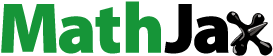
Abstract
A novel design of a blood cleaner on-chip using an optical waveguide known as a PANDA ring resonator is proposed. By controlling some suitable parameters, the optical vortices (gradient optical fields/wells) can be generated and used to form the trapping tools in the same way as optical tweezers. In operation, the trapping force is formed by the combination between the gradient field and scattering photons by using the intense optical vortices generated within the PANDA ring resonator. This can be used for blood waste trapping and moves dynamically within the blood cleaner on-chip system (artificial kidney), and is performed within the wavelength routers. Finally, the blood quality test is exploited by the external probe before sending to the destination. The advantage of the proposed kidney on-chip system is that the unwanted substances can be trapped and filtered from the artificial kidney, which can be available for blood cleaning applications.
Introduction
Human kidneys are important excretory organs whose main functions are maintaining the concentrations of various ions and other important substances constant, and the removal of wastes and other unwanted substances in the urine.Citation1 One living human kidney has from 300,000 to over 1,000,000 nephrons,Citation2 the number of which is related to birth weights.Citation3 Each nephron comprises two parts, namely the glomerulus and the tubules. The glomerulus is comprised of capillary vessels and the blood plasma is filtered from the blood through the porous wall of the vessels.Citation1
Chronic kidney disease (CKD) is a worldwide health problem increasing dramatically in several countries.Citation4,Citation5 This is a key focus for health care planning, even in the developed world, in which many clinical organizations have been established for the development of hemodialysis devices and methods.Citation6–Citation8 CKD and acute renal failure occurs when one suffers from gradual and usually permanent loss of kidney function over time and loses the ability to filter and remove waste and extra fluids from the body.
Hemodialysis is the process of removing waste products from the blood. This removal is normally done by the kidneys. If they are impaired and require treatment,Citation9 a dialysis machine (artificial kidney) can perform a similar function. The invention, manufacture, and implantation of the artificial kidney has been developed from the human organ.Citation10–Citation14 But artificial kidney dialysis has several disadvantages such as time wastage for blood dialysis (2–4 hours/visit), and the need for patients to visit the hospital every week (2–3 times/week). This has led researchers and inventors to develop an implantable artificial kidney,Citation15 which would increase convenience.
Optical trapping was first invented by Ashkin.Citation16 It emerged as a powerful tool with wide-reaching applications in biology, physics, engineering, and medicine.Citation17 The ability of optical trapping and manipulation of viruses, living cells, and bacteria without organelle damageCitation18 by laser radiation pressure has been demonstrated.Citation19,Citation20 This is specific to medicine and the application of nanotechnology. Lee et alCitation21,Citation22 carried out a single red blood cell (RBC) deformability test, which was performed by using optical trapping plastic in a microfluidics chip, and a lab-on-a-chip for RBC transportation in the capillary network to circulate oxygen and carbon dioxide throughout the human body.Citation23 The optical trapping manipulation molecules in the liquid-core waveguide and its application to drug delivery has been reported by Suwanpayak et al,Citation24 in which a PANDA ring resonator is used to form, transmit, and receive the microscopic volume of the drug by controlling the ring parameters. This microscopic volume can be trapped and transported dynamically within the wavelength router or network.
Recently, the promising technique of microscopic volume trapping and transportation within an add/drop multiplexer have been reported both in theoryCitation25 and experiment,Citation26 respectively. Here the transporter is known as an optical tweezer. The optical tweezer generation technique is used as a powerful tool for the manipulation of micrometer-sized particles. To date, the usefulness of static tweezers is well recognized. Moreover, the use of dynamic tweezers is now also used in practical work.Citation27–Citation29 Schulz et alCitation30 have shown the possibility of trapped atom transfer between two optical potentials. In principle, an optical tweezer uses the forces exerted by intensity gradients in the strongly focused beams of light to trap and move the microscopic volumes of matter by a combination of forces induced by the interaction between photons, due to the photon scattering effect. In application, the field intensity can be adjusted and tuned to the desired gradient field. The scattering force can then form the suitable trapping force. Hence, the appropriate force can be configured as the transmitter/receiver for performing long distance microscopic transportation.
In this paper, the dynamic optical tweezers/vortices are generated using dark soliton, bright soliton, and Gaussian pulse propagating within an add/drop optical multiplexer incorporating two nanoring resonators (PANDA ring resonator). The dynamical behavior of soliton and Gaussian pulse is well described by Tasakorn et al.Citation31 By using the proposed system, the blood waste and unwanted substances can be trapped and transported (filtered) from the artificial human kidney. Here, the required trapping tool sizes can be generated and formed for the specific blood waste molecules, where finally the clean blood can be obtained and sent to the destination via the through port. However, in practice, several sensors are required for environmental and blood quality control, which will be the topic of future investigation.
Theoretical background
In theory, the trapping forces are exerted by the intensity gradients in the strongly focused beams of light to trap and move the microscopic volumes of matter, in which the optical forces are customarily defined by the relationship below.Citation32
Here, Q is a dimensionless efficiency, nm is the refractive index of the suspending medium, c is the speed of light, and P is the incident laser power, measured using the specimen. Q represents the fraction of power utilized to exert force. For plane waves incident on a perfectly absorbing particle, Q is equal to 1. To achieve stable trapping, the radiation pressure must create a stable, three-dimensional equilibrium. Because biological specimens are usually contained in an aqueous medium, the dependence of F on nm can rarely be exploited to achieve higher trapping forces. Increasing the laser power is possible, but only over a limited range due to the possibility of optical damage. Q itself is therefore the main determinant of trapping force. It depends upon the NA (numerical aperture), laser wavelength, light polarization state, laser mode structure, relative index of refraction, and the geometry of the particle.
Furthermore, in the Rayleigh regime, trapping forces decompose naturally into two components. Since the electromagnetic field is uniform across the dielectric, particles can be treated as induced point dipoles. The scattering force is given by:Citation32
Here, σ is the scattering cross section of a Rayleigh sphere with radius r. 〈S〉 is the time averaged Poynting vector, n is the index of refraction of the particle, m = n/nm is the relative index, and k = 2πnm/λ is the wavenumber of the light. The scattering force is proportional to the energy flux and points along the direction of the propagation of the incident light. The gradient field (Fgrad) is the Lorentz force acting on the dipole induced by the light field, and is given by:Citation32
In our proposal, the trapping force is formed by using a dark soliton, in which the valley of the dark soliton is generated and controlled within the PANDA ring resonator by the control port signals. In operation, the optical tweezers can be trapped, transported, and stored within the PANDA ring resonator and wavelength router, which can be used to form the microscopic volume (molecule) transportation, thus allowing drug delivery via the waveguide.Citation34 The manipulation of trapped microscopic volumes within the optical tweezers has been previously reported.Citation25
Kidney on-chip manipulation
Microfluidics is a burgeoning field with important applications in areas such as medical devices, biotechnology, chemical synthesis, and analytical chemistry.Citation34 The Erickson labCitation35–Citation40 research interests revolve around the study of micronanofluidics, including advancing flows, delivery, and implantable devices for living organs, in combination with optics.Citation41–Citation43 In this paper, we propose use of the optical trapping tools in the application of blood manipulation dialysis (kidney dialysis) using the PANDA ring resonator systems by incorporating a wavelength router. In the blood cleaner on-chip (), we used the same theory of optical trapping and transportation technique for a blood dialysis system, in which the blood flowsCitation44,Citation45 into the input port of the PANDA ring resonator, where the PANDA ring resonator system 1 (P1) works the same way as PANDA ring resonator system 2 (P2). The whole blood volume can be trapped and delivered in liquid-core waveguide,Citation46 of both high and low refractive indices, depending on the blood concentration, in which the whole blood concentration is 20% (n = 1.35), 40% (n = 1.35), 60% (n = 1.37).Citation47,Citation48
In operation, when blood flows in the waveguide channel,Citation49,Citation50 it is detected by a blood concentrate detector (versatile sensor, 3).Citation51,Citation52 Most important for blood dialysis is protein reabsorption into the renal artery, with the clean blood protein sensorCitation53,Citation54 placed to check the protein quality before release into the urine. The protein sensor will detect the blood protein component and help to regulate blood circulation until it is clean. Additionally, oxygen can be fed into the system via the add port (control) for high quality whole blood which is detected by wireless blood pressureCitation55 before delivery into the renal artery. In this case, the filtrate components are sodium (∼0.4 nm), chlorine (∼0.6 nm), and glucose (∼0.72 nm).Citation56 Our system is integrated to be a single chip, which is equivalent to one Bowman’s capsule consisting of the glomerulus build-up in the capillary vessels.
A schematic of a blood cleaner is as shown in , which is formed by PANDA ring resonators and liquid-core waveguide as shown in . The waveguides were constructed by using different sizes and refractive indices as shown in , where in this case the blood cell index is 1.35. The different waveguides with sizes of 5–8 μm and 1 nm were constructed for blood cells and proteins, respectively. The whole blood is input into the system via liquid-core 1 (input port), and the required trapping molecules can be trapped and filtered by liquid-core 2. Finally, the unwanted substances are trapped and filtered via the drop ports. In , the whole blood cells are input into the blood cleaner via the input ports (Ein), which is equivalent to the blood flow into the afferent artery as shown in . After the blood waste substances are filtered by the glomerulus, they are filtrated via the proximal tube. Finally, the clean blood is received via the efferent artery. The blood artery is replaced by the blood waveguide structure, which is formed by the liquid-core waveguide as shown in , where the red blood cells (RBCs) with 5–8 μm are input into the system via the input ports (liquid core 1), while the unwanted substances are trapped by the optical tweezers and filtered by the drop ports. Liquid core 2 is used for the trapped proteins, in which the protein molecule size is 1 nm, which is smaller than the RBC, and therefore, the RCBs cannot be transported via the liquid-cores, which means only the unwanted substances are being trapped and transported in the liquid-core 2.
To form the optical trapping tools, a bright soliton with center wavelength at 1.50 μm, peak power 4 W, pulse 35fs is input into the system via the input port. The coupling coefficients are given as κ0 = 0.5, κ1 = 0.35, κ2 = 0.1, and κ3 = 0.35, respectively. The ring radii are Radd = 100 and 75 μm, RR = 40 and 20 μm, and RL = 40 and 20 μm, respectively. To date, the evidence of a practical device with a radius of 30 nm has been reported by Tasakorn et al.Citation31 Aeff are 300 μm2 (r ≈ 9.77 μm) and 0.5 μm2 (r ≈ 400 nm). In this case, the dynamic tweezers (gradient fields) can be in the form of bright solitons, Gaussian pulses, and dark solitons for trapping the required blood waste. In , there are four different center wavelengths of tweezers generated, where the dynamical movements are a) |E1|2, b) |E2|2 c) |E3|2, d) |E4|2, e) through port, and f) drop port signals. In this case, the trapped molecules (blood waste) are filtered and obtained by the drop port. For instance, by using a whole blood index (n) of 1.35, the trapping probe widths of 20 nm at the wavelength center at 1.60 μm are generated (see ). More results in terms of coupling constants are shown in , where in this case the tunable trapping can be obtained. In practice, the more reliable device (blood cleaner on-chip) fabrication parameters are the ring resonator radii instead of coupling constants. More results of the optical trapping probes generated within the PANDA ring with different wavelengths are as shown in ; the bright soliton is used as the control signal for the tunable result. The output optical tweezers of the through and drop ports with different coupling constants are as shown in , which forms the basis for the selected blood waste molecules. In application, the clean blood can be transported into the human body via the through port. The filtering molecules (urine) are received via the drop port. The advantage of the proposed system is that it can be fabricated on-chip and alternatively operated by a single device.Citation57 The proposed system can be performed as a hemofiltration device, which is 90 μm in depth, 200 μm in width, and 300 μm in length. The unwanted substances can be trapped (filtrated) by the optical gradient field, and can be delivered via the control port (different wavelengths).Citation58 In operation, blood concentration does not affect the optical trapping ability, because the blood refractive index is slightly different, although it may affect the filtration speed.
Conclusion
We have shown that blood waste molecules can be trapped and filtered (transported) from the artificial blood cleaner (kidney), which can be performed on-chip. By utilizing the appropriate dark soliton input power, the required trapping tool sizes can be controlled and obtained, where finally the clean blood can be obtained before sending to the final destination (through port). Moreover, oxygen can be fed into the system via the control port, which is available for blood cleaning application. However, in practice, the blood cleaner on-chip system requires a specific environment, in which there are several sensors required to integrate into the system as shown in , including bio-sensor, blood sensor, versatile sensor, protein sensor, and blood pressure sensor. Lastly, large molecules may be problematic for the generated trapping tool size. Therefore, the search for new media for guide pipes,Citation59 for instance, nano tube materials, and sensors, will be the next focus of investigation.
Acknowledgements
We would like to thank the Institute of Advanced Photonics Science, Nanotechnology Research Alliance, Universiti Teknologi Malaysia (UTM) and King Mongkut’s Institute of Technology (KMITL), Thailand for providing the research facilities. This research work has been supported by UTM’s Tier 1 Research Grant, MyBrain15 Fellowship, and the Ministry of Higher Education (MOHE) research grant.
Disclosure
No conflicts of interest were declared in relation to this paper.
References
- WessonLGPhysiology of human kidney The Jefferson Medical College of Philadelphia PaGrune and Stratton, IncNew York1969
- McNamaraBJDioufBDouglas-DentonRNHughsonMDHoyWEBertramJFComparison of nephron number, glomerular volume and kidney weight in Senegalese Africans and African AmericansNephrol Dial Transplant2010251514152020154008
- ManalichAReyesLHerreraMMelendiCFundoraIRelationship between weight at birth and the number and size of renal glomeruli in humans: a histomorphometric studyKidney Int20005877077710916101
- RashadSBarsoumMDChronic kidney disease in the developing worldN Engl J Med200635499799916525136
- ScarpioniRRicardiMMelfaLCristinelliLDyslipidemia in chronic kidney disease: are statins still indicated in reduction cardiovascular risk in patients on dialysis treatmentCardiovasc Ther20102836136820553296
- CoiraultCPournyJCLambertFLecarpentierYMechanical property analysis of stored red blood cell using optical tweezersMed Sci200319364367
- GrenierSRWeiMTBaiJJChiouADynamic deformation of red blood cell in dual-trap optical tweezersOpt Express201018104621047220588900
- NissensonARRoncoCPergamitGEdelsteinMWattsRContinuously functioning artificial nephron system: the promise of nanotechnologyHemodial Int2005921021716191071
- LocatelliFValderrabanoFHoenichNBommerJLeunissenKCambiVProgress in dialysis technoloty: membrame selection and patient outcomeNephrol Dial Transplant2000151133113910910435
- GuraVMacyASBeizaiMEzonCGolperTATechnical breakthroughs in the wearable artificial kidneyClin J Am Soc Nephrol200941441144819696219
- ForniLGHiltonPJContinuous hemofiltration in the treatment of acute renal failureN Engl J Med1997336130313399113935
- BennieJWilliamPMatherFW inventors.Method for making artificial kidney United States patent US 4211597198078
- HardyMD inventorImplantable artificial kidney United States patent US 5092886199233
- FissellWFleischmanAJHumesHDRoySDevelopment of continuous implantable renal replacement: past and futureTranslational Res2007150327336
- DykstraTM inventorKidney dialysis method and device United States patent US 5876366199932
- AshkinADziedzicJMYamaneTObservation of a single-beam gradient force optical trap for dielectricOpt Lett19861128829019730608
- LuSJFengQParkJSBiologic properties and enucleation of red blood cells from human embryonic stem cellsBlood20081124362436319029448
- ChenHDGeKLiYApplication of optical tweezers in the research of molecular interaction between lymphocyte function associated antigen-1 and its monoclonal antibodyCell Mol Immunol2007422122517601377
- AshkinADziedzicJMOptical trapping and manipulation of viruses and bacteriaScience1987235151715203547653
- ZhaoXSunYBuJZhuSYuanXCMicrolens array enabled on-chip optical trapping and sortingApplied Opt201150318322
- LeeWGParkKBangHSingle red blood cell defromnility test using optical trapping in plastic microfluid chipProceedings of the 31 Annual International IEEE EMBS Special Topic on Conference Microtechnologies in Medicine and BiologyKahuku, Oahu, Hawaii12–15 May 2005389390
- ObristDWeberBBuckAJennyPRed blood cell distribution in simplified capillaryPhil Trans R Soc A20103682897291820478913
- ChenYCChenGYLinYCWangGJA lab-on-a-chip capillary network for red blood cell hydrodynamicsMicrofluid Nanofluid20109585591
- SuwanpayakNJalilMATeekaTAliJYupapinPPOptical vortices generated by a PANDA ring resonator for drug trapping and delivery applicationsBio Med Opt Express20112159168
- PiyatamrongBKulsiriratKTechithdeeraMitathaSYupapinPPDynamic potential well generation and control using double resonators incorporating in an add/drop filterMod Phys Lett B20102430713082
- CaiHPoonAOptical manipulation and transport of microparticle on silicon nitride microring resonator – based add-drop devicesOpt Lett2010352855285720808347
- AshkinADziedzicJMYamaneTOptical trapping and manipulation of single cells using infrared laser beamsNature19873307697713320757
- EgashiraKTerasakiAKondowTPhoton-trap spectroscopy applied to molecules adsorbed on a solid surface: probing with a standing wave versus a propagating waveApp Opt19988051135115
- KachynskiAVKuzminANPudavarHEKaputaDSCartwrightANPrasadPNMeasurement of optical trapping forces by use of the two-photon-excited fluorescence of microspheresOpt Lett2003282288229014680158
- SchulzMCrepazHSchmidt-KalerFEschnerJBlattRTransfer of trapped atoms between two optical tweezer potentialsJ Mod Opt20075416191626
- TasakornMTeekaCJomtarakRYupapinPPMultitweezers generation control within a nanoring resonator systemOpt Eng201049075002
- SvobodaKBlockSMBiological applications of optical forcesAnnu Rev Biophys Biomol Struct1994232472837919782
- ZhuJOzdemirSKXiaoYFOn-chip single nanoparticle detection and sizing by mode splitting in an ultrahigh-Q microresonatorNat Photonics201044649
- SuwanpayakNYupapinPPMolecular buffer using a PANDA ring resonator for drug delivery useInt J Nanomed20116575580
- PsaltisDQuakeSRYangCDeveloping optofluidic technology through the fusion of microfluidics and opticsNature200644238138616871205
- YangAJHEricksonDOptofluidic ring resonator switch for optical particle transportLab Chip20101076977420221566
- ChungAJHuhYSEricksonDA robust, electrochemically driven microwell drug delivery system for controlled vasopressin releaseBiomed Microdevices20091186186719353273
- KrishnanMTolleyMLipsonHEricksonDHydrodynamically tunable affinities for fluidic assemblyLangmuir2009253769374419708253
- ChungAJEricksonDEngineering insect flight metabolics using immature stage implanted microfluidicsLab Chip2009966967619224016
- SchmidtBSYangAHJEricksonDLipsonMOptofluidic trapping and transport on solid core waveguides within a microfluidic deviceOptics Express20071143221433419550709
- SegevMChristodoulidesDNRotschildC inventorsMethod and system for manipulating fluid medium United States patent US 2011/0023973A201123
- BuggeMPalmersG inventorsImplantable device for utilization of the hydraulic energy of the heart United States patent US RE41,394E.2010622
- ChenSYHuSHLiuDMKuoKT inventors. Drug delivery nanodevice, its preparation method and used there of United States patent US 2011/0014296A12011120
- YamamotoKIKobayashiKEndoKHollow-fiber blood-dialysis membranes: superoxide generation, permeation, and dismutation measured by chemiluminescenceJ Artif Organs2005825726216362524
- HuangHWChing ShihTCLiauhCTPredicting effects of blood flow rate and size of vessels in a vasculature on hyperthermia treatments using computer simulationBio Med Engin On Line2010918
- HudetzAGBlood flow in the cerebral capillary network: a review emphasizing observations with intravital microscopyMicrocirculation199742332529219216
- SardarDKLevyLBOptical properties of whole bloodLasers Med Sci199813106111
- BonannoLMDeLouiseLAWhole blood optical biosensorBiosens Bioelectron20072344444817720473
- HarrisonRVHarelNPanesarJMountRJBlood capillary distribution correlates with hemodynamic-based functional imaging in cerebral cortexCerebral Cortex20021222523311839597
- TurkstraEBraamBKoomansHAImpaired renal blood flow autoregulation in two-kidney, one-clip hypertensive rats is caused by enhanced activity of nitric oxideJ Am Soc Nephrol20001184785510770962
- ThomsonRJ inventor.Blood concentrate detector United States patent US 2009/0310123A1.20091217
- CarraraSNano-bio-technology and sensing chips: new systems for detection in personalized therapies and cell biologySensors201010526543
- PreejithPVLimCSKishenAJohnMSAsundiATotal protein measurement using a fiber-optic evanescent wave-based biosensorBiotechnol Lett20032510511012882283
- DasJKelleySOProtein detection using arrayed microsensor chips: tuning sensor footprint to achieve ultrasensitive readout of CA-125 in serum and whole bloodAnal Chem2011831167117221244005
- OyriKBalasinghamISamsetEHøgetveitJOFosseEWireless continuous arterial blood pressure monitoring during surgery: a pilot studyAnesth Analg200610247848316428546
- HsuTWChenYCWuMJLiAFYYangWCNgYYReinfusion of ascites during hemodialysis as a treatment of massive refractory ascites and acute renal failureInt J Nephrol Renovasc Dis20014293321694946
- SantiniJTRichardsACScheidtRCimaMJLangerRMicrochip as controlled drug delivery devicesAngew Chem Int Ed20003923962407
- JesacherAMaurerCFurhapterSSchwaighoferABernetSRitsch-MarteMOptical tweezers of programmable shape with transverse scattering forcesOpt Comm200828122072212
- AshkinAOptical trapping and manipulation of neutral particles using lasersProc Natl Acad Sci U S A199794485348609144154