this polymer in nanoparticle preparation using an ionic gelation method and its gene delivery potentials were investigated by complexing a fluorescently labeled control siRNA. The result showed that suitable nanoparticles can be synthesized using this polymer and that they have capacity to carry genes and provide adequate transfection efficacy with no toxicity when tested in neuronal cells.
Introduction
Chitosan is a linear polymer composed of β-(1–4)-2-amino-2-deoxy-D-glucopyranose units. Being a polycationic, nontoxic, biodegradable, and biocompatible polymer, chitosan has attracted much attention and has wide applications in biotechnology, pharmaceutical, textile, food, cosmetics, and agricultural industries.Citation1,Citation2 Current research with chitosan focuses on its use as a novel drug, gene, peptide, and vaccine delivery vehicle, and scaffold for targeted delivery and tissue engineering applications.Citation3–Citation6 Chitosan has achieved much interest compared with chitin and cellulose due to the presence of primary amine groups present in its repeating units. The presence of primary amine groups makes chitosan an excellent cell transfectant. Like polyethyleneimine, chitosan exhibits a “proton sponge” effect, which refers to the swelling behavior of the polymer on encountering an acidic pH inside the cell’s endosome, making it an efficient carrier for therapeutic molecules.Citation7–Citation9 A number of chitosan derivatives have been synthesized in the past with modification on the primary amine groups of the polymer.Citation10,Citation11 Chitosan is soluble only in acidic aqueous solutions due to the presence of amine groups that become protonated, and thus its poor solubility in other organic solvents has become a major drawback, limiting its effective utilization.
To facilitate the effective use of chitosan in gene delivery and other biomedical applications, it is necessary to make chitosan soluble either in water or organic solvents. Such attempts can be made by graft copolymerization of chitosan through chemical modifications with other polymers, such as poly (ethylene glycol) (PEG), of different molecular weights.Citation12 This allows chitosan to retain its inherent characteristics of, for example, molecular structure and length, and allows for a variety of chemical modifications or reactions on its side chain. However, chemical modification at amine groups leads to a change in the fundamental skeleton of chitosan, causing it to lose its original physiochemical and biochemical activities.Citation12 Recently, modifying hydroxyl groups of chitosan has gained much importance as it does not influence the characteristic structural and functional features of chitosan.
PEG is widely used as a graft-forming polymer. It is soluble in both water and organic solvents, has no toxicity, antigenicity, and immunogenicity, and is biodegradable and biocompatible.Citation13 PEGylation of chitosan through hydroxyl groups was first proposed by Gorochovceva and Makuska.Citation14 PEG has been used mainly as a graft polymer, where it is used as a crosslinker and forms interconnected channels to enable drug release.Citation15,Citation16 Various preparation techniques can be used to prepare chitosan nanocarriers by altering parameters such as concentration of the polymer or the crosslinker, the molecular weight of chitosan, the ratio of drug/gene:polymer, pH, and finally stabilizers or surfactants. All these factors collectively affect the structural and morphological properties of chitosan nanoparticles and the release rate of the loaded therapeutic molecule.Citation17
This study aims to achieve facile chemoselective conjugation of PEG at the hydroxyl group of chitosan, where the amine groups of chitosan were first protected using phthalic anhydride.Citation18 Nanoparticles were formed using an ionic gelation method. The concentration of the polymer versus crosslinker and the pH of the solution were adjusted with reference to our previously optimized study.Citation19 The current study opens up the future application of forming PEG-grafted chitosan nanoparticles for efficient gene/drug delivery.
Materials and methods
Materials
Low-molecular-weight chitosan was obtained from Wako (Richmond, VA, USA), having a viscosity of 5–20 cP and a degree of deacetylation of 80.0%. Poly (ethylene glycol) monomethyl ether (MW 5000), phthalic anhydride, sodium hydride (NaH), pyridine, hydrazine monohydrate, sodium tripolyphosphate (TPP), glacial acetic acid, agarose (low gelling temperature), and ethidium bromide (10 mg/mL) of analytical grade were obtained from Sigma-Aldrich (Oakville, ON, Canada). Anhydrous tetrahydrofuran (THF), anhydrous N,N-dimethylformamide (DMF), sodium hydroxide (NaOH) and methanol were obtained from Thermo Fisher Scientific (Ottawa, ON, Canada). Thionyl chloride (SOCl2) was obtained from VWR (Mississauga, ON, Canada). For dilution purposes, ultra pure double-distilled water (ddH2O) was used from a laboratory-installed Barnstead Nanopure diamond™ water supply unit. siGLO (Green) transfection indicator (20 nmole) was obtained from Dharmacon (Lafayette, IN, USA). CellTiter 96® AQueous One Solution Reagent was purchased from Promega (Madison, WI, USA) to perform cell viability assay. TrackIt 10 bp DNA ladder (0.5 μg/μL) was obtained from Invitrogen (Burlington, ON, Canada). Neuro2a cell line and Eagle’s minimum essential medium (EMEM) were obtained from Cedarlane (Burlington, ON, Canada) and supplemented with 10% fetal bovine serum (FBS) from Invitrogen (Burlington, ON, Canada).
Deacetylation of chitosan
Commercially available low-molecular-weight chitosan (5 g) was added to a 40% (w/v) aqueous NaOH solution. The mixture was stirred for 4 hours at 110°C under a nitrogen atmosphere. After 4 hours the mixture was vacuum filtered, pulverized, and was again treated with 40% (w/v) NaOH under similar conditions. The resultant product (b) in was freeze-dried.Citation20
Figure 1 Schematic of O-PEGylated chitosan polymer preparation using NaH and THF: A) chitosan; B) deacetylated chitosan; C) phthaloyl chitosan; D) chlorinated phthaloyl chitosan intermediate; E) PEGylated phthaloyl chitosan; and F) PEGylated chitosan.
Abbreviations: NaH, sodium hydride; THF, tetrahydrofuran.
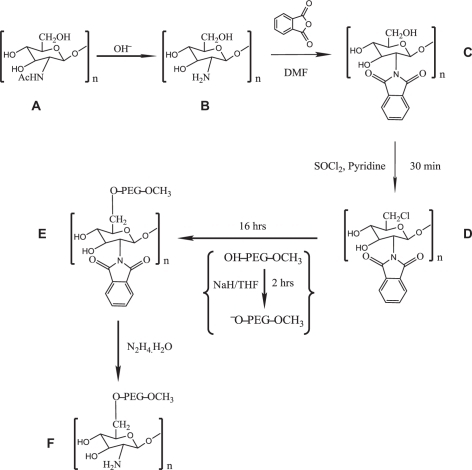
Phthaloylation of chitosan
Deacetylated chitosan (1.00 g) was added to a solution of phthalic anhydride (2.76 g) in 20 mL of DMF. The mixture was stirred for 8 hours at 120°C under a nitrogen atmosphere. The resultant product (c) in was cooled to room temperature and precipitated in ice cold water. The precipitate was filtered, washed with methanol overnight, and vacuum dried.Citation18,Citation21
Synthesis of PEGylated chitosan (chitosan-O-PEG)
We employed a novel scheme to PEGylate chitosan chemoselectively. The scheme depicted in utilizes the activation of PEG by NaH, used as a catalyst to conjugate PEG and chitosan. The synthesis was accomplished in 3 steps: 1) chlorination of chitosan, 2) activation of OH-PEG-OCH3 (mPEG) with NaH, and 3) grafting activated mPEG onto chlorinated chitosan. To prepare chlorinated chitosan, SOCl2 was added in 10-fold excess compared to phthaloylated chitosan (0.1 g) in 20 mL of pyridine. The reaction was stirred at 80°C for 30 minutes under a nitrogen atmosphere. After 30 minutes, the reaction was cooled to room temperature, precipitated in ice cold water, filtered, and vacuum dried to yield chlorinated-phthaloyl chitosan, product (d) in . PEG was activated by adding 4 g of OH-PEG-OCH3 (mPEG) to a suspension of NaH (10 mg) in 50 mL of anhydrous THF. The reaction was stirred at 60°C for 2 hours under nitrogen atmosphere. After 2 hours, chlorinated-phthaloyl chitosan (60 mg) was added to the reaction mix and stirred for another 16 hours under similar conditions. After 16 hours the reaction was allowed to cool at room temperature and was precipitated in methanol, filtered, and vacuum dried to yield product (e) in .
Deprotection of PEGylated-phthaloyl chitosan
PEGylated-phthaloyl chitosan (100 mg), hydrazine monohydrate (15 mL), and distilled water (30 mL) were mixed and heated at 100°C for 16 hours under constant magnetic stirring. Excess hydrazine monohydrate was removed by evaporating the mixture using a rotary evaporator until a viscous solution was left. The process was repeated 3 times by reconstituting the mix with distilled water each time and then rotary evaporating it until a solid residue was left. The final product was dried under vacuum to obtain the desired PEGylated chitosan.
Preparation of PEGylated chitosan nanoparticles
PEGylated chitosan nanoparticles were prepared by an ionic gelation procedure, as explained in previous studies.Citation22 After the deprotection of PEGylated-phthaloyl chitosan, the polymer was dissolved in 1% (v/v) acetic acid solution to yield a concentration of 0.5 mg/mL and the pH was adjusted to 5. TPP was dissolved in ddH2O to obtain a concentration of 0.7 mg/mL and the pH was adjusted to 3.Citation19 Nanoparticles were formed after the addition of TPP (drop-wise) to the chitosan solution under constant magnetic stirring for 1 hour at room temperature. The nanoparticles obtained were characterized by TEM for their morphology and size.
Gene loading efficiency by the gel retardation assay
The encapsulation/gene loading efficiency of PEGylated chitosan polymer was determined by complexing it with siGLO, a transfection indicator, to form nanoparticles following similar conditions as described in section 2.6. The free siGLO in PBS and siGLO complexed with nanoparticles were run in duplicates on a 4% w/v agarose gel electrophoresis for 4 hours at 55 V in Tris/Borate/EDTA (TBE) buffer (pH 8.3). The siGLO complexation with nanoparticles was performed at a ratio of 200:1 (polymer:gene) (w/w), as determined in our previous study.Citation19 The TBE buffer used contained ethidium bromide at a concentration of 0.5 μg/mL, which is required for the visualization of the RNA bands under UV transilluminator at a wavelength of 365 nm using a ChemiDoc™ XRS System (Bio-Rad, Hercules, CA).
Transfection efficiency and cell viability assay
Mouse neuroblastoma cells (Neuro2a) were seeded in a 96-well plate at a density of 20,000 cells per well in complete growth media (antibiotics-free EMEM with 10% serum). The transfection study was performed after 24 hours of initial seeding. The different tested samples were: chitosan–TPP nanoparticles and free siGLO as controls, chitosan–TPP:siGLO nanoparticles, and PEGylated chitosan–TPP:siGLO nanoparticles (200:1 w/w) as treatment samples. The cells were then incubated at 37°C, 5% CO2 for 4 hours, after which the cells were observed under fluorescence microscope (Nikon Eclipse TE2000-U) at a wavelength of 490 nm. The same treatment and control samples were also used for a cytotoxicity assay. The cytotoxicity assay was performed after 4 hours using Cell-Titer 96® AQueous One Solution Reagent (MTS assay), as per manufacturer’s protocol. After an additional incubation of 4 hours with the MTS assay the absorbance in each well was recorded at 490 nm using a microtiter plate reader (Perkin Elmer® 1420 Multilabel Counter Victor 3TM V). The cell study was performed in triplicate.
Statistical analysis
Values are expressed as means ± standard deviations (SD). The statistical significance was determined by using an independent t-test to compare the values of chitosan-TPP nanoparticles with PEGylated chitosan–TPP nanoparticles (n = 3 for each group). P values of <0.05 were considered significant. Statistical analysis was carried out using Minitab (Minitab, Version 14; Minitab Inc, State College, PA).
Results
Deacetylation of chitosan
The 13C nuclear magnetic resonance (NMR) of deacetylated chitosan confirmed the absence of an acetyl peak (−CH3) at 23.88 ppm and a carbonyl peak (−C═O) at 175.04 ppm present in the commercially available chitosan. NMR: (topological substructural molecular design (TOSS mode) of commercially available chitosan: δC 23.88 (CH3), 59.01 (C-2), 61.76 (C-6), 75.64 (C-5, 3), 82.51 (C-4), 105.68 (C-1), 175.04 (C═O). 13C CP/MAS NMR: (TOSS mode) of deacetylated chitosan: δC 60.76 (C-2, 6), 75.84 (C-4, 5, 3), 102.80 (C-1).
Phthaloylation of chitosan
The 13C NMR in TOSS mode of phthaloylated chitosan confirmed the appearance of peaks Phth phenylene and Phth C═O at 134.42 ppm and 169.66 ppm, respectively, and the appearance of Phth C-1,2 and Phth C═O peaks at 131.63 ppm and 169.89 ppm, respectively, in TOSDL mode. 13C CP/MAS NMR: (TOSS mode): δC 58.19 (C-2), 61.76 (C-6), 72.44 (C-3), 75.42 (C-5), 83.91 (C-4), 101.24 (C-1), 124.62, 131.70, 134.42 (Phth phenylene), and 169.66 (Phth C═O); (TOSDL mode): δC 131.63 (Phth C-1,2) and 169.89 (Phth C═O). Fourier transform infrared (FTIR) of phthaloylated chitosan as represented in : νmax/cm−1 3200–3400 (OH), 1774 (imide C═O), 1710 (imide C═O), 1150–1000 (pyranose), and 720 (arom). FTIR of commercially available chitosan as shown in : νmax/cm−1 1630 (amide I), 1542 (amide II), and 1024 (pyranose).
Figure 2 Fourier transform infrared spectra of the chitosan intermediates and O-PEGylated chitosan: A) deacetylated chitosan; B) phthaloylated chitosan: peaks at 1774 cm−1and 1702 cm−1. The OH groups of phthaloylated chitosan was chlorinated using thionyl chloride, represented by the reduction in peak: from 3500 to 3200 cm−1; C) OH-PEG-OCH3, M.W. 5,000; D) 6-O-PEG-g-2-N-phthaloyl chitosan; E) PEG-grafted chitosan: disappearance of peaks 1774 cm−1 and 1702 cm−1.
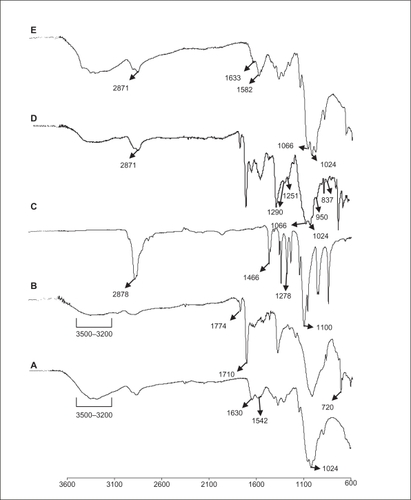
Synthesis of PEGylated chitosan
The FTIR spectra presented in represent PEGylated chitosan with the characteristic peaks at νmax/cm−1: 2871 (C-H stretching), 1066 (C-O stretching), 1290, 1251, 950 and 837, confirming the PEG5000 substitution when compared to that represents the FTIR spectra of PEG only with the characteristic peaks at νmax/cm−1: 2878 (C-H stretching), 1100 (C-O stretching), 1466, and 1278.
Deprotection of PEGylated-phthaloyl chitosan
The FTIR spectra presented in represent deprotected PEGylated chitosan with the characteristic peaks at νmax/cm−1: 2878 (C-H stretching), 1066 (C-O stretching) belonging to PEG, the appearance of 1633 (amide I), 1582 (amide II), and 1024 (pyranose) belonging to chitosan, and the disappearance of 1774 (imide C═O), 1710 (imide C═O) belonging to the phthaloyl group, as shown in .
Preparation of PEGylated chitosan nanoparticles
Deprotected PEGylated chitosan polymer was crosslinked with TPP by the electrostatic interaction between the cationic charges of the primary amine groups on chitosan with the anionic charges of TPP. The crosslinking results in the formation of nanoparticles ranging from 100 to 150 nm in size as determined by transmission electron microscopy (TEM). represents the TEM image of the PEGylated chitosan polymer (magnification: 57000X). represents the chitosan–TPP nanoparticles (magnification: 22000X) and represents the PEGylated chitosan-TPP nanoparticles at magnification 57000X and 135000X respectively. It was observed that PEGylation yielded a spherical shape to the nanoparticles.
Gene loading efficiency of PEGylated chitosan nanoparticles
In order to evaluate the complexation of siGLO with PEGylated chitosan-TPP nanoparticles, a gel retardation assay was performed, wherein a 10 bp DNA ladder was used as a reference, free siGLO at a concentration of 6 μg/mL of PBS solution was used as the control and PEGylated chitosan–TPP nanoparticles complexing siGLO at a ratio of 200:1 (w/w), as determined in our previous study, was used as the treatment sample.Citation19 The gel, under a UV transilluminator, shows bands indicating the presence of unbound siGLO in the suspension, as shown in , for the control sample (ie, free siGLO in PBS), whereas there is no band in a treatment sample, which confirms the complete complexation of the siGLO with PEGylated chitosan nanoparticles. The strong interaction pertains to the positive charges available on the chitosan chain after the deprotection procedure on the polymer allowing the interaction with the negatively charged siGLO and TPP.
Figure 4 Gel retardation assay: lane 1) control 10 bp DNA ladder; lane 2 and 3 control siGLO, and lane 4 and 5 are PEGylated chitosan complexed siGLO at a ratio of 200:1 (w/w). In all groups the siGLO concentration was kept constant at 6 μg/mL. The disappearance of the band size at 40 bp in PEGylated chitosan complexed siGLO (lanes 4 and 5), compared to siGlO control (lanes 2 and 3), indicates the complete complexation of the siGLO.
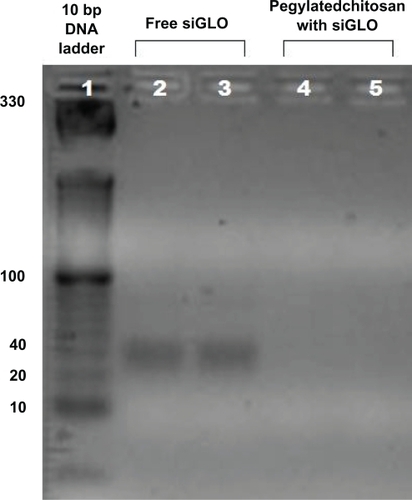
Transfection efficiency and cell viability assay
The transfection efficiency was analyzed by measuring the fluorescence intensity of the siGLO-loaded nanoparticles. siGLO is a transfection indicator, which is a fluorescent oligonucleotide that is localized in the nucleus of the mammalian cell (). Free siGLO, chitosan–TPP nanoparticles, and cells without any treatment sample were used as negative controls. An efficient transfection efficiency was observed with both chitosan–TPP:siGLO and PEGylated chitosan–TPP:siGLO nanoparticles prepared at a weight ratio of 200:1 (). The cell viability assay was performed on these cells after 4 hours, wherein it was observed that cells treated with PEGylated chitosan–TPP:siGLO nanoparticles showed significant increase in cell viability (≈70%) compared with the chitosan–TPP:siGLO nanoparticles (≈20%) (independent t-test, P < 0.05), as observed in .
Figure 5 Transfection efficiency of nanoparticles on Neuro2a cells after 4 hours of incubation at 37°C and 5% CO2: a) PEGylated-chitosan-TPP:siGLO nanoparticles; b) chitosan-TPP:siGLO nanoparticles; c) siGLO only (negative control); d) chitosan-TPP nanoparticles (negative control); e) cells only (negative control)
Abbreviation: TPP, sodium tripolyphosphate.
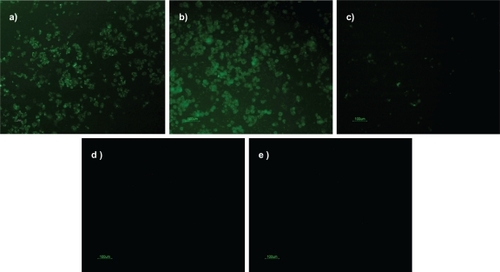
Figure 6 Designed CS-TPP-PEG-siGLO nanoparticle cytotoxicity was investigated using Neuro2a cells. For the experiment, various control nanoparticle formulations were used, the Neuro2a cells were exposed for 4 hours and cell viability was evaluated using spectrophotometer at 490 nm using standard MTS assays (n = 3).
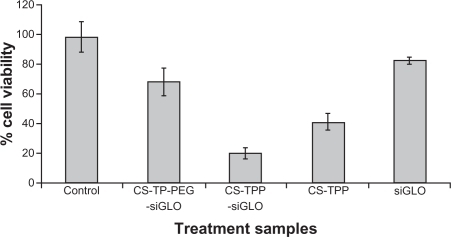
Discussion
The degree of N-acetylation of chitin differentiates it from chitosan. The lower degree of N-acetylation of chitosan widens its application for chemical modifications with the amine groups, imparts greater solubility to the polymer, and enhances its biological properties. Unlike chitin, chitosan is soluble only in acidic aqueous solutions. The solubility of the polymer is an important parameter for having a homogeneous reaction mixture in order to obtain successful chemical modifications. The solubility of chitosan also depends on its molecular weight, its degree of neutralization of amine groups, the ionic strength of the solvent, and the distribution of N-acetyl glucosamine residues along the backbone of the chain.Citation23 In these experiments complete deacetylation of chitosan was achieved after treating it twice with a 40% (w/v) NaOH solution. Products (a) and (b) in represent the schematic of acetylated chitosan (commercially available) and deacetylated chitosan, respectively.
The complete deacetylation is required to achieve a chitosan with fully activated amine groups allowing for further chemical reactions but renders crystalline character to the polymer, making it insoluble in most organic solvents.Citation21 In order to solubilize chitosan in organic solvents and to avoid the interference of amine groups while performing chemical modifications on the backbone, the amine groups of chitosan were protected using phthalic anhydride.Citation21 Since it has been observed that phthaloylation of chitosan in 100% DMF yields partial O-phthaloylation along with N-phthaloylation, we attempted to use DMF/water (95:5 v/v) as a solvent.Citation21 However, it was observed that the product obtained did not solubilize homogeneously in most of the organic solvents, which in turn was a hindrance to perform further chemical reactions. Thus, in this chemical scheme we did not incorporate the use of DMF/water (95:5 v/v) as a solvent to synthesize phthaloylated chitosan.
13C NMR data confirm the successful N-phthaloylation of the product. Product (c) in shows the schematic representation of the N-phthaloyl chitosan. The total suppression of side bands (TOSS mode) exhibits peaks at 124.62 ppm, 131.70 ppm, 134.42 ppm corresponding to phenylene, and 169.66 ppm and carbonyl group of phthaloyl group and the TOSS – dipolar dephasing (TOSDL) mode confirms the absence of CH and CH2 peaks due to their short relaxation time and presence of only 2 peaks 131.63 ppm and 169.89 ppm assigned to C 1, 2 and carbonyl of phthaloyl group, respectively. The IR data show distinct sharp peaks at 1774 cm−1 and 1702 cm−1 corresponding to imide of phthaloyl group (). The phthaloyl chitosan prepared by this method becomes gel-like when precipitated in water, which supports the data shown by Kurita et al of formation of a uniform structure of phthaloylated chitosan.Citation21
The 2-N-phthaloylated chitosan formed was further reacted with thionyl chloride to obtain chlorinated chitosan as represented by product (d) in . The IR data in show the disappearance of the OH peak in the range of 3200 cm−1 to 3400 cm−1 in chlorinated phthaloyl chitosan, confirming the replacement of hydroxyl groups with chlorine and the peaks pertaining to imide bond of phthaloyl group (1774 cm−1 and 1710 cm−1) remain intact.
Simultaneously, mPEG (OH-PEG-OCH3) was activated by NaH in THF to form PEG alkoxide (−O-PEG-OCH3). To this intermediate, chlorinated phthaloyl chitosan was added to form PEG-grafted phthaloyl chitosan. The desired product was precipitated in methanol, with unreacted PEG being soluble in this solvent. This method is the most direct and the easiest way to obtain PEG-grafted chitosan and does not require any intensive purification procedures. Product (e) in represents the schematic of PEGylated phthaloyl chitosan.
The deprotection of the chitosan was successfully achieved by treating the PEGylated phthaloyl chitosan, product (e) in , with hydrazine monohydrate, which causes destabilization of the phthaloyl moiety by creating an excess alkaline condition of pH > 12. Product (f) in represents the final product achieved: PEG-grafted chitosan. The absence of peaks at 1774 cm−1 and 1710 cm−1 in product (e) confirms the complete dissociation of phthalimido group from chitosan and the appearance of peaks 1633 cm−1 (amide I) and 1582 cm−1 (amide II) corresponds to the presence of primary amine groups in chitosan. The peak at 2871 cm−1 refers to the presence of PEG in product (e), . The data on 1H NMR are not shown since it was observed that the multiple peaks of oxymethyl groups in PEG obtained at δ 3.3 to 3.7 cover over the signals of the pyranose ring of chitosan.
The reaction conditions used in the scheme for O-PE-Gylating chitosan are less intensive in terms of operation and purification. However, NaH, used as a catalyst, makes the reaction conditions alkaline which can potentially have a deleterious effect on the phthaloyl group of chitosan. But, a correct proportion of NaH, PEG, and chitosan can optimize the reaction conditions. In our study we used 2:4:1 molar ratio of NaH:PEG:chitosan. The studies conducted by Makuska’s group used Ag2O as a catalyst, producing less alkalinity in the solution, suitable for the etherification reaction between chitosan and PEG. The reaction yielded a high degree of substitution of PEG on chitosan, but as stated by the researchers, the residual silver salts that remain in the reaction lead to degradation of chitosan polymer while undergoing deprotection with hydrazine.Citation24 Also, the overall yield of the PEGylated chitosan was less and involved 28 to 30 hours of reaction time. Even after implementing several cycles of purification on PEGylated chitosan intermediate, it could not completely remove the silver particles. Thus, the procedure involves intensive and multiple steps to obtain the desired product. The use of NaH as a catalyst is more promising than using Ag2O as a catalyst. In our case, the degree of substitution of PEG on chitosan can be increased by using low molecular weight PEG, but not <1000 due to the high solubility of PEG in aqueous as well as organic solvents. We also tried PEGylating chitosan through a scheme followed by Jian and You-Lo, in which phthaloylated chitosan was first swelled in pyridine overnight.Citation25 Other researchers have used NaOH to swell chitosan prior to the reaction in order to achieve homogeneous conditions. NaOH can deprotect the phthaloyl group on chitosan, and therefore its use was avoided and using pyridine as a solvent was favored due to the solubility of phthaloylated chitosan in pyridine. We obtained similar results in terms of yield of PEGylated chitosan by following the protocol of Jian and You-Lo with the exception of the use of PEG acyl chloride followed by multiple steps that take more time (36–40 hours) in terms of reaction and purification at each step to obtain the final product. The total reaction time employed in this scheme, using NaH, was 18 to 20 hours.
The proposed scheme yielded a moderate (15%–50%) degree of substitution of PEG on chitosan, which was soluble in acetone, toluene, chloroform, DMF, and ethyl acetate at 50°C. Also, as reported earlier, O-PEGylated chitosans with free amino groups have a wider application in biotechnology and biomedical systems because of the unchanged poly (glucosamine) skeleton.Citation14 As PEG separates the chitosan backbone, thereby decreasing intermolecular hydrogen bonding and making it water soluble, it acts as an important intermediate to carry out further chemical modifications.
Chitosan nanoparticles were prepared by ionic gelation that involves a mixture of 2 aqueous phases, of which one is the polymer chitosan and the other is a polyanion TPP. The positively charged amino group of chitosan interacts with negatively charged TPP to form complex coacervates with a size of about 100 nm.Citation26–Citation27 The nanoparticles formed can have a significant application as a drug/gene carrier system for biomedical applications both in vitro and in vivo. In this study, we have complexed siGLO, a scrambled siRNA with a fluorescent tag with the nanoparticles prepared from the proposed formulation of PEGylated chitosan polymer. Complete complexation of the siGLO was achieved as confirmed by the gel retardation study. The optimal concentration of siGLO to be used for complexation was initially determined by our group and has been published previously as a characterization study.Citation19 The PEGylated chitosan polymer complexing siGLO was used as a nanocarrier to transfect a neuronal cell line in order to determine the transfection efficiency of the nanoparticle/carrier. It has been reported by many researchers that PEGylation reduces the transfection efficiency of the nanoparticles since PEG, being neutral in charge, does not efficiently interact with the negatively charged cell membrane. However, in this study, the facile chemoselective substitution of PEG on the C6 position was favored, especially so as not to affect the primary amine groups on chitosan that play a major role is gene complexation and cell transfection.
The transfection study, as shown in , confirmed the efficient uptake of PEGylated chitosan nanoparticles (a) compared with chitosan nanoparticles alone (b). This proves that conjugating PEG on the C6 position of the chitosan polymer did not hinder the inherent character of the chitosan polymer to complex and to deliver the siGLO to neuronal cells. To further investigate the effect of nanoparticles on the cells, a cell viability assay was performed. This confirmed the stressed conditions of cells transfected with chitosan nanoparticles alone. The results indicated that although primary amine groups contribute significantly to transfection they may also disrupt the cellular integrity of the cell membrane due to an excessive positive charge on the nanoparticles. The presence of PEG on the nanoparticles reduces steric hindrance and makes nanoparticles more hydrophilic. Also, as observed in , the cytotoxicity was greater for the chitosan nanoparticles complexing siGLO than the chitosan nanparticles alone. This could be inferred from a reduction in size of the nanoparticles after complexing siGLO, which in turn allows more particle interaction with the cell membrane, thereby increasing the surface area. The optimization study based on the particle size and zeta potential of chitosan nanoparticles with and without siGLO has previously been published by our group.Citation19
Conclusion
We achieved successful grafting of PEG on chitosan polymer through a novel scheme of forming PEG alkoxide using NaH as a catalyst. The PEGylated chitosan polymer obtained was easily soluble in water and other organic solvents. The overall procedure is easier and requires less intensive reaction conditions and purification steps to obtain the desired product in a short period of time than the other methods used by other protocols reported in the literature. Also, the procedure does not impart any deleterious effect on the chitosan chain. The PEGylated chitosan nanoparticles successfully complexed siRNA and transfected into the neuronal cell line with minimal cytotoxicity effects. The proposed formulation could have a major implication for biomedical drug targeting strategies involving nanoparticle-mediated targeted delivery.
Acknowledgements
The authors would like to acknowledge the Canadian Institutes of Health Research (CIHR) grant (MOP-93641) to Dr S Prakash, the support of McGill Major Scholarship to M Malhotra, the support of Industrial Innovation Scholarship (IIS) BMP Innovation – NSERC, FQRNT scholarship and Micropharma Limited Scholarship to Catherine Tomaro-Duchesneau. We would like to acknowledge the support of the Centre of Biorecognition and Biosensors, the NMR Facility at Quebec/Eastern Canada High Field NMR Center, the Centre for self-assembled Chemical Structures (CSACS) and the facility of Electron Microscopy Research at McGill University. We would like to acknowledge the guidance of Dr Hani Al-Salami for statistical analysis.
Disclosure
The authors declare no conflicts of interest.
References
- LiQDunnETGrandmasionEWGoosenMFAApplications and properties of chitosanGoosenMFAApplications of Chitin and ChitosanLancasterTechnomic Publishing Company, Inc1992329
- Ravi-KumarMNVA review of chitin and chitosan applicationsReact Funct Polym200046127
- Bernkop-SchnurchAChitosan and its derivatives: potential excipients for peroral peptide delivery systemsInt J Pharm200019411310601680
- IllumLJabbal-GillIHinchcliffeMFisherANDavisSSChitosan as a novel nasal delivery system for vaccinesAdv Drug Del Rev2001518196
- LiuWGYaoKDChitosan and its derivatives – a promising non-viral vector for gene transfectionJ Control Release20028311112220833
- SundararajanVMHowardWTMPorous chitosan scaffolds for tissue engineeringBiomaterials1999201133114210382829
- BoussifOLezoualchFZantaMAMergnyMDSchermanDA versatile vector for gene and oligonucleotide transfer into cells in culture and in-vivo – polyethylenimineProc Natl Acad Sci U S A199592729773017638184
- ChoYWKimJDParkKPolycation gene delivery systems: escape from endosomes to cytosolJ Pharm Pharmacol20035572173412841931
- IshiiTOkahataYSatoTMechanism of cell transfection with plasmid/chitosan complexesBiochim Biophys Acta20011514516411513804
- SkryabinKGVikhorevaGAVarlamovVPChitin and chitosan Production properties and usageMoscowNauka2002 [in Russian].
- Ravi-KumarMNVMuzzarelliRAAMuzzarelliCSashiwaHDombAJChitosan chemistry and pharmaceutical perspectivesChem Rev20041046017608415584695
- SugimotoMMorimotoMSashiwaHSaimotoHShigemasaYChitin and chitosan derivativesCarbohydr Polym1998364959
- HarrisJMZalipskySPoly (ethylene glycol) Chemistry: and Biological ApplicationsWashington, DCAmerican Chemical Society1997489
- GorochovcevaNMakuskaRSynthesis and study of water-soluble chitosan-O-poly (ethylene glycol) graft copolymersEuropean Polymer Journal200440685691
- LinWJLeeHGDesign of a microporous controlled delivery system for theophylline tabletsJ Control Release20038917918712711442
- LinWJLeeHGWangDMThe influence of plasticizers on the release of theophylline from microporous-controlled tabletsJ Control Release20049941542115451599
- LiuXHowardKADongMAndersonMORahbekULThe influence of polymeric properties on chitosan/siRNA nanoparticle formulation and gene silencingBiomaterials2007281280128817126901
- NishimuraSKohgoOKuzuharaHKuritaKChemospecific manipulations of a rigid polysaccharide: syntheses of novel chitosan derivatives with excellent solubility in common organic solvents by regioselective chemical modificationsMacromolecules19912447454748
- MalhotraMKulamarvaASebakSPaulABhathenaJUltrafine chitosan nanoparticles as an efficient nucleic acid delivery system targeting neuronal cellsDrug Dev Ind Pharm20093571972619514987
- ToriiYIkedaHShimojohMKuritaKChemoselective protection of chitosan by dichlorophthaloylation: preparation of a key intermediate for chemical modificationsPolym Bull200962749759
- KuritaKIkedaHYoshidaYShimojohMHarataMChemoselective protection of amino groups of chitosan by controlled phthaloylation: Facile preparation of a precursor useful for chemical modificationsBiomacromolecules200231411866549
- CalvoPRemunan-lopezCVila-JatoJLAlonsoMJNovel hydrophilic chitosan-polyethylene oxide nanoparticles as protein carriersJ Appl Polym Sci200763125132
- KasaaiMRVarious methods for determination of the degree of N-acetylation of chitin and chitosan: a reviewJ Agric Food Chem2009571667167619187020
- MakuskaRGorochvcevaNRegioselective grafting of poly(ethylene glycol) onto chitosan through C-6 position of glucosamine unitsCarbohydr Polym200664319327
- JianDYou-LoHPEGylation of chitosan for improved solubility and fiber formation via electrospinningCellulose200714543552
- JanesKAFresneauMPMarazuelaAFabraAAlonsoMJPolysaccharide colloidal particles as delivery systems for macromoleculesJ Control Release20017325526711516503
- PanYLiYZhaoHZhengJXuHBioadhesive polysaccharide in protein delivery system: chitosan nanoparticles improve the intestinal absorption of insulin in vivoInt J Pharm200224913914712433442