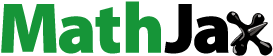
Abstract
This study deals with the preparation and investigation of a nanoscale delivery system for the anticancer drug doxorubicin (DOX) using its complexation with polyanionic carbohydrate dextran sulfate (DS). Dynamic light scattering, SEM, and zeta potential determination were used to characterize nanocomplexes. DOX-DS complexation was studied in the presence of ethanol as a hydrogen-bond disrupting agent, NaCl as an electrostatic shielding agent, and chitosan as a positively charged polymer. Thermodynamics of DOX-DS interaction was studied using isothermal titration calorimetry (ITC). A dialysis method was applied to investigate the release profile of DOX from DOX-DS nanocomplexes. Spherical and smooth-surfaced DOX-DS nanocomplexes (250–500 nm) with negative zeta potential were formed at a DS/DOX (w/w) ratio of 0.4–0.6, with over 90% drug encapsulation efficiency. DOX when complexed with DS showed lower fluorescence emission and 480 nm absorbance plus a 15 nm bathometric shift in its visible absorbance spectrum. Electrostatic hydrogen bonding and π-π stacking interactions are the main contributing interactions in DOX-DS complexation. Thermal analysis of DOX-DS complexation by ITC revealed that each DOX molecule binds with 3 DS glycosyl monomers. Drug release profile of nanocomplexes showed a fast DOX release followed by a slow sustained release, leading to release of 32% of entrapped DOX within 15 days. DOX-DS nanocomplexes may serve as a drug delivery system with efficient drug encapsulation and also may be taken into consideration in designing DOX controlled-release systems.
Introduction
The anthracycline antibiotic doxorubicin (DOX) is a potent chemotherapeutic agent that has been used for over 30 years to treat a wide spectrum of human malignancies, especially breast cancer and lymphoma.Citation1 However, despite its efficiency and wide use, its therapeutic utility is limited due to dose-limiting toxicities such as bone marrow suppressionCitation2 and congestive heart failure.Citation3–Citation5 Another challenge met by DOX is the development of resistance mechanisms by tumor cells,Citation6–Citation8 which reduce the intracellular drug concentration either by reducing drug uptake or enhancing drug efflux.Citation9
Numerous studies have sought the solution to these problems in nanoparticulate carriers.Citation10–Citation19 Nanoscale drug carriers are intended to overcome drug resistance presumably by means of being enveloped in an endosome when entering the cell and thereby bypassing P-glycoprotein efflux pumps (one of the main drug-resistance mechanisms) and preventing drug efflux from the resistant tumors.Citation20–Citation23 One of the nano-oriented approaches undertaken to this end is the complexation of drugs with polymers.Citation16 Electrostatic interaction can result in complexation between cationic drugs and anionic polymers.Citation24,Citation25 DOX possesses positive charge and has been studied for its complexation with various anionic polymers such as poly(acrylic acid),Citation26,Citation27 γ-polyglutamic acid,Citation28 polyaspartate,Citation24,Citation29 polyglutamate,Citation30 block ionomers of aspartate, benzyl aspartate,Citation31–Citation34 benzyl glutamate,Citation29 and polyethylene oxide. These polyanions form polyelectrolyte complexes with DOX. Aromatic stacking and hydrogen bonding interactions are also assumed to play an important role in such complexes.Citation26,Citation27
Dextran sulfate (DS) is a highly anionic carbohydrate which has been investigated for improving encapsulation efficiency of DOX. Successful augmentation of DOX encapsulation into albumin microspheres was achieved with the aid of DS.Citation35 Janes et al employed DS to increase DOX loading into chitosan nanoparticles.Citation36 DOX has also been encapsulated into chitosan microparticles formulated via the complex coacervation method with DS.Citation37 In a series of studies, DOX was conjugated with polymeric dextrans of various molecular weights and it was found that the cytotoxicity, and the DNA-binding kinetics of DOX were subsequently modified.Citation38–Citation40 Antitumor activity, acute toxicity, and plasma pharmacokinetics of DOX-DS conjugate were compared with free DOX in rats following iv administration. Lam et alCitation41,Citation42 showed that the DOX-DS conjugate exerted superior antitumor activity to the free DOX in multi-drug-resistant cell lines, the reason being cited as a decrease in the removal rate of DOX from P-glycoprotein overexpressing multidrug-resistant cells once conjugated to dextran. Another group further investigated this possibility of overcoming the DOX resistance in vitro by using DOX-DS conjugate in a multidrug resistance subline of human carcinoma and found similar results.Citation43 Herein, we report the formation of electrostatic complexes at nanoscale between positively charged DOX and negatively charged DS (DOX-DS). The experimental approach includes probing the nature of interactions in DOX-DS complexation, characterization of DOX-DS nano-complexes, and release profile of drug molecules. DOX-DS interaction is also studied in the presence of the positively charged polymer chitosan.
Materials and methods
Materials
DS (D4911, MW of 8000) was obtained from Sigma Chemical Co. Doxorubicin (purity ~98.5%) was purchased from RPG Life Sciences Limited (Ankleshwar, India). All other chemicals were of analytical grade; deionized water was used throughout.
Preparation and characterization of DOX-DS nanocomplexes
DOX HCl was dissolved in deionized water at a concentration of 0.1 mg/mL. To this solution, an equal volume of DS solution in deionized water was added to give final DOX-DS solutions of 0.1, 0.2, 0.3 0.4, 0.5, 0.6, 0.8, 1, 1.5, 2, 3, and 4 w/w. Solutions were left for 45 minutes in the dark while stirring. The hydrodynamic mean diameter and zeta potential of DOX-DS nanocomplexes were measured by, respectively, dynamic light scattering and laser doppler electrophoresis using Zetasizer (Nano-ZS, Malvern, UK). All dynamic light scattering measurements were performed with a wavelength of 633 nm at 25°C with an angle detection of 90°. All measurements were run in triplicate.
Analysis of encapsulation efficiency
Encapsulation efficiency of DOX-DS nanocomplexes was analyzed by ultracentrifugation of complexes at 80,000 rpm for 30 minutes. To make sure that free drug did not precipitate at that high rotation speed, free drug solution was used as control. The concentration of remaining DOX in supernatant was measured spectroscopically at 480 nm and encapsulation efficiency was determined by applying the following equation:
Scanning electron microscopy (SEM)
The morphological characteristics of nanoparticles were observed by using an SEM (XL 30, Philips, The Netherlands). Nanoparticle suspensions were spread on an aluminium disc and allowed to dry at room temperature. The dried nanoparticles were then coated with gold metal using a sputter coater (SCD 005, Bal-Tec, Switzerland).
Spectrophotometry and fluorescence spectroscopic study of DOX-DS nanocomplexes
DOX solutions of different concentrations were mixed with DS in ratios of 0.1:1, 0.2:1, 0.3:1, 0.4:1, 0.5:1, 0.6:1, 0.7:1, 0.8:1, 1:1, 1.5:1, 2:1, 3:1, and 4:1. The absorbance of the resulting nanocomplexes was measured at 480 nm using a UV-VIS spectrophotometer (Scinco S-3100, Korea). The fluorescence measurement was carried out on a fluorescence spectrophotometer (Cary Eclipse, Varian, Australia) equipped with a thermostatically controlled cell holder at an ambient temperature. The monochromatic slits were set at 5 nm to reduce the intensity of the signal depending on the experiment. The wavelength of excitation was 470 nm and the spectra were recorded between 500 and 700 nm. Water was used as the baseline reference in both studies.
DOX-DS interaction study
To come to a deeper understanding of the nature of interactions involved, DOX-DS complexation was studied in presence of ethanol as a hydrogen-bond disrupting agent and NaCl as an electrostatic shielding agent. Ethanol was added to the DOX-DS nanocomplex solution (DOX: 51.7 μM, DS/DOX: 0.6) at different v/v proportions and the absorbance at 480 nm was measured and compared with that of pure DOX in the corresponding ethanol–water cosolvent system. The absorbance of the DOX-DS formulation was also studied in different ionic strengths, ie, different concentrations of NaCl.
In addition, the absorbance of the DOX-DS system was examined in presence of chitosan (CS) as a positively charged polymer. CS was added to DOX-DS system (DOX: 51.7 μM, DS/DOX: 0.6) at CS/DS (w/w) ratio of 0.25, 0.5, 0.75, 1, 2, and 3; the resulting 480 nm absorbance was studied.
In vitro evaluation of DOX release from nanocomplexes
In vitro drug release profile of DOX-DS nanocomplexes was investigated as follows. Nanocomplex suspension (DOX 50 μg/mL, DS/DOX 0.6) was placed into dialysis tubing (Sigma dialyses tubes Mw cutoff 12 kDa) with phosphate buffer solution (PBS) as release medium. The nanocomplex was then incubated in a shaking water bath at 37°C. At appropriate time intervals, a certain volume of the release medium was taken out and was replaced by the same volume of fresh PBS. Sampling volumes were selected to ensure sink conditions, ie, to maintain the drug concentration in dialysate below 10% of its aqueous solubility. The amount of DOX in the release medium was examined by HPLC. A solution of free DOX was also loaded into dialysis tubing and dialyzed under the same condition as control.
The cumulative fraction of released DOX was calculated according to the following equation:
where [DOX]i and Vi represent the measured DOX concentration in and volume of sample taken at time ti; [DOX]f and Vf, final measured DOX concentration in and final volume of release medium at time (tn); [DOX]0 and V0, initial DOX concentration in and volume of the nanocomplex formulation under study.
In addition, DOX release rate (%/hr) was estimated as:
where (%DOX)tn and (%DOX)tn–1 are percentage of released DOX at sampling time tn(hr) and tn–1(hr).
HPLC analysis of DOX
Released DOX in the dialysate was quantified by C18 reverse phase column connected to a Knauer HPLC system; the Knauer HPLC apparatus consisted of an S 1000 pump with fluorescence detector (RF 10 Axl). Mobile phase was composed of a mixture of 0.01 M potassium dihydrogen phosphate and acetonitrile in a ratio of 65:35 whose final pH was adjusted to 4.2 using phosphoric acid. The flow rate was set to 1 mL/minute and the fluorescence (excitation 470 nm, emission 550 nm) was recorded. All determinations were carried out at ambient temperature. Under these conditions DOX showed a retention time of about 4 minutes. DOX concentrations were calculated with reference to a calibration curve.
ITC study
The isothermal titration microcalorimetric experiments were performed with ITC instrument (VP-ITC, MicroCal, LLC, Northampton, MA). The titration vessel was made from stainless steel. DS solution (4797 μM glycosyl unit) was injected by use of a Hamilton syringe into the calorimetric titration vessel, which contained 1.8 mL DOX (862 μM). Thin (0.15 mm inner diameter) stainless steel hypodermic needles, permanently fixed to the syringe, reached directly into the calorimetric vessel. Injection of the DS solution into the perfusion vessel was repeated 20 times, with 10 μL per injection. The calorimetric signal was measured by a digital voltmeter which was part of a computerized recording system. The heat of each injection was calculated by the Origin 7.0 software program (OriginLab Corp, Northampton, MA). The microcalorimeter was frequently calibrated electrically during the course of the study.
The ITC of study was also performed with DOX and DS solved in 0.15 M aqueous solution of NaCl (ionic strength 0.15 M). Injections were carried out 20 times (15 μL each time).
Results
Characterization of nanocomplexes
As illustrated in , mean hydrodynamic diameter, polydispersity index, and zeta potential of DOX-DS nanocomplexes containing DOX at the concentration of 0.05 mg/mL and different DS/DOX ratios were measured. Among different DS/DOX ratios examined, ratios between 0.4 and 0.6 led to nanocomplexes.
Table 1 Mean hydrodynamic diameter, polydispersity index (PDI), and zeta potential of DOX-DS complexes containing DOX at the concentration of 0.05 mg/mL and different DS/DOX ratios
Complexation efficiency
The efficiency of DOX encapsulation by DOX-DS nanocomplexes was highly affected by DS/DOX ratio. DOX complexation was enhanced with increasing DS/DOX ratio until it reached a peak of 95% at a DS/DOX ratio of 0.5. Efficiency then declined with further increments in the DS/DOX ratio ().
Surface morphology of nanocomplexes
clearly shows regular smooth and spherical nanocomplexes free from aggregation.
Absorbance and florescence spectrum of DOX-DS nanocomplexes
Absorbance spectrum of DOX in water shows a maximum at 480 nm and 2 shoulders at 500 and 535 nm. Complexation with DS as depicted in caused a bathochromic shift to λmax 495 nm, and a hypochromic and broadened spectrum. No shift was observed in the florescence emission spectrum, but the intensity was lowered more drastically compared with the visible absorbance spectrum ().
The relative decrease in 480 nm absorbance of DOX-DS complexes with respect to free DOX was independent of DOX concentration and dependent on DS/DOX ratios. As shown in , absorbance decreased with further addition of DS until a plateau (A480DOX–DS/A480DOX = 0.68) was achieved at DS/DOX ratio of 0.4, after which no further decrease in the above absorbance fraction was observed.
DOX-DS interaction
To investigate the role played by hydrogen bond and electrostatic interaction in DOX-DS complexation, such interactions between DOX and DS were inhibited by the addition of ethanol and NaCl and the corresponding absorbance was measured. The results revealed that in presence of either agent, the absorbance increased; moreover, at concentrations >50% v/v ethanol or 0.3 M NaCl, the absorbance of DOX cosolved with DS was equal to that of free drug ().
Figure 4 A) Effect of ethanol concentration, B) effect of NaCl concentration, and C) effect of polycationic chitosan (CS) on absorbance of doxorubicin–dextran sulfate (DOX-DS) nanocomplexes (DOX: 60 μg/mL, DS/DOX [w/w]: 0.6).
![Figure 4 A) Effect of ethanol concentration, B) effect of NaCl concentration, and C) effect of polycationic chitosan (CS) on absorbance of doxorubicin–dextran sulfate (DOX-DS) nanocomplexes (DOX: 60 μg/mL, DS/DOX [w/w]: 0.6).](/cms/asset/72667852-b410-44e4-83d3-2461460bb3e6/dijn_a_18535_f0004_b.jpg)
Moreover, polycationic carbohydrate was demonstrated to influence DOX-DS interaction as well. shows that the presence of CS up to CS/DS (w/w) 0.5 caused a slight decrease in DOX-DS absorbance. Higher CS/DS (w/w), however, increased DOX-DS absorbance, until at CS/DS (w/w) >3, DOX-DS absorbance reached that of free drug.
In vitro drug-release studies
A dialysis method was applied to investigate the release profile of DOX from DOX-DS nanocomplexes. shows that 14% of DOX was released during the first 24 hours followed by slow release of 32% over a time span of 15 days. presents a clearer view of release behavior of DOX-DS nanocomplexes. Furthermore, a controlled release study of free DOX revealed complete diffusion of drug through dialysis membrane within 10 hours ().
Thermal analysis of DOX-DS complexation
The thermodynamics of DOX-DS interaction was studied by ITC. Analysis by Thermometric Digitam 3 () yielded a binding constant of 0.337 ± 0.00139 molDOX/molGlycosyl, an enthalpy value (ΔH) of value of −6.989 kcal/mol, and an entropy value (ΔS) of 0.011 kcal/mol°K. Free energy (ΔG) was calculated equal to −10.267 kcal/mol by putting ΔH and ΔS into the Gibbs free energy equation (ΔG = ΔH – TΔS) ().
Figure 6 Isothermal titration calorimetry curve obtained from titration of 862 μM doxorubicin (DOX) with dextran sulfate (4797 μM glycosyl units) in (A) water (B) NaCl 0.15 M.
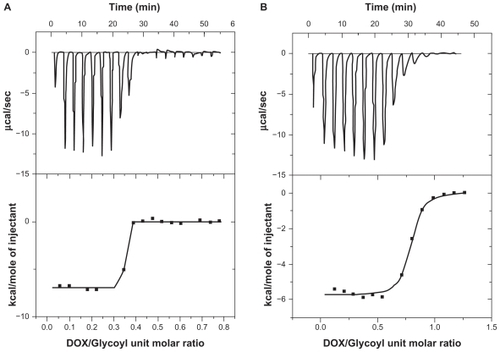
For DOX-DS interaction in the presence of NaCl 0.15 M, the thermodynamic parameters obtained were: ΔH = −5.37 kcal/mol; ΔS = 0.006 kcal/mol; ΔG = −7.158 kcal/mol (); binding constant was 0.757 ± 0.0061 molDOX/molGlycosyl.
Discussion
The main objective of this study was to study DOX-DS complexation as a potential drug carrier. DOX () is composed of an aglycone moiety to which an amino sugar daunosamine is attached via a glycosidic bond. The aglycone part is a tetracyclic chromophore adiamycinone in which the B, C, and D rings form a planar anthraquinone system. These aromatic rings result in π-π stacking properties of DOX, which allows its intercalation into the DNA double helix (one of the mechanisms of action proposed for this anticancer drug). In addition, the amino sugar of DOX has a pKa value of 8.6, which confers alkaline properties onto this molecule and positive charge at neutral pH.Citation44,Citation45 Because of the positive charge of DOX, DOX is expected to associate at a high level with polyanionic DS.
The importance of electrostatic interaction in DOX-DS complexation was established by studying the absorption spectrum under different ionic strengths, ie, different concentrations of NaCl. Na+ and Cl− shield electrostatic charge of DS and DOX, respectively, and hamper their interaction with one another. At ionic strengths >0.3 M, DOX-DS electrostatic interaction is thoroughly disturbed and the absorption spectrum is not different from that of free DOX. The result is in accordance with that of Kitaeva et al who observed preservation of florescence spectrum of DOX when binding with polyacrylic acid in the presence of NaCl.Citation26 On the other hand, despite the weakening effect of NaCl on complexation of DOX with pluronic P85-b-polyacrylic acid block copolymers, Tian et al reported no significant dissociation of DOX-pluronic P85-b-poly(acrylic acid) block copolymers.Citation28 ITC experiments also demonstrated DOX-DS interaction to be strongly salt-dependent; the enthalpy of binding decreased from ~−7.489 to ~−5.37 kcal/mol in the presence of NaCl 0.15 M. The binding constant also increased from 0.33 to 0.75 molGlycosyl/molDOX; the shielding effect of NaCl weakens the intrarepulsion of the negatively charged sulfate groups and makes the DS structure less extended. As a result, higher numbers of glycosyl units can be accommodated around each DOX molecule leading to a higher molGlycosyl/molDOX ratio.
Furthermore, addition of an H-bond disturbing agent such as ethanol to the system caused recovery of the DOX absorption spectrum. This indicates the contribution of hydrogen bonds to DOX-DX nanocomplexes. According to Tian et al, these hydrogen bonds are ascribed to substituent (HO-C-CO-CH2OH) shown by a dashed circle in ,Citation27,Citation46 which also takes part in its interaction with DNA.Citation47
Addition of DS to DOX resulted in a decrease in the optical density of DOX solution (λmax = 480 nm) along with a bathochromic shift of about 15 nm. Such alteration in DOX spectra was previously reported in the interaction of DOX with other anionic biopolymers such as DNA,Citation48 polyacrylic acid,Citation26 and γ-polyglutamic acid.Citation28 Florescence intensity of DOX was also weakened by DS complexation. However, the reduction in florescence was greater than the reduction in 480 nm absorbance.
In addition, there is a threshold for this hypochromic effect of DS. In fact, complexation of DOX with DS reduces its absorbance to 0.68 with respect to free drug at a DS/DOX ratio of 0.4; above this ratio, further addition of DS does not affect absorbance of DOX.
On the other hand, the complexation efficiency curve obtained by the ultracentrifugation method shows a peak rather than a plateau; the curve indicates a maximum association of DOX with DS at a DS/DOX ratio of 0.5. The results reveal that DOX is encapsulated in up to 95% of DOX-DS complexes up to 0.5 and then declines again at higher DS/DOX ratios. Margaritis et al also reported such high DOX complexation efficiency in DOX-γ-polyglutamic acid complexes.Citation28 This discrepancy between spectroscopy and encapsulation studies may be resolved through dynamic light scattering measurements according to which DOX-DS complexes form particles at a nanoscale level at DS/DOX ratios of 0.4 to 0.6. From all these results, the following mechanism is suggested for DOX-DS complexation: negatively charged DS interacts electrostatically with positively charged DOX molecules, and this interaction is strengthened by hydrogen-bonding interactions between hydroxyl groups of carbohydrate DS and hydroxyl groups attached to the anthraquinone ring in DOX. These interactions bring aromatic-structured DOX molecules in each other’s vicinity and facilitate π-π stacking interactions between them. In fact, DOX molecules are known to form self-associated structures at [DOX] >130 μM;Citation49 this gives rise to a change in the absorption spectrum of DOX, a phenomenon known as metachromasy.Citation50 In the presence of a polyanionic chain such as DS, this metachromatic behavior occurs at lower DOX concentrations.
As DS/DOX ratios increase up to 0.4, more DOX molecules become involved in electrostatic and hydrogen-bonding interactions with DS chains and stacking interactions with each other, and consequently DOX absorbance decreases. At a DS/DOX ratio of 0.4, DOX comes into equilibrium between bulk solution and DOX-DS complexes; DS ratio is high enough to accommodate a maximum fraction of DOX. As illustrated in , in the 0.4–0.6 range, a stacked array of DOX molecules acts as a physical crosslinker which, along with DOX-DS electrostatic interaction, makes DOX-DS complexes compact enough to form drug–polymer nanocomplexes. With further addition of DS to the system at DS/DOX > 0.6, DOX molecules are distributed among a higher number of DS chains and are positioned farther from each other; stacking interactions are weakened and the DOX-DS network is loosened. Therefore, DOX-DS complexes are not compact enough to form nanocomplexes and are of insufficient density to sediment during ultracentrifugation, which explains the descending part of the encapsulation curve. In fact, a close look at the absorption spectrum of the supernatant of ultracentrifuged DOX-DS complexes reveals the bathometric shift in DOX spectrum at DS/DOX > 0.6; this confirms the presence of DOX-DS complexes not sedimenting by ultracentrifugation.
Thermal analysis of DOX-DS complexation by ITC revealed a binding constant of 0.337 ± 0.00139 mol DOX unit per 1 mol DS glycosyl unit; in other words, each DOX molecule binds with 3 DS glycosyl monomers. The ITC curve shows a jump in ΔH of system from DOX/glycosyl unit molar ratio 0.25–0.4, equal to 0.18–0.3 DS/DOX (w/w). It seems that a DS/DOX ratio of 0.18 is a critical point where electrostatic interaction is enough to stimulate DOX-DS binding via H-bonding and then stacking interactions, and consequently results in the onset of the exothermic peak.
According to the in vitro release study, DOX-DS nanocomplexes release 14% of DOX at a fast rate during the first 24 hours. This is probably due to release of DOX molecules weakly bound with DS. However, the remaining DOX molecules are tightly complexed to DS by several electrostatic hydrogen-bonding or π-π interactions; 32% of this strongly entrapped DOX is released slowly within 2 weeks. Controlled release study of free DOX also demonstrated fast diffusion of drug into dialysate under sink conditions, which proves that diffusion through dialysis tubing (MWCO 12 kDa) is not a barrier to the passing of the drug, freed from nanocomplexes, into dialysate. Such DOX-DS nanocomplexes may be employed in designing systems for controlled release of DOX. However, in systems dealing with ionic polymers, eg, CS nanoparticles,Citation36,Citation37 the competitive effect of the second ionic polymer on DOX-DS interaction should be borne in mind. Contrary to Janes et al,Citation36 who observed only partial dissociation of DOX-DS complex in DS/DOX (w/w) 10, our results showed complete resumption of DOX absorbance at CS/DOX (w/w) > 3, indicating complete dissociation of DOX-DS complexation. The higher charge density of CS and its lower steric hindrance compared with bulky DOX molecules makes it a more favorable electrostatic partner for DS and disrupts DOX-DS complexation.
Conclusion
DOX-DS nanocomplexes may serve as a drug delivery system with efficient drug encapsulation. Complexes are in the nanoscale range at a DS/DOX (w/w) ratio of 0.4–0.6. Electrostatic hydrogen bonding and aromatic interactions play the main roles in their formation. The DOX release profile of nanocomplexes shows an initial fast release followed by a sustained release. DOX-DS interaction is affected by other anionic polymers, an important point when applying DS in order to enhance DOX encapsulation into other polymeric delivery systems.
Disclosure
The authors declare no conflicts of interest.
References
- HoskinsWJPerezCAYoungRCBarakatRRMarkmanMPrinciples and Practice of Gynecologic Oncology4th edLippincott Williams & Wilkins2005
- HrusheskyWJMBone marrow suppression from doxorubicin and cis-diamminedichloroplatinum is substantially dependent upon both circadian and circannual stages of administrationAnn N York Acad Sci1982397293295
- SwainSMWhaleyFSEwerMSCongestive heart failure in patients treated with doxorubicin: A retrospective analysis of three trialsCancer200397112869287912767102
- SchwartzRGMcKenzieWBAlexanderJCongestive heart failure and left ventricular dysfunction complicating doxorubicin therapy: Seven-year experience using serial radionuclide angiocardiographyAm J Med1987826110911183605130
- Von HoffDDLayardMWBasaPRisk factors for doxorubicin-induced congestive heart failureAnn Intern Med1979915710717496103
- ZijlstraJGDe VriesEGEMulderNHMultifactorial drug resistance in an Adriamycin-resistant human small cell lung carcinoma cell lineCancer Res1987477178017843028613
- KayeSMerrySTumour cell resistance to anthracyclines: A reviewCancer Chemother Pharmacol1985142961032982511
- NairSSinghSVSamyTSAKrishanAAnthracycline resistance in murine leukemic P388 cells. Role of drug efflux and glutathione related enzymesBiochem Pharmacol19903947237282306280
- BradleyGJurankePFLingVMechanism of multidrug resistanceBiochim Biophys Acta – Reviews on Cancer1988948187128
- PatilRRGuhagarkarSADevarajanPVEngineered nanocarriers of doxorubicin: a current updateCrit Rev Ther Drug Carrier Syst200825116118540835
- GilESLiJXiaoHLoweTLQuaternary ammonium β-cyclodextrin nanoparticles for enhancing doxorubicin permeability across the in vitro blood-brain barrierBiomacromolecules200910350551619216528
- SubediRKKangKWChoiHKPreparation and characterization of solid lipid nanoparticles loaded with doxorubicinEur J Pharm Sci2009373–450851319406231
- MissirlisDKawamuraRTirelliNHubbellJADoxorubicin encapsulation and diffusional release from stable, polymeric, hydrogel nanoparticlesEur J Pharm Sci200629212012916904301
- ParkJFongPMLuJPEGylated PLGA nanoparticles for the improved delivery of doxorubicinNanomed200954410418
- AtyabiFTalaieFDinarvandRThiolated chitosan nanoparticles as an oral delivery system for amikacin: In vitro and ex vivo evaluationsJ Nanosci Nanotechnol2009984593460319928123
- EsmaeiliFDinarvandRGhahremaniMHDocetaxel-albumin conjugates: Preparation, in vitro evaluation and biodistribution studiesJ Pharm Sci20099882718273018972321
- SaremiSAtyabiFAkhlaghiSPOstadSNDinarvandRThiolated chitosan nanoparticles for enhancing oral absorption of docetaxel: Preparation, in vitro and ex vivo evaluationInt J Nanomedicine20116111912821289989
- AkhlaghiSPSaremiSOstadSNDinarvandRAtyabiFDiscriminated effects of thiolated chitosan-coated pMMA paclitaxel-loaded nano-particles on different normal and cancer cell linesNanomedicine20106568969720172052
- MajzoobSAtyabiFDorkooshFKafedjiiskiKLoretzBBernkop-SchnürchAPectin-cysteine conjugate: Synthesis and in- vitro evaluation of its potential for drug deliveryJ Pharm Pharmacol200658121601161017331323
- LeeESNaKBaeYHDoxorubicin loaded pH-sensitive polymeric micelles for reversal of resistant MCF-7 tumorJ Control Release2005103240541815763623
- VerdièreACDubernetCNématiFPouponMFPuisieuxFCouvreurPUptake of doxorubicin from loaded nanoparticles in multidrug-resistant leukemic murine cellsCancer Chemother Pharmacol19943365045088137462
- De VerdièreACDubernetCNématiFReversion of multidrug resistance with polyalkylcyanoacrylate nanoparticles: Towards a mechanism of actionBr J Cancer19977621982059231919
- WongHLBendayanRRauthAMXueHYBabakhanianKWuXYA mechanistic study of enhanced doxorubicin uptake and retention in multidrug resistant breast cancer cells using a polymer-lipid hybrid nanoparticle systemJ Pharmacol Exp Ther200631731372138116547167
- EhtezaziTGovenderTStolnikSHydrogen bonding and electrostatic interaction contributions to the interaction of a cationic drug with polyaspartic acidPharm Res200017787187710990208
- GovenderTEhtezaziTStolnikSIllumLDavisSSComplex formation between the anionic polymer (PAA) and a cationic drug (procaine HCl): characterization by microcalorimetric studiesPharm Res19991671125113110450942
- KitaevaMVMelik-NubarovNSMengerFMYaroslavovAADoxorubicin-poly(acrylic acid) complexes: interaction with liposomesLangmuir200420166575657915274557
- TianYBrombergLLinSNAlan HattonTTamKCComplexation and release of doxorubicin from its complexes with pluronic P85-b-poly(acrylic acid) block copolymersJ Control Release2007121313714517630011
- MargaritisAManochaBControlled release of doxorubicin from doxorubicin/γ-polyglutamic acid ionic complexJournal of Nanomaterials201018
- PratesiGSaviGPezzoniGPoly-L-aspartic acid as a carrier for doxorubicin: A comparative in vivo study of free and polymer-bound drugBr J Cancer19855268418484074638
- BogushTSmirnovaGShubinaISyrkinARobertJDirect evaluatlon of intracellular accumulation of free and polymer-bound anthracyclinesCancer Chemother Pharmacol19953565015057533670
- KwonGSYokoyamaMOkanoTSakuraiYKataokaKBiodistribution of micelle-forming polymer–drug conjugatesPharm Res19931079709748378259
- JeongY-INahJ-WLeeH-CKimS-HChoCSAdriamycin release from flower-type polymeric micelle based on star-block copolymer composed of poly([gamma]-benzyl-glutamate) as the hydrophobic part and poly(ethylene oxide) as the hydrophilic partInt J Pharm19991881495810528082
- KataokaKMatsumotoTYokoyamaMDoxorubicin-loaded poly(ethylene glycol)-poly([beta]-benzyl – aspartate) copolymer micelles: their pharmaceutical characteristics and biological significanceJ Control Release2000641–314315310640653
- OhILeeKKwonHYRelease of adriamycin from poly(I³-benzyl-L-glutamate)/poly(ethylene oxide) nanoparticlesInt J Pharm1999181110711510370207
- ChenYSynthesis of albumin-dextran sulfate microspheres possessing favourable loading and release characteristics for the anticancer drug doxorubicinJ Control Release19943114954
- JanesKAFresneauMPMarazuelaAFabraAAlonsoMJChitosan nanoparticles as delivery systems for doxorubicinJ Control Release2001732–325526711516503
- TanMLFriedhuberAMDunstanDEChoongPFMDassCRThe performance of doxorubicin encapsulated in chitosan-dextran sulphate microparticles in an osteosarcoma modelBiomaterials31354155119836833
- YangMChanHLLamWFongWFCytotoxicity and DNA binding characteristics of dextran-conjugated doxorubicinsBiochimica et Biophysica Acta – General Subjects199813803329335
- ShinozawaSFukudaTArakiYOdaTEffect of dextran sulfate on the survival time and mitochondrial function of adriamycin (doxorubicin)-treated miceToxicol Appl Pharmacol19857923533572408357
- BishtSMaitraADextran–doxorubicin/chitosan nanoparticles for solid tumor therapyWiley Interdisciplinary Reviews: Nanomedicine and Nanobiotechnology20091441542520049807
- LamWChanHYangMChengSFongWSynergism of energy starvation and dextran-conjugated doxorubicin in the killing of multidrug-resistant KB carcinoma cellsAnticancer Drugs199910217117810211547
- LamWLeungCHChanHLFongWFToxicity and DNA binding of dextran-doxorubicin conjugates in multidrug-resistant KB-V1 cells: optimization of dextran sizeAnticancer Drugs200011537738410912954
- SheldonKMarksABaumalRSensitivity of multidrug resistant KB-C1 cells to an antibody-dextran-adriamycin conjugateAnticancer Res1989936376412475051
- AngererJSchallerKHAnalyses of Hazardous Substances in Biological Materials7WeinheimWiley-VCH2001
- RubinSCChemotherapy of Gynecologic Cancers: Society of Gynecologic Oncologists Handbook2nd edBaltimoreLippincott Williams & Wilkins2003
- TianYRaviPBrombergLHattonTATamKCSynthesis and aggregation behavior of pluronic F87/poly(acrylic acid) block copolymer in the presence of doxorubicinLangmuir20072352638264617269798
- ChairesJBSatyanarayanaSSuhDFoktIPrzewlokaTPriebeWParsing the free energy of anthracycline antibiotic binding to DNABiochemistry1996357204720538652545
- HajianRShamsNMohagheghianMStudy on the interaction between doxorubicin and deoxyribonucleic acid with the use of methylene blue as a probeJ Braz Chem Soc200920813991405
- MichaelisLGranickSMetachromasy of basic dyestuffsJ Am Chem Soc194567712121219
- KrishnamurthyKVMethods in Cell Wall CytochemistryLLCCRC Press1999