Abstract
Nanotechnology is an exciting field of investigation for the development of new treatments for many human diseases. However, it is necessary to assess the biocompatibility of nanoparticles in vitro and in vivo before considering clinical applications. Our characterization of polyol-produced maghemite γ-Fe2O3 nanoparticles showed high structural quality. The particles showed a homogeneous spherical size around 10 nm and could form aggregates depending on the dispersion conditions. Such nanoparticles were efficiently taken up in vitro by human endothelial cells, which represent the first biological barrier to nanoparticles in vivo. However, γ-Fe2O3 can cause cell death within 24 hours of exposure, most likely through oxidative stress. Further in vivo exploration suggests that although γ-Fe2O3 nanoparticles are rapidly cleared through the urine, they can lead to toxicity in the liver, kidneys and lungs, while the brain and heart remain unaffected. In conclusion, γ-Fe2O3 could exhibit harmful properties and therefore surface coating, cellular targeting, and local exposure should be considered before developing clinical applications.
Introduction
Nanomaterial structures with dimensions between 1 and 100 nm are currently used for medical applications; such as magnetic drug targeting, hyperthermia, and enhanced resolution magnetic resonance imaging.Citation1 Nanomedicine formulations aim at improving distribution and accumulation at the target sites.Citation2 Hence, nanomaterials could improve the therapeutic index of low molecular weight drugs and provide more effective and less toxic treatment.Citation3,Citation4 However, levels of inorganic nanoparticles (NP) have already increased in ambient air and have impacted public health.Citation1,Citation2,Citation5
Previous studies revealed that NP, such as iron oxide NP, can enter into cells and accumulate in mitochondria, vesicles, phagosomes and lysosomes.Citation6–Citation8 It has also been shown that coating of NP can efficiently improve internalization in many cell types.Citation9 Toxic effects caused by iron oxide NP exposure remain controversial. Although the mechanism underlying NP-induced cytotoxicity is not completely understood, it could be related to inflammatory response and oxidative stress.Citation6,Citation10,Citation11 While it has been shown that NP do not induce inflammatory reaction in vascular endothelial cells in vitro, they can significantly decrease cancer cell viability.Citation7,Citation8,Citation12 NP activity and toxicity depend not only on their chemical and structural properties but also on their size and surface properties.Citation13,Citation14 For instance, cell viability is enhanced by particle hydrodynamic size.Citation15 However, little is known about the mechanisms involved in the potential adverse effects of NP, and their clinical development requires further evaluation of their biocompatibility. In this general context, we examined the cytotoxic effects of uncoated chemically-engineered iron oxide NP.
Material and methods
Iron oxide NP synthesis, characterization and labeling
Maghemite γ-Fe2O3 NP were engineered by a two-step method: magnetite Fe3O4 particles were first prepared by the polyol method using iron acetate salt as the precursor and diethyleneglycol as the solvent.Citation16 Hot water was used to wash out the adsorbed organic residues on the NP surfaces and oxidize them to the maghemite form. The structure, size and shape of the particles produced were investigated by X-ray diffraction (PANalytical X’PertPro; PANalytical, Almelo, the Netherlands) and transmission electron microscopy (JEOL 100-CX II, 100 kV; JEOL, Tokyo, Japan). Their hydrodynamic mean size and surface charge were determined by a dynamic light scattering method (633 nm; Malvern Zetasizer; Nano, Malvern, UK) in deionized water, MgCl2- and CaCl2-free phosphate saline buffer (PBS) pH 7.2, Dulbecco’s Modified Eagle Medium (DMEM; Invitrogen, Carlsbad, CA) and NaCl 0.9% solution. γ-Fe2O3 NP were functionalized with 3,4-dihydroxyphenylethylamine hydrochloride where the amine function serves to attach fluorescein isothiocyanate (FITC) chromophore. The functionalized γ-Fe2O3 NP (1 mg/mL in PBS) was mixed with 10 mg/mL of FITC dissolved in NaHCO3/Na2CO3 1 M overnight. Labeled NP were eluted with PBS and filtered through a 0.22 μm membrane. Labeling did not affect γ-Fe2O3 NP properties, as estimated by dynamic light scattering.
Cell culture
Immortalized human umbilical vascular endothelial cells (HUVEC, Ea.hy 926 cloneCitation17) were maintained in DMEM plus 10% fetal bovine serum and 1% penicillin/streptomycin (Invitrogen).
Confocal and electron microscopy
Cells were seeded on glass coverslips 24 hours prior to treatment with 10 μg/mL of FITC-labeled γ-Fe2O3 NP for indicated times. Fixed samples (4% paraformaldehyde/PBS, 15 minutes) were mounted in 4,6-diaminidino-2-phenylindole (DAPI)-containing mounting medium (Vector Laboratories, Burlingame, CA). Images were captured using a Leica SP2 confocal microscope (Leica Microsystems GmbH, Wetzlar, Germany; Imagery facility, Institut Cochin).
Cells were seeded on glass coverslips 24 hours prior to treatment with 10 μg/mL of γ-Fe2O3 NP for indicated times, then prepared for electron microscopy (3% glutaraldehyde/PBS, 1 hour). After incubation in 1% OsO4, cells were dehydrated in graded dilutions of ethanol, embedded in artificial resin (Epon; Momentive Specialty Chemicals, Colombus, OH) and processed for electron microscopy. Subcellular localization of NP was monitored by transmission electron microscopy (EM10CR; Zeiss, Oberkochen, Germany; Imagery facility, Institut Cochin) performed at 60–80 kV on unstained thin sections.
Western blot
Proteins were collected in TNT buffer (10 mM Tris-HCl pH 7.5, 150 mM NaCl, 1% Triton X100, 2 mM EDTA) plus protease inhibitors (Sigma-Aldrich, St Louis, MO), 200 mM NaF and 0.1 mM Na3VO4. Equal amounts of proteins (Pierce microBCA kit; Thermo Scientific, Pittsburg, PA) were separated with 4%–12% Nupage gels (Invitrogen) and transferred onto polyvinylidene fluoride (PVDF) membranes (Thermo Scientific). Primary anti-phospho-Akt, Akt, phospho-eNOS (endothelial nitric oxide synthase), eNOS, and Caspase 3 antibodies were used (Cell Signaling Technology, Beverly, MA). Alexa 680-conjugated secondary antibodies (Invitrogen) were used and membranes were scanned using the Odyssey infrared imaging system (Li-Cor Biosciences GmbH, Bad Homburg vor der Höhe, Germany).
Cell viability, toxicity and intracellular reactive oxygen species assays
Five thousand HUVEC were cultured overnight onto 96-well plates. After sonication in serum-free DMEM, freshly produced γ-Fe2O3 NP were added at increasing concentrations at the indicated times. Cell viability was assessed using the 3-(4,5-dimethylthiazol-2-yl)diphenyltetrazolium bromide (MTT) method according to manufacturer’s instructions (Sigma-Aldrich) as previously reported.Citation18 Cell viability was calculated as a ratio between treated (sample) and untreated (control).
Toxicity was measured using the lactate dehydrogenase (LDH) detection kit according to manufacturer’s instructions (Roche, Basel, Switzerland) on cell culture supernatant and cell lysates recovered in 2% Triton-X100. Optical density (OD) was measured at 492 nm with a standard microplate reader (Multiskan Ex; Thermo Scientific). LDH leakage was calculated using the following equation: [Supernatant OD/(Supernatant OD + Lysate OD) × 100].
Forty thousand HUVEC were cultured in 96-well plate format. At designated times, cells were washed and incubated with Hank’s Buffered Salt Solution (HBSS) containing 2′,7′-dichlorofluorescin diacetate (DCFH-DA, 20μM, Sigma) for 30 minutes. Fluorescence was read at 485 nm Ex/530 nm Em (Fusion). Values were normalized to their respective controls.
In vivo studies
Rats were cared for under the Tunisian Code of Practice for the Care and Use of Animals for Scientific purposes, and experimental protocols were approved by the Ethics Committee of the Faculte des Sciences de Bizerte (Tunisia). Wistar rats weighing 100–150 g (Institut Pasteur, Tunisia) were randomly divided into experimental and control groups. Rats received either γ-Fe2O3 NP (0.8 mg/kg) by single intravenous injection for the experimental group or saline solution (NaCl 0.9%) for the control group. Animal weight was monitored during the course of the experiment. Two weeks later, rats were sacrificed and blood samples and organs were collected. For histological analysis, 4 μm-thick sections of paraffin-embedded organs were stained with hematoxylin and eosin. A sample of 0.5 mL of blood was collected from each animal at the end of the experiment. White and red blood cells were counted (Medonic Blood Cell Analyzer CA 620; Medonic, Walpole, MA).
For clearance analysis, 0.3 mg/mL FITC-labeled γ-Fe2O3 NP was injected into the tail vein. Blood and urine were collected at the indicated times. Fluorescence was quantified by fluorimeter (VARIAN Cary Eclipse, Ex485 nm/Em500–590 nm; Agilent Technologies, Palo Alto, CA) and flow cytometry (FACS Coulter Epics XL, EXPO32 software; Applied Cytometry Systems, Sacramento, CA).
Statistical analysis
All experiments were carried out in triplicate and six animals were used per group. Data were analyzed using one-way analysis of variance (ANOVA) followed by unpaired Student’s t-test (Prism, GraphPad Software, Inc, La Jolla, CA). Values are expressed as mean ± standard deviation (SD). P-values of <0.05 were considered statistically significant.
Results
Iron oxide NP characterization
The powder recovered by centrifugation corresponds to the γ-Fe2O4 phase which crystallizes in the cubic spinel structure () with a cell parameter (a = 8.36 Å) close to that of bulk maghemite (a = 8.3515 Å).Citation20 Potentiometric titration, using potassium permanganate solution, after dissolution of an appropriate amount of powder in concentrated sulfuric acid, established that the iron species are exclusively ferric ions, in agreement with the formation of the maghemite phase. To determine the morphology and the mean size of the as-produced maghemite particles, low- and high-resolution transmission electron microscopy was performed. Pictures show that γ-Fe2O3 NP were mainly uniform in size with a 10 nm ± 20% diameter on average and with a spherical shape (). These results are in agreement with the average crystal size inferred from X-ray diffraction line broadening using the Scherrer formula.Citation16,Citation18 There is no evidence of physical defects such as dislocation and stacking, or of amorphous regions at the surface, suggesting high crystalline quality in single particles.
Figure 1 Nanoparticles exhibit spherical shapes. A) Polyol-made iron oxide powder consists of maghemite nanocrystals as shown by their broadened X-ray diffraction pattern. B) Fe2O3 iron oxide nanoparticle morphology was observed by transmission electron microscopy on a collection of as-produced particles (left image). A zoom on one representative particle is shown (right panel).
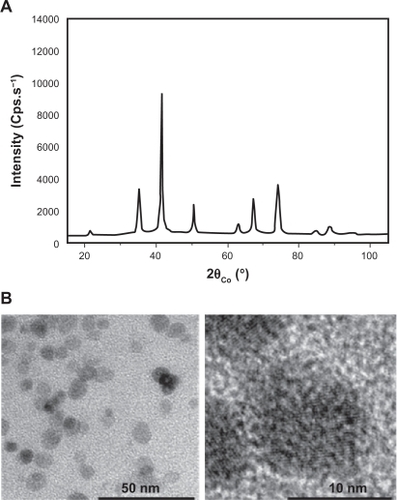
Hydrodynamic diameter and zeta potential dispersed in distilled water, PBS, DMEM and saline solution after ultrasonication are given in . The measured hydrodynamic sizes appeared larger than when evaluated by electron microscopy, probably because magnetic dipole-dipole interactions between 10 nm sized crystals can provoke γ-Fe2O3 NP aggregation. This aggregation can reach micrometer-size ranges in water, where surface charges are positive. In contrast, they are negative in PBS, NaCl 0.9% and DMEM. These physicochemical properties might help to explain the potential interactions between γ-Fe2O3 NP and the biological environment.
Table 1 Physical properties of iron oxide nanoparticle
Internalization of iron oxide NP
The endothelium may represent the first biological barrier to NP when used in vivo. We initially examined the interactions between human endothelial cells and γ-Fe2O3 NP by confocal microscopy. FITC-labelled γ-Fe2O3 NP were incubated with HUVEC monolayers. This analysis clearly showed that γ-Fe2O3 NP could be internalized in HUVEC and most of the fluorescence borne by γ-Fe2O3 NP seems to localize within the cytoplasm (). Electron microscopy showed that γ-Fe2O3 NP are found outside the cell periphery in the short term, but can efficiently reach internal structures within a few hours (). Interestingly, aggregate features of γ-Fe2O3 NP were observed both freely diffused in the cytoplasm and trapped in single membrane organelles (). They were however not detected in the nucleus even after 48 hours of incubation. Interestingly, γ-Fe2O3 NP could penetrate cells without any protein-coated vesicles, suggesting a micropi-nocytotic process.
Figure 2 Nanoparticle internalization in human endothelial cells. A and B) Human umbilical vein endothelial cells (HUVEC) were incubated with FITC-labelled nanoparticle (NP, 10 μg/mL, green) for the indicated times in serum-free medium. Nuclei were counterstained with 4,6-diamidino-2-phenylindole (DAPI) (blue) and samples were analyzed by confocal microscopy.
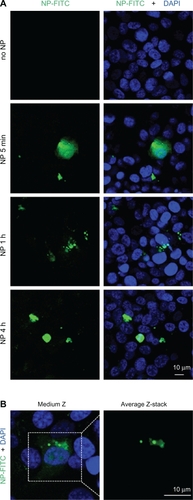
Iron oxide NP alter endothelial viability
In order to assess biocompatibility, we investigated whether γ-Fe2O3 NP could alter the endothelial biology once they had entered the cells. We performed MTT assays that measured the mitochondrial activity and therefore reflected the number of viable cells. We found that γ-Fe2O3 NP had no significant effect on HUVEC viability up to 24 hours after exposure, but a severe drop-off was revealed after 48 hours and persisted at 72 hours (). Strikingly, no dose effects were detected, even at concentrations as low as 0.1 mg/mL. We checked these results by measuring the cell toxicity of γ-Fe2O3 NP. Cell death was monitored by the so-called LDH leakage assay. This stable cytosolic enzyme can leak into the extracellular fluid after membrane damage occurring during necrosis. Toxicity markedly increased after 24 hours of contact with γ-Fe2O3 NP (), corroborating the results obtained with MTT. Furthermore, phosphorylation of Akt, a pro-survival protein was decreased on γ-Fe2O3 NP exposure, while caspase 3 cleavage, a sign of apoptosis, was not observed (). Hence, our results suggest that γ-Fe2O3 NP can induce long-term toxicity in human endothelial cells by a necrotic process. Interestingly, endothelial nitric oxide synthase (eNOS) seems to be transiently phosphorylated in cells exposed to γ-Fe2O3 NP (). To further evaluate whether eNOS activation was accompanied by an increase in oxidative stress, reactive oxygen species (ROS) production was monitored. As shown in , relative ROS quantity was slightly but significantly enhanced in γ-Fe2O3 NP-stimulated HUVEC after 24 hours. Thus, cell death and ROS production both peak at 24 hours postexposure to γ-Fe2O3 NP. Our results suggest that γ-Fe2O3 NP can promote cell death after 24 hours of exposure, most likely through oxidative stress and necrosis mechanisms.
Figure 4 Effect of nanoparticles on endothelial cell viability. A) The number of viable cells was measured by 3-(4,5-dimethylthiazol-2-yl) diphenyltetrazolium bromide (MTT) when exposed to nanoparticles (NP) at the indicated times and concentrations. Results were expressed as a ratio to nonstimulated serum-free cultured cells for each time point. B) Cell toxicity was evaluated by LDH leakage and calculated as described in the method section, in human umbilical vein endothelial cells (HUVEC) exposed to 10 μg/mL of NP. C) Cells exposed to 10 μg/mL of NP were harvested for Western blot analysis at the indicated time points. Phosphorylation (p) of Akt and endothelial nitric oxide synthase (eNOS) was monitored, along with Akt, eNOS and Caspase 3. D) Reactive oxygen species (ROS) production was examined in NP (10 μg/mL)-exposed HUVEC at the indicated time points. Fluorescence values were normalized to the ones obtained in control conditions.
Notes: All results are representative of three independent experiments, **P < 0.01; *P < 0.05.

In vivo toxicity of iron oxide NP
Because in vitro toxicity was observed after 24 hours, we decided to explore the effects of γ-Fe2O3 NP exposure in vivo. Clearance of injected FITC-labeled γ-Fe2O3 NP was estimated, compared to background fluorescence in controls (). Blood analysis showed that 40% of injected γ-Fe2O3 NP were removed from systemic circulation after 24 hours and that 75% were eliminated after 72 hours (). A large quantity was cleared in the urine (). Animal weight was monitored during the course of the experiments. The graph presented in illustrates the fact that γ-Fe2O3 NP did not alter the general health of the animals. In addition, no signs of distress, such as loss of hair or behavioral changes were observed. However, the number of white blood cells was augmented by about 50% in γ-Fe2O3 NP-injected animals, while red blood cell counts remained unaffected (). This prompted us to investigate the histology of the main organs for any sign of immunological reactions. Inflammation as indicated by eosin-positive infiltrated cells was detected in lungs, liver and kidney (, squares). Heart and brain structures do not appear to be modified in NP-injected animals.
Figure 5 In vivo clearance and toxicity of nanoparticles. Six animals for each groups received saline (Sham) or nanoparticle (NP) (0.8 mg/kg NP) by intravenous injection. A and B) Blood and urine clearance profile of fluorescein isothiocyanate (FITC)-labeled NP was measured either by flow cytometry or spectrophotometry, respectively, after 24 and 72 hours. C) Animals were weighed during the course of control and NP treatment. D) Numbers of white and red blood cells (WBC, RBC) were examined in animals treated with control and NP for 14 days. E) Organs were collected 14 days post-treatment and processed for staining with hematoxylin and eosin.
Notes: **P < 0.01; *P < 0.05.

We concluded that γ-Fe2O3 NP are efficiently taken up by endothelial cells where they produce toxic effects after 24 hours. When injected in vivo, such NP can promote cellular damage in the liver, kidney and lungs, although they are rapidly cleared. Clinical applications require improvement of NP biocompatibility.
Discussion
Our work was designed to determine whether 10 nm-sized iron oxide NP (γ-Fe2O3 NP) are biocompatible with clinical use, especially local hyperthermia application in solid tumors. We first aimed at understanding whether γ-Fe2O3 NP could penetrate the endothelium without causing any potential toxicity, using in vitro human endothelial cells (HUVEC) and an in vivo rat model. We found that γ-Fe2O3 NP are internalized in HUVEC quite efficiently within a few hours, but can provoke cell death 24 hours postexposure, most likely through the oxidative stress pathway. Although γ-Fe2O3 NP are eliminated through urine when injected in vivo, they could induce toxicity in some organs while sparing the brain and heart. Our study suggests that γ-Fe2O3 NP could be used in vivo for very short times but they may need further improvement to reduce their toxicity and to increase their targeted delivery.
Particles used here correspond to the maghemite phase and are free from any coating. DLS measurements have shown that the particles tend to aggregate when dispersed in hydrophilic solutions. This was also confirmed by electron microscopy analysis. This property may affect their biological action; for example, increased toxicity was correlated with carbon nanotube aggregation.Citation19 In addition, it has been suggested that cellular uptake may be modulated by aggregation state.Citation3 Experiments demonstrated that γ-Fe2O3 NP are internalized either in the cytosol or in membrane-delimited vesicles.
Evaluation of cell toxicity by different means suggest a long-term toxic effect of γ-Fe2O3 NP in human endothelial cells. No dose-dependent action of γ-Fe2O3 NP was detected. Our results point to a correlation between exposure time and cell toxicity. Molecular mechanisms involved in γ-Fe2O3 NP-mediated cell death may rely on oxidative stress occurring after 24 hours. NP-decreased cell viability kinetics are significantly slower than canonical death inducers, such as staurosporine. The mechanism involved here is rather complex, as it requires NP entrance and interference with oxidative stress pathways.
To examine in vivo effects, γ-Fe2O3 NP were administered by single intravenous injection to allow maximum bioavailability. Their persistence and their proportional distribution depend on their size.Citation3 Aggregation of particles, evidenced by DLS measurement in saline solution, may decrease when in the blood stream, because of its molecular and cellular composition and viscosity, suggesting that smaller clusters could form and cross the endothelial barrier. This was confirmed by in vitro experiments. Iron oxide NP led to cytopathological effects in lungs, liver and kidneys following systemic administration. The perivascular inflammation indicates that γ-Fe2O3 NP could reach several organs such as the lungs and kidneys. Correlating with this, we also found an increase in white blood cell numbers, suggesting an inflammatory process. In contrast, the red blood cell number remained unchanged, indicating low toxicity of these particles towards erythrocytes. The brain may be protected by the blood–brain barrier, which is selective to particles larger than 1 nm. We did not observe any toxicity of γ-Fe2O3 NP when applied in vitro to human brain endothelial cells. Absorption of γ-Fe2O3 NP in lungs, liver and kidneys may be most likely related to their specific vascularization and vessel permeability. Our data suggest that despite rapid clearance, polyol-made maghemite 10 nm diameter particles induced in vitro and in vivo cytotoxicity after prolonged exposure. γ-Fe2O3 NP could induce adverse effects and therefore surface coating, cellular targeting, and local exposure should be considered before clinical applications are developed.
Acknowledgements
The authors are indebted to Dr F Herbst and Dr M Hamadi for their assistance in electron microscopy analysis and chemical NP surface modification. This research was supported by a grant from Ligue Nationale contre le Cancer, comite de Paris, the CMCU France-Tunisia Cooperation Research Program and Association pour la Recherche sur le Cancer. AH is supported by a PhD fellowship from the Ministere des Affaires Etrangeres, Bourse d’Excellence Eiffel.
Disclosure
The authors report no conflicts of interest in this work.
References
- MaynardADAitkenRJButzTSafe handling of nanotechnologyNature200644426726917108940
- LanoneSBoczkowskiJBiomedical applications and potential health risks of nanomaterials: molecular mechanismsCurr Mol Med2006665166317022735
- HallJBDobrovolskaiaMAPatriAKMcNeilSECharacterization of nanoparticles for therapeuticsNanomedicine (Lond)2007278980318095846
- LammersTKiesslingFHenninkWEStormGNanotheranostics and image-guided drug delivery: Current concepts and future directionsMol Pharm201071899191220822168
- ColvinVLThe potential environmental impact of engineered nanomaterialsNat Biotechnol2003211166117014520401
- XiaTKovochichMBrantJComparison of the abilities of ambient and manufactured nanoparticles to induce cellular toxicity according to an oxidative stress paradigmNano Lett200661794180716895376
- GojovaAGuoBKotaRSRutledgeJCKennedyIMBarakatAIInduction of inflammation in vascular endothelial cells by metal oxide nanoparticles: effect of particle compositionEnviron Health Perspect200711540340917431490
- KirchnerCLiedlTKuderaSCytotoxicity of colloidal CdSe and CdSe/ZnS nanoparticlesNano Lett2005533133815794621
- BhirdeAAPatelVGavardJTargeted killing of cancer cells in vivo and in vitro with EGF-directed carbon nanotube-based drug deliveryACS Nano2009330731619236065
- HussainSMHessKLGearhartJMGeissKTSchlagerJJIn vitro toxicity of nanoparticles in BRL 3A rat liver cellsToxicol In Vitro20051997598316125895
- Braydich-StolleHussainSSchlagerJJHofmannMCIn vitro cytotoxicity of nanoparticles in mammalian germline stem cellsToxicol Sci20058841241916014736
- DobrovolskaiaMAMcNeilSEImmunological properties of engineered nanomaterialsNat Nanotechnol2007246947818654343
- WarheitDBWebbTRReedKLFrerichsSSayesCMPulmonary toxicity study in rats with three forms of ultrafine-TiO2 particles: differential responses related to surface propertiesToxicology20072309010417196727
- NelAXiaTMadlerLLiNToxic potential of materials at the nanolevelScience200631162262716456071
- MahmoudiMSimchiAImaniMMilaniASStroevePAn in vitro study of bare and poly(ethylene glycol)-co-fumarate-coated super-paramagnetic iron oxide nanoparticles: a new toxicity identification procedureNanotechnology20092022510419433870
- BastiHBen TaharLSmiriLSCatechol derivatives-coated Fe3O4 and gamma-Fe2O3 nanoparticles as potential MRI contrast agentsJ Colloid Interface Sci201034124825419853857
- EdgellCJMcDonaldCCGrahamJBPermanent cell line expressing human factor VIII-related antigen established by hybridizationProc Natl Acad Sci U S A198380373437376407019
- BejiZHaniniASmiriLSMagnetic properties of Zn-substituted MnFe2O4 nanoparticles synthesized in polyol as potential heating agents for hyperthermia. Evaluation of their toxicity on Endothelial cellsChem Mater20102254205429
- DingMKisinERZhaoJSize-dependent effects of tungsten carbide-cobalt particles on oxygen radical production and activation of cell signaling pathways in murine epidermal cellsToxicol Appl Pharmacol200924126026819747498
- CornellRMSchwertmannUThe iron oxides: structures, properties, reactions, occurrence and usesNew YorkVHC1996