Abstract
Purpose
The objective of the present investigation was to evaluate the antibacterial properties and the biocompatibility of composite electrospun nanofibrous membranes (NFMs) with low-molecular-weight fish scale collagen peptides (FSCP) and chito-oligosaccharide (COS), to determine their potential for use as wound dressings.
Methods
Low-molecular-weight FSCP were combined with COS to prepare nanofibers by electrospinning, and polyvinyl alcohol (PVA) was used for enhancing fiber-forming ability. Transmission electron microscope and scanning electron microscope methods were used to observe bacterial adhesion and the bacterial cell membrane. Fibroblast cell viability was determined using the 3-(4,5-dimethylthiazol-2-yl)-2,5-diphenyltetrazolium bromide (MTT) assay.
Results
The best FSCP/COS mass ratio for electrospinning was 2:1, and the nanofibers had small dimensions ranging from 50 to 100 nm. The NFM showed good antibacterial activities against Gram-positive Staphylococcus aureus and Gram-negative Escherichia coli. The antibacterial activity against S. aureus was higher than against E. coli. The pili and adhesive fimbriae of E. coli promoted bacterial adhesion to the NFM surfaces, and S. aureus biofilms aided S. aureus adhesion on the surface of NFMs. Damage to the bacterial cell membrane indicates that the NFMs could lead to the release of intracellular materials, particularly with S. aureus. In addition, FSCP/COS NFM rapidly increased the permeability of the outer membranes of E. coli. The electrospun NFM with FSCP and COS had good biocompatibility in vitro and supported proliferation of human skin fibroblasts.
Conclusion
FSCP are superior to mammalian collagen, and have feasibility and potency for wound dressings. FSCP/COS NFMs had good anti-bactericidal activity that improved with increased COS, and showed good biocompatibility in vitro and supported the proliferation of fibroblasts.
Introduction
Nanomaterials are a new type of biomedical material that may have a wide range of advantages as medical dressings, including strong adsorption and the ability to assume variable shapes. They can act as antimicrobial barrier films to prevent wound infection and bleeding, and to promote wound healing.Citation1 Electrospinning (ES) is a simple and effective nanofabrication method for preparing nanofibers, with diameters ranging from 5 to 500 nm, 102 to 104 times smaller than those prepared by traditional methods.Citation2 Because of the very high surface area, together with many small secondary structures, electrospun NFMs have a strong adhesive force, good air filtration, high adhesion barrier activity, and heat resistance.Citation3–Citation6 Their structures make NFMs similar to the skin structure of the human body. Some electrospun membranes have been used specifically in biomedical fields for purposes such as biofilms, wound dressing materials, hemostatic materials, artificial blood vessels, drug and gene delivery, and tissue engineering scaffold materials.Citation2,Citation7 High-molecular-weight (MW) polymers are good materials for nanofiber preparation, but to date, the feasibility of electrospinning low-MW polymers such as collagen peptides with MW less than 10,000, combined with COS, has not been studied.
Most collagens used for nanomaterials are from pig and cattle skin and bones, or mammalian leathers.Citation8,Citation9 In recent years the occurrence of human–animal cross-infections has increased concern about using collagen extracts from terrestrial animal sources.Citation10 Consequently, extracts from fish skins, scales, and bones have become a potential alternative source for collagen, and may be superior to mammalian collagen because of the absence of the risk of animal-related contamination. However, there have been no reports concerning the feasibility of using fish scale collagen for nanomaterials preparation. Collagen peptides (CP) are low-MW fragments from collagen.Citation11 Compared with collagen, which has a MW of 300,000 Da and above, collagen peptides enable easier direct absorption by the human body. The lower-MW collagen peptides not only go through the skin’s stratum corneum, but also penetrate the deep dermal layer. Furthermore, they have the same important role as human peptides in tissue repair and reconstruction of skin.Citation12 Collagen peptides can extend the life of skin cells, inhibit lipid peroxidation, and defend against oxidative free radical attacks on DNA.Citation13 In addition, they have other effects such as: the inhibition of tyrosinase activity; improvement of skin immunity and skin water-holding capacity; and promotion of collagen synthesis and dermal hyaluronic acid synthesis. FSCP of low MW have low antigenicity and are nonallergenic, but no work has been done on using them in wound dressings.
Chitosan has a wide inhibition spectrum for Gram-positive and Gram-negative bacteria, yeasts, and molds. Also, it has no cytotoxicity, causes no hemolysis, contains no known allergens, and has excellent biocompatibility and good biological safety, so chitosans from various sources are widely used in the fields of biomedical materials, textiles, and food additives. The oligomer of chitosan, COS, is water-soluble and has similar biological activity to chitosan. With respect to wound dressing, because of the relatively rapid interaction between the wound and the water-soluble, easily swelling COS in a moist healing environment, COS is a more effective wound-healing accelerator than chitosan. PVA is a hydrophilic, nontoxic, biocompatible, and fully degradable synthetic polymer, with excellent film-forming, emulsifying, and adhesive properties.Citation14 Therefore, materials blended with PVA have a great affinity for skin and extracellular matrix materials. Only a few papers have reported on the preparation of electrospun nanomembranes of collagen or chitosan for wound dressing.Citation15–Citation17 Moreover, to date no work has been reported on the electrospinning of low-MW FSCP and COS, and whether or not they could be combined with PVA to obtain composite nanofibrous membranes for wound dressing. Peptide- or collagen peptide-based wound dressing products are still in the early stages of research. Recently, a few studies have focused on the use of peptides for early-stage wound healing.Citation18
In this study, low-MW FSCP and COS were electrospun to form composite nanofibers. The antibacterial properties of the composite nanofibrous membranes were then evaluated, using two typical clinical wound pathogens: Staphylococcus aureus and Escherichia coli. The potency of the composite nanomembranes for wound dressing was tested using the 3-(4,5-dimethylthiazol-2-yl)-2,5-diphenyltetrazolium bromide (MTT) method with clinically relevant human skin fibroblasts.
Materials and methods
Materials
For electrospinning the fish scale collagen peptide/COS nanofibrous membranes, FSCP with an average manufacturer’s specified MW of 800 Da were used (Hubei Reco Bio-Tech Co Ltd, Wuhan, China), and chitosan, with a manufacturer’s specified MW of 8 kDa and deacetylation degree of 90%, was purchased from Shanghai Lanji Technology Development Co, Ltd (Shanghai, China). PVA was purchased from Sinopharm Chemical Reagent Co, Ltd (Shanghai, China). The cells used in this study were human skin fibroblasts (HSF) (Anhui Medical University, Hefei, China). Fetal bovine serum (FBS), dimethyl sulfoxide (DMSO), and Dulbecco’s modified Eagle’s medium (DMEM) were purchased from Sigma-Aldrich Chemical Co (St Louis, MO). All other chemicals were analytical grade.
Types of bacteria
The strains used for antimicrobial activity measurements were S. aureus ATCC 6538 and E. coli ATCC 8739.
Preparation of FSCP/COS nanofibrous membrane
A series of FSCP, COS, and PVA solutions was prepared by dissolving collagen peptides and PVA in water, and COS in methanoic acid, respectively. After PVA was dissolved with at a contentration of 6%, and the contentrations of FSCP and COS were 12%. The FSCP/COS mass ratios were varied between 1:3, 1:2, 1:1, 2:1, and 3:1. During electrospinning, nanofibers were collected on aluminum foil by connecting a high voltage power supply (GDW-A, Beijing Institute of High Voltage Electrical and Mechanical Technology Inc, Beijing, China). A syringe pump (WZ-50C2, Zhejiang University Medical Instrument Co Ltd, Hanzhou, China) was used to deliver the polymer solution at flow rates from 0.3 to 1.5 mL/hour. Electrospinning was done at voltages from 20 to 50 kV with a needle to a collector gap distance of 5 to 15 cm. Finally, the treated membrane was dried for 7 days in a vacuum oven at 37°C.
Nanofiber characterization
The morphology of electrospun FSCP/COS complex fibers was observed using scanning electron microscopy (SEM) (XL-30E; Philips, Tokyo, Japan) operating with an accelerating voltage of 10 or 15 kV. Prior to SEM, the samples were sputter coated with gold. Based on the SEM micrographs, the average diameter and diameter distribution were determined by choosing 100 fibers at random from 1000 × magnification SEM images and analyzing them using image analysis software (Adobe Photoshop 7.0; Adobe Systems Inc, San Jose, CA).
Fourier transform infrared (FTIR) spectroscopy studies were done on compressed films containing potassium bromide pellets and sampled using an FTIR spectrophotometer (Avatar 380; Thermo Scientific, Austin, TX). All spectra were recorded in absorption mode at 2 cm−1 interval wave-numbers from 600 to 3800 cm−1.
Antimicrobial properties of FSCP/COS nanofibrous membranes
The different FSCP/PVA, COS/PVA, FSCP/COS/PVA, and PVA NFMs were treated with UV for 20 minutes. Then 1 mL of the test strain (106 CFU/mL) of S. aureus or E. coli was added to the surface of the NFM and incubated at 37°C for 10 hours. The residual colonies were washed from the NFM and then the decrease in colonies on incubated agar plates was used to evaluate antimicrobial activity. The PVA NFM sample served as the control. The bactericidal activity was calculated using the following equation: Antimicrobial activity (%) = {(C − T)/C} × 100, where C is the number of colonies counted for the control and T is the number of colonies obtained from each tested sample.
The morphology of the bacterial adhesion was observed by SEM.
Integrity of bacterial cell membrane analyses
Bacteria were cultured in liquid lysogeny broth medium in tubes at 37°C. The electrospun membranes were cut into 2 cm × 2 cm squares in a sterile environment and one placed into each tube. After incubating for different times, bacterial cells were washed and then distributed on copper mesh. Cell morphology was examined by transmission electron microscopy (TEM) (JEM-2010; JEOL, Tokyo, Japan) at Tongji University in China. The release of material from bacteria after treatment with NFMs was examined by determining the absorption values at 260 nm and 280 nm.Citation19 Bacterial cultures were harvested, washed and resuspended in sterile physiological saline. The final cell suspension was adjusted to an absorbance at 420 nm of 0.8. A 2 cm × 2 cm portion of membranes was mixed with 3.0 mL of each bacterial cell suspension. PVA NFMs served as the control, and the release over time of materials absorbing at 260 nm and 280 nm was monitored with a 1601 UV–VIS spectrophotometer (Shimadzu, Tokyo, Japan).
Fibroblast cell viability tests
Human skin fibroblasts were seeded in 6-cm culture dishes and cultured in DMEM. The samples for fibroblast cell viability tests were FSCP, COS, PVA, FSCP/COS NFM, and PVA as a positive control. The NFMs were cut into 2 cm × 2 cm squares and placed into the center of the dish. After incubating for different times, cell morphology was examined using an inverted optical microscope (IX-81; Olympus, Tokyo, Japan).
MTT methods were based on the method of Natthan et al with modification.Citation20 The different electrospun membranes were sterilized by UV radiation for 1 hour, then immersed in a serum-free medium containing only DMEM, in an incubator for 24 hours to produce an extraction media of 1 mg/mL. Fresh culture medium (DMEM), was supplemented with 10% FBS, and used as a negative control. HSF cells were plated in 90 μL of DMEM, at a density of 1 × 104 cells/well in 96-well plates, and cultured at 37°C under a 5% CO2/95% atmosphere. When the cells were cultured for 24 hours after plating, the tested extraction media were replaced and the cells were reincubated for 24 hours. After treatment for different times, the tested extraction solutions were removed. Finally, the cells were incubated with 100 μL of a MTT-containing medium (1 mg/mL) for 4 hours. After the medium was removed, the cells were rinsed with phosphate buffered saline (pH 7.4), and the formazan crystals formed in living cells were dissolved in 100 μL DMSO per well. Relative viability (%) was calculated based on the absorbance at 570 nm using a microplate reader (MicroReader 4; Hyperion, Miami, FL).
Statistical analyses
The data analyses were performed using SPSS (SPSS Inc, Chicago, IL). The values were reported as mean ± SD for all of the results. The P-values were 2-tailed and P < 0.05 was considered as significant.
Results and discussion
Electrospun FSCP/COS fibers
Due to the large net charges of collagen peptide and COS, they cannot form nanofibers during electrospinning. PVA can be used to decrease the conductivity of the solution, so it is well suited to enhancing fiber-forming ability.Citation21 For electrospinning of soluble FSCP combined with COS, methanoic acid was used to dissolve COS. The viscosity and conductivity of the solution, the spinning voltage, the flow rate, and the collecting distance were adjusted in preliminary experiments to optimize the electrospinning conditions. During electrospinning of a FSCP/COS solution, the solution viscosity was found to play an important role in nanofiber formation. COS was more viscous than FSCP. When the COS/FSCP mass ratio was more than 1:1, many beads were observed in the nanofibers (). When the COS/FSCP mass ratio was less than 1:1, most fibers had smooth surfaces with no phase separation, which indicates that FSCP was compatible with COS and PVA (–). Some split and ribbon-type fibers were also observed (–), which may have resulted from solvent evaporation during the electrospinning process.
Figure 1 SEM images of FSCP/COS NFMs with different FSCP/COS mass ratios of 1:3 (A), 1:2 (B), 1:1 (C), 2:1 (D), and 3:1 (E); TEM image of FSCP/COS NFM with FSCP/COS at a mass ratio of 2:1 (F).
Abbreviations: COS, chito-oligosaccharides; FSCP, fish scale collagen peptides; NFMs, nanofibrous membranes.

Instead of electrospinning collagen from calf skin using 1,1,1,3,3,3-hexafluoroisopropanol as a solvent,Citation8 in our study, only one organic solvent, methanoic acid, with relatively lower toxicity, was used for FSCP/COS electrospinning. The FSCP/COS fibers have smaller dimensions than mammal collagen fibers from calf skin, with diameters ranging from 50 to 100 nm ().Citation8
To evaluate the composite NFM and its constituent polymers, FTIR spectra were used. After mixing with COS and PVA, the IR spectrum of FSCP changed markedly (). For the composite NFM, peaks at 3788 and 1093 cm−1 were assigned to the stretching vibration of −OH and C−O in COS. In addition, the peak between 3000 and 3500 cm−1 was assigned to the free −OH, and the wide and strong peak was attributed to the increase in the degree of intermolecular association for hydroxyl groups. An absorption peak at 849 cm−1 was also found in the spectrum of NFM, which was assigned to the −CH2 in PVA. Typical peaks for collagen peptides were clearly observed, such as amide I at 1640 cm−1 and the flexing vibrations of N−H at 654 cm−1 (). For the composite NFM, the characteristic peaks of collagen peptide, COS, and PVA were identified, which confirms that the membrane was composed of all three polymers.
Antimicrobial activity of FSCP/COS NFMs
Antimicrobial activity is considered to be one of the most important properties directly linked to wound dressing. FSCP/COS NFMs showed higher antimicrobial activity against S. aureus than against E. coli. The half maximal inhibitory concentration (IC50) value of FSCP/COS NFM with S. aureus was 0.144 g/mL, lower that for E. coli, with an IC50 value of 0.191 g/mL (). The time-dependence of antibacterial activities of different NFM-treated bacteria are shown in . NFM containing COS showed considerable bactericidal activity against E. coli, whereas that without COS was somewhat less active. Therefore, COS plays an important role in the antibacterial activity of FSCP/COS NFMs.
Figure 3 Antibacterial activities of different concentration of FSCP/COS NFMs against S. aureus (▴) and E. coli (▪) (A); Antibacterial activities of different NFMs at 0.8 g per sample against S. aureus (B), and E. coli (C).
Note: *P < 0.05 compared with FSCP NFMs.
Abbreviations: COS, chito-oligosaccharides; FSCP, fish scale collagen peptides; NFM, nanofibrous membranes.
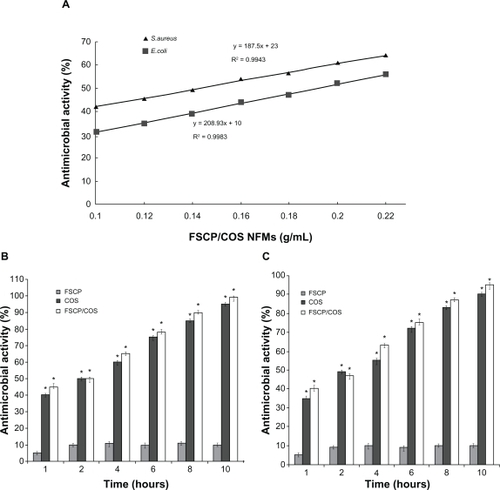
Bacterial adhesion and integrity of bacterial cell membranes
In electrospinning, a polymer solution is pushed out of a nozzle towards a surface under an applied voltage, which produces a disordered fibrous surface with uniform features and roughness. It is also possible to control the direction of the fibers on the surface.Citation22 An extra advantage is that this type of fibrous surface may mimic the structure of the extracellular matrix.Citation23 From electron microscopy photographs, E. coli tightly adhered to the NFMs and S. aureus biofilms were formed on the surface of FSCP/COS NFMs (). It has been reported that some bacteria may have fimbriae, called pili or curli, which aid in attachment to surfaces.Citation24 Many E. coli strains are motile and generally have both sex pili and adhesive fimbriae (). The pili and adhesive fimbriae of E. coli promoted adhesion to the NFM’s surfaces (). Whereas single cells can adhere to surfaces independently of each other, bacteria live on surfaces as a community, within a species, using strain-specific extracellular polymeric substances (EPS) for binding. This assembly of bacteria and EPS is termed a biofilm.Citation25,Citation26 S. aureus is a prominent bacterial pathogen known to form biofilms on many medical implants and host tissues.Citation27 The FSCP/COS NFM permitted the Gram-positive bacterium S. aureus to form biofilms on the membrane surfaces. Furthermore, the biofilms strongly influenced S. aureus adhesion on the surface of NFMs ().
Figure 4 SEM images of bacteria attached on the surfaces of FSCP/COS NFMs after 2 hours of incubation.
Abbreviations: COS, chito-oligosaccharides; FSCP, fish scale collagen peptides; NFMs, nanofibrous membranes.

Figure 5 Photomicrographs of E. coli (A), and S. aureus (B) treated with FSCP/COS NFMs. 1, 2, 3, 4, and 5 refer to the samples at 0, 15, 30, 45, and 60 minutes, respectively.
Abbreviations: COS, chito-oligosaccharides; FSCP, fish scale collagen peptides; NFMs, nanofibrous membranes.
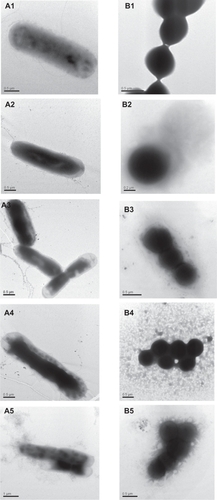
Integrity of bacterial cell membranes was tested and the results show that FSCP/COS NFM treatment resulted in bacterial cell deformation (). Compared with the control, when treated for a prolonged time E. coli were disrupted and covered by an additional tooth-like layer (). The photomicrographs show that FSCP/COS NFM damaged the cell membranes of dividing cells. In photomicrographs of FSCP/COS NFM-treated S. aureus, the membranes of cells were disrupted in the constricting region (–), with loss of cell contents through leakage (). However, cells of S. aureus that were not dividing were not visibly affected except for the thickening of their cell walls. Thus, membrane damage may be one mechanism for inactivation of both E. coli and S. aureus treated with FSCP/COS NFMs.
The cytoplasm and membranes of bacteria are the target for many inhibitory agents. When bacterial membranes become compromised by interaction with inhibitory agents, initially the low-MW substances such as K+ and PO43− tend to leach out, followed by DNA, RNA, protein and other higher MW materials. The intracellular nucleotides in solution are easily detected by absorption at 260 nm, and the protein at 280 nm, which serves as an indication of membrane damage.Citation19 When E. coli suspensions were treated with FSCP/COS NFMs, the OD260 values increased rapidly at first then at a decreasing rate after 60 minutes (). When S. aureus suspensions were treated with the NFMs, the OD260 values also increased at first but there was no further increase after 40 minutes. OD260 values were greater for S. aureus suspensions than E. coli. Thus, the damage to cell membranes for S. aureus was greater than for E. coli, which agreed with the findings for bactericidal activity. Jeon et alCitation28 found that when both E. coli and S. aureus were treated with dimethylaminoethyl-chitosan, the release of 260 nm-absorbing materials quickly increased, but due to the difference in cell structures, the absorbance values for E. coli and S. aureus were different.Citation28
Figure 6 Release of cell materials absorbing at 260 nm (A) and 280 (B) from E. coli or S. aureus suspensions treated with FSCP/COS NFMs, and PVA NFM treatment as a control.
Abbreviations: COS, chito-oligosaccharides; FSCP, fish scale collagen peptides; NFMs, nanofibrous membranes; PVA, polyvinyl alcohol.
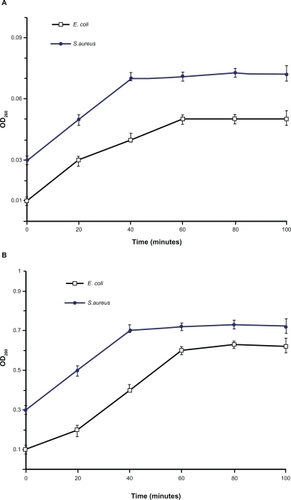
The release of intracellular proteins from both E. coli and S. aureus treated with FSCP/COS NFMs is shown in . When NFM was mixed with the bacterial suspension, the initial absorption at 280 nm markedly increased. OD280 values increased up to 40 minutes for S. aureus, and 60 minutes for E. coli, respectively, but little change was found in OD thereafter, suggesting completion of leakage. Composite NFMs containing COS appeared to be more effective for S. aureus, which was also consistent with the results of the antimicrobial activity assays.
Fibroblast cell viability
The morphology of human skin fibroblast cells cultured with various NFMs for 1 and 7 days was examined. After culture for 1 day, the fibroblasts treated with COS, FSCP, and FSCP/COS NFMs had tended to expand completely, and clear tentacles were found in many fibroblast cells (–). However, fibroblasts treated with PVA still kept the long spindle (). After 7 days, the number of fibroblasts in cultures treated with PVA NFMs seemed to be fewer than with the other three NFM treatments, and it was observed that FSCP/COS NFMs were very beneficial for the growth of fibroblasts (). Fibroblast cell proliferation was tested using the MTT methods with different NFMs. MTT absorbance for fibroblast cells grown in culture treated with PVA NFMs was significantly less than with the other three groups (). The results showed that PVA NFMs had little effect on fibroblast photomicrographs (). COS and FSCP treatments both showed good in vitro biocompatibility and supported proliferation of fibroblasts. FSCP combined with COS effectively enhanced the proliferation of fibroblasts (). The MTT absorbance of FSCP, COS, and FSCP combined with COS treatments were similar to those of the negative control (fresh culture medium) and significantly different from those of the positive control (PVA) throughout the testing period. Chitin and chitin derivatives could accelerate the development of tensile strength in wounds by speeding fibroblastic synthesis of collagen in the first few days of wound healing.Citation29 Carboxymethyl-chitosan, a water-soluble chitin derivative, was reported to stimulate the extracellular lysozyme activity of skin fibroblasts, and doubled the number of skin fibroblasts while inhibiting the proliferation of keloid fibroblast cells.Citation30 In this study COS could accelerate proliferation of normal human skin fibroblast cells. FSCP combined with COS in NFMs showed in vitro biocompatibility and may have the potential to be used as a functional biomaterial for wound dressing.
Figure 7 Photomicrographs of human skin fibroblasts after growing for 1 day and 7 days, respectively, with treatments of PVA (A, B), COS (C, D), FSCP (E, F), and FSCP/COS (G, H).
Abbreviations: COS, chito-oligosaccharides; FSCP, fish scale collagen peptides; NFM, nanofibrous membranes; PVA, polyvinyl alcohol.
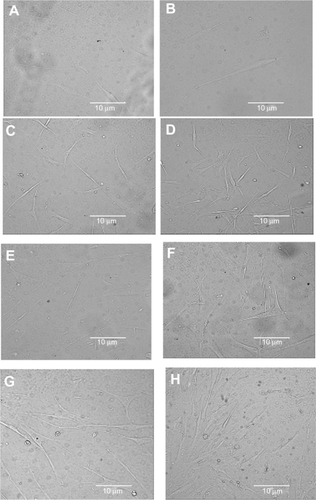
Figure 8 Fibroblast cell viability using the MTT assays. Fresh medium was used as a negative control and PVA as a positive control. The absorbance was normalized against the negative control at each time interval, which is taken as 100%.
Note: *P < 0.05 compared with the negative control.
Abbreviations: COS, chito-oligosaccharides; FSCP, fish scale collagen peptides; MTT, 3-(4,5-Dimethylthiazol-2-yl)-2,5-diphenyltetrazolium bromide; NFMs, nanofibrous membranes; PVA, polyvinyl alcohol.
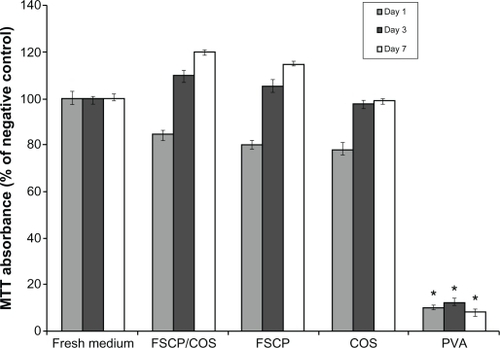
Conclusion
FSCP from fish scale collagen are superior to mammalian collagen, and are potential ingredients in wound dressing materials. To permit electrospinning of low MW FSCP and COS, PVA was added to enhance fiber formation. The antimicrobial activity of FSCP/COS NFMs is important for wound dressings. Studies using a representative Gram-positive bacterium, S. aureus, and a Gram-negative bacterium, E. coli, showed that the NFMs had a high bactericidal activity which was increased by COS content. Furthermore, the FSCP/COS NFMs had higher antimicrobial activity against S. aureus than against E. coli. The effects of FSCP/COS NFMs on bacterial adhesion suggested that bacteria adhere to the surfaces in different ways. In addition, COS with an average MW of 8 kDa could accelerate normal human skin fibroblast proliferation. Thus, composite electrospun nanomembranes with FSCP/COS have good potential to be used as functional biomaterials for wound dressings.
Acknowledgements
This work was supported by the National Natural Science Foundation of China (No. 20976138 and No. 20576002), the Natural Science Foundation of Shanghai (No. 09ZR1434500), the Ministry of Agriculture of China (No. 2009ZX08009-37B), and the China Postdoctoral Science Foundation (No. 20100470732). We thank Professor Joe M Regenstein for our English language copy editing, Dr Dongdong Lu for his technical support and kind help, and Ting Mo for her experimental help.
Disclosure
The authors declare that there are no conflicts of interest.
References
- KubikTBoguniaKKSugisakaMNanotechnology on duty in medical applicationsCurr Pharm Biotechnol20056173315727553
- BognitzkiMCzadoWFreseTNanostructured fiber via electrospinningAdv Mater2001137072
- RenekerDHChunINanometre diameter fibres of polymer, produced by electrospinningNanotechnology19967216223
- FongHChunIRenekerDHFongHChunIRenekerDHBeaded nanofibers formed during electrospinningPolymer19994045854592
- HuangZMZhangYZKotakiMA review on polymer nanofibers by electrospinning and their applications in nanocompositesCompos Sci Technol20036322232253
- YoshimotoHShinYMTeraiHVacantiJPA biodegradable nanofiber scaffold by electrospinning and its potential for bone tissue engineeringBiomaterials2003242077208212628828
- LarsenGVelarde-OrtizRMinchowKBarreroALoscertalesIGA method for making inorganic and hybrid (organic/inorganic) fibers and vesicles with diameters in the submicrometer and micrometer range via sol-gel chemistry and electrically forced liquid jetsJ Am Chem Soc20031251154115512553802
- YangLFitiéCFCWerfKOBenninkMLDijkstraaPJFeijenJMechanical properties of single electrospun collagen type I fibersBiomaterials20082995596218082253
- RujitanarojPPimphaNSupapholPWound-dressing materials with antibacterial activity from electrospun gelatin fiber mats containing silver nanoparticlesPolymer20084947234732
- HerbertGReinhardSGelatine Handbook: Theory and Industrial PracticeWeinheim, GermanyWiley2007
- EckhartHWernerRSynthesis and investigation of collagen model peptidesAdv Polym Sci198243143203
- TanakaMKoyamaYINomuraYEffects of collagen peptide ingestion on UV-B-induced skin damageBiosci Biotech Bioch200973930932
- KimSKKimYTByunHGNamKSJooDSShahidiFIsolation and characterization of antioxidative peptides from gelatin hydrolysate of Alaska pollack skinJ Agric Food Chem2001491984198911308357
- HassanCMPeppasNAStructure and applications of poly(vinyl alcohol) hydrogels produced by conventional crosslinking or by freezing/thawing methodsAdv Polym Sci20001533765
- OngSYWuJMoochhalaSMTanMHLuJDevelopment of a chitosan-based wound dressing with improved hemostatic and antimicrobial propertiesBiomaterials2008294323433218708251
- LiuSJKauYCChouCYChenJKWuRCYeheWLElectrospun PLGA/collagen nanofibrous membrane as early-stage wound dressingJ Membrane Sci20103555359
- MurakamiKAokiHNakamuraSHydrogel blends of chitin/chitosan, fucoidan and alginate as healing-impaired wound dressingsBiomaterials201031839019775748
- MinSKLeeSCHongSDChungCPParkWHMinBMThe effect of a laminin-5-derived peptide coated onto chitin microfibers on re-epithelialization in early-stage wound healingBiomaterials2010174725473020303583
- ChenCZCooperSLInteractions between dendrimer biocides and bacterial membranesBiomaterials2002233359336812099278
- NatthanCPraneetOTheerasakRTanasaitNPittSPreparation and characterization of chitosan-hydroxybenzotriazole/polyvinyl alcohol blend nanofibers by the electrospinning techniqueCarbohydr Polym201081675680
- ChenCZMaoJWWuQYPreparation and conductivity of polyvinyl alcohol (PVA) films composited with molybdotungstovanadogermanic heteropoly acidMater Chem Phys200485416419
- TeoWERamakrishnaSA review on electrospinning design and nanofibre assembliesNanotechnology20061789106
- KimTGParkTGBiomimicking extracellular matrix: cell adhesive RGD peptide modified electrospun poly (D, L-lactic–co-glycolic acid) nanofiber meshTissue Eng20061222123316548681
- ProftTBakerENPili in Gram-negative and Gram-positive bacteria structure, assembly and their role in diseaseCell Mol Life Sci20096661363518953686
- CostertonJWIntroduction to biofilmInt J Antimicrob Agents19991121722110394973
- Hall-StoodleyLCostertonJWStoodleyPBacterial biofilms: from the natural environment to infectious diseasesNat Rev Microbiol200429510815040259
- BolesBRHorswillARAgr-mediated dispersal of Staphylococcus aureus biofilmsPLoS Pathog20084113
- JeonYJParkPJKimSKAntimicrobial effect of chitooligosaccharides produced by bioreactorCarbohydr Polym2001447176
- ChungLYSchmidtRJHamlynPFSagarBFAndrewsAMTurnerTDBiocompatibility of potential wound management products: fungal mycelia as a source of chitin/chitosan and their effect on the proliferation of human F1000 fibroblasts in cultureJ Biomed Mater Res1994284634698006051
- ChenXGWangZLiuWSParkHJThe effect of carboxymethyl-chitosan on proliferation and collagen secretion of normal and keloid skin fibroblastsBiomaterials2002234609461412322982