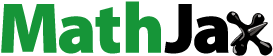
Abstract
This study concerns the encapsulation and controlled release of both hydrophobic and hydrophilic medications with one polymer, which are delivered together as a combined therapy to treat diseased tissue. To test our hypothesis that the novel PEG-graft-PLA (PEG, polyethylene glycol; PLA, polylactic acid) can deliver both the hydrophobic and hydrophilic medications on account of its amphiphility, charge, and graft structure, PEG-graft-PLA (molecular weight of PEG = 1900) with very low critical micelle concentration was synthesized. One hydrophilic (insulin) and one hydrophobic (naproxen) model medication were loaded in separately during its self-assembly in aqueous solution. The resulting nanoparticles (NPs) were narrowly distributed and spherical, with average particle size around 200 nm, zeta potential >−10 mV, and encapsulation efficiency >50%. The NPs realized controlled release of insulin and naproxen for over 24 and 160 hours, respectively. Specifically, the bioactivity of the insulin released from the NPs was maintained. Owing to encapsulation, both for hydrophobic and hydrophilic medicines, and NPs obtained with similar size and zeta potential, as well as maintenance of bioactivity of loaded protein, we expect the applications of PEG-graft-PLA NPs in combination therapy.
Introduction
Many studies have reported that, in many diseases, combination therapy is more effective than monotherapy.Citation1–Citation5 Although combination therapy is costlier than monotherapy in the short term, it offers significant savings in terms of lower treatment-failure rate, lower case-fatality ratios, slower development of resistance, and, consequently, is less expensive for the development of new drugs. In combination therapy, various medications, irrespective of their hydrophobic or hydrophilic nature, have been used.Citation1–Citation5 In particular, proteins, one of the most effective and fastest growing group of medicines for the treatment of nearly 150 diseases, including various severe chronic conditions such as cancer, diabetes, hepatitis, leukemia, and rheumatoid arthritis, have shown prominent effects.Citation6 However, to date, very few studies have reported the delivery of both hydrophobic and hydrophilic medications with one biomaterial, even though many single-drug delivery studies have been conducted. Moreover, several problems still exist in protein-delivery vehicles, especially due to the denaturation of entrapped proteins.Citation7–Citation10 We aimed at developing a system with the capability of encapsulation and controlled release of both hydrophobic and hydrophilic medications, especially proteins, and delivering them to the same diseased tissue to realize combination therapy. In this study, we focused on carrying the hydrophobic and hydrophilic drugs separately.
Polylactic acid (PLA), a known US Food and Drug Administration-approved biodegradable polymer for bio-medical applications,Citation11 has been studied as a drug carrier in particle form since 1970.Citation12 However, various drawbacks have limited its further application. First, the emulsion method has to be used in particle preparation due to the hydrophobicity of PLA, where a relatively large amount of organic solvent (eg, chloroform, dichloromethane) and surfactant are added. Even though the organic solvent is removed afterwards, the residual solvent and surfactant may have harmful or cytotoxic effects. Second, the particle size prepared by emulsion is generally in the micrometer range, which limits the encapsulation efficiency and application of PLA particles compared with nanoparticles (NPs). Last, PLA particles are easily captured by the reticulo-endothelial systemCitation13 in vivo and eliminated from the body, which in turn limits the use ratio of loaded medicine.
To overcome these shortcomings, amphiphilic polymers of polyethylene glycol (PEG)–block-PLA, such as diblock polymer of PLA-PEG and triblock polymer of PEG-PLA-PEG, have been synthesized and applied in the encapsulation of medications of small molecular weight (Mw), including paclitaxel,Citation14 papaverine,Citation15 sulindac, and tetracaine.Citation16 PEG-block- PLA is a surfactant polymer that can self-assemble into nanomicelles and entrap medicines simultaneously, which simplifies particle preparation and avoids or minimizes the use of the surfactant and organic solvent. Moreover, nanoscale vehicles improve the loading rate of medicine compared with microscale formulations. The introduction of PEG block also suppresses cellular and protein adsorption, thus increasing the circulation time of PEG-block-PLA micelles in vivo and the effectiveness of the medicine.Citation17 However, although PEG-block-PLA improves PLA in many ways, only small hydrophobic medications have been encapsulated in them.Citation15–Citation19 Furthermore, to obtain stable nanomicelles, PEG with a molecular weight (Mw) >4000 Da is usually used in the PEG-block- PLA, which might not be degraded in the body.Citation20 In our previous study, a PEG-graft-PLA with PEG of lower Mw (600 Da) self-assembled into NPs in water and showed good protein resistance and cellular compatibility in vitro.Citation21
In the current study, we hypothesized that PEG-graft-PLA could realize the delivery of both hydrophobic and hydrophilic medications via self-assembly in aqueous medium and form NPs with increased circulation time compared with particles of PLA alone, on account of its amiphiphility, charge, and graft structure. To test our hypothesis, PEG-graft-PLA with PEG of Mw 1900 Da was synthesized, followed by the critical micelle concentration (CMC) test. One protein and one small hydrophobic model medication, insulin and naproxen, respectively, were chosen to be loaded. Insulin, a peptide hormone composed of 51 amino acids with Mw of 5808 Da and isoelectric point of 5.3, was chosen to explore the possibility of PEG-graft-PLA to encapsulate protein medications and keep their bioactivity during entrapment and release. Naproxen, a nonsteroidal anti-inflammatory drug with a carboxyl group and Mw of 230 Da, was chosen to study the capability of PEG-graft-PLA to encapsulate small hydrophobic medications and compare the effect with PEG-block- PLA. The NPs encapsulated with or without (nude NPs) insulin or naproxen were prepared and characterized. The stability of the NPs under dilution or pH change as well as the controlled release of medications was studied in vitro.
Methods
Synthesis of PEG-graft-PLA
The PEG-graft-PLA was synthesized as described previously. Citation21 Briefly, 20 and 5 mL of tetrahydrofuran (THF) were used to dissolve 6.12 g of O,O′-Bis-(2-aminopropyl) polypropylene glycol-block-polyethylene oxide-block-polypropylene glycol (H2N-PEG-NH2, Mw 1900 Da, Sigma, St Louis, MO) and 10.00 g of 10% maleic anhydride graft PLA (MPLA), prepared in our lab,Citation22 respectively. To MPLA, H2N-PEG-NH2 was added dropwise at 50–55°C, followed by 1 more hour of stirring. The resulting product was purified by precipitation in 160 mL distilled water and dissolution in 20 mL THF three times before being vacuum-dried at room temperature to a stable weight. A white and nontransparent solid (that is, PEG-graft-PLA), soluble in acetone, chloroform, dimethylformamide (DMF), and dimethyl sulfoxide (DMSO) was yielded. A higher Mw PEG (Mw = 1900) was used in this study to realize the encapsulation of both the hydrophobic and hydrophilic materials.
CMC tests
To evaluate the surfactivity and the possibility of encapsulation, CMC of PEG-graft-PLA was determined using the established fluorescence method, with pyrene (Sigma, St Louis, MO) as extrinsic probe.Citation23 Serial solutions with fixed pyrene concentration of 6.0 × 10−7 M and various concentrations of PEG-graft-PLA between 1.08 × 10−6 and 2.16 × 10−2 mg/mL were prepared. The excitation intensity of pyrene at 329 and 327 nm was obtained (PerkinElmer LS-50B, Waltham, MA) according to the scanning excitation spectrum between 321 and 340 nm by fixing the emission spectrum at 394 nm and the excitation and emission bandwidth at 2.5 nm. The CMC was determined by taking the midpoint in the plot of the intensity ratio of 329/327 to the logarithm concentration of PEG-graft-PLA.
Preparation of nude and insulin or naproxen-loaded NPs
Procine insulin (Wanbang Biopharma, Jiangsu, China) was loaded into PEG-graft-PLA according to the following procedure: 10 mg PEG-graft-PLA dissolved in 1 mL organic solvent was dropped into 2 mg insulin solution, obtained by dissolution in 10 mL of 0.01 N HCl and pH adjusted to 5.3 with 2 N NaOH under constant stirring. Naproxen (Hengshuo Chemical Co Ltd, Hubei, China) was entrapped by co-dissolving 0.8 mg naproxen and 10 mg PEG-graft-PLA in 1 mL organic solvent and dropping into 10 mL distilled water under stirring (0.8 mg naproxen was supersaturated in 10 mL distilled water). A different organic solvent that can easily mix with water such as acetone, THF, DMF, or DMSO could be used in this preparation. After evaporation (for acetone or THF) or dialysis (for DMF or DMSO), to remove organic solvent, NPs encapsulated with insulin or naproxen were obtained. The main difference in using these solvents was the stability of the obtained NPs. The NPs prepared with THF were not as stable as those prepared with acetone, DMF, and DMSO. We applied the water solution of pH 5.3 (for insulin) and distilled water (for naproxen) during the entrapment to get lower solubility of the medicine in the solution and possible higher loading rate in the NPs. The nude PEG-graft-PLA NPs were prepared by dissolving 10 mg PEG-graft-PLA in 1 mL organic solvent and dropping into 10 mL phosphate buffered saline (PBS) under stirring before removal of the organic solvent.
NP characterization
The morphology of the nude NPs and the insulin- or naproxen-loaded NPs was observed by a Philips Tecnai 10 transmission electron microscope (Philips, Eindhoven, The Netherlands) under 80 kV after staining with 2% sodium phosphotungstate for 3 minutes. Average size and zeta potential of the NP solution were tested at room temperature by a dynamic light scattering (DLS) instrument (Zetasizer Nano S90; Malvern Instruments, Worcestershire, UK) using argon ion laser.
NP stability tests
Since NPs are diluted or encounter an acidic or basic environment besides the neutral conditions in the body, we studied the stability of various NPs under dilution (in aqueous solutions with PBS) or pH change (acidic and basic conditions). The stability of the NPs was also delineated as the average particle size and zeta potential change before and after dilution (10 or 100 times) or pH change (2.0 or 10.5, adjusted with 0.1 N HCl or NaOH, respectively).
Protein adsorption on the surface of NPs
The protein adsorption on the surface of the NPs was used to evaluate its possible circulation time in vivo.Citation21 The fluorospectrophotometer was used with fluorescein 5- isothiocyanate conjugated bovine serum albumin (FITC-BSA) as model protein based on the fact that albumin is the dominant protein in human plasma when adsorbed on PLA.Citation24 A 5-mL original NP solution was mixed with 5 mL FITC-BSA water solution (0.04 mg/mL) and incubated at 37°C for 40 minutes with stirring before NPs were collected by repetitive high-speed centrifuge (Ultra Centrifuge, Beckman Coulter, Inc, Brea, CA) at 18,000 rpm for 10 minutes and washed with Millipore water. The FITC-BSA-incubated NPs were vacuum-dried for 24 hours and dissolved with 3 mL DMF. The fluorescent emission of the DMF solution was tested with [θ] spectrofluorometer (PerkinElmer LS-50B, Maltham, MA) at 510 nm with 490 nm excitation. PLA particles prepared by dropping PLA solution in THF to 1% gelatin solution in water were used as control. The particle size was recorded before adsorption measurement. The ratio of FITC-BSA adsorbed on the particles of PEG-graft-PLA and PLA was calculated according to their particle size (D) and fluorescent emission (E) values as below:
The encapsulation efficiency and in vitro release of insulin and naproxen
The encapsulation efficiency (EE) of insulin in the NPs was tested by centrifuging the loaded NPs at 16,000 rpm, 4°C for 20 minutes, collecting the supernatant, which contained the unencapsulated insulin, and measuring its concentration at 595 nm (PerkinElmer LS-50B) after reaction with Coomassie brilliant blue for 10 minutes. The EE was calculated according to the equation:
The cumulative release of insulin was tested at 37°C and 150 rpm in 30 mL of NP solution, prepared with 5 mL original NP solution (without removing the unencapsulated insulin) plus 25 mL 0.01 M PBS. At various timepoints, 1 mL solution was taken out, centrifuged at 16,000 rpm to collect supernatant, and tested for insulin concentration (Coomassie brilliant blue coloration method) and bioactivity (porcine insulin enzyme-linked immunosorbent assay [ELISA] kit, R & D Systems, Inc, Minneapolis, MN). The cumulative release of insulin was expressed as the percentage change of insulin amount compared with total entrapped insulin (tested with Coomassie brilliant blue coloration and ELISA method) in bath solution over time. In parallel, the bioactivity of 0.08 mg/mL insulin water solution (same concentration as the unencapsulated insulin in the NP solution) was tested with ELISA as a control. The EE of naproxen was tested by centrifuging the NPs at 3000 rpm to collect the unencapsulated and insoluble naproxen, followed by dissolving the precipitate with DMF and testing the concentration of naproxen in DMF at 331 nm. Our preliminary studies have shown that the insoluble naproxen in water solution could be removed by centrifugation at <3000 rpm. The EE was calculated by:
The cumulative release of naproxen was tested with the dialysis method at 37°C and 150 rpm. A 5-mL NP solution in which the unencapsulated and insoluble naproxen had been removed via centrifuge at 3000 rpm was put into a dialysis membrane (cut off Mw = 8000 Da) followed by the addition of 45 mL PBS as dialysis solution. A 1-mL dialysis solution was sampled, and an equal amount of fresh PBS was added at various timepoints. The naproxen concentration was directly tested at 331 nm where the PEG-graft-PLA had no absorbance. The cumulative release of naproxen was expressed as the percentage change of naproxen amount in dialysis solution compared with the total entrapped naproxen over time. Each test was replicated three times.
Results
Low CMC of PEG-graft-PLA in water
The CMC of PEG-graft-PLA in water was 2.0 × 10−3 mg/mL, as shown in , which was comparable to PEG-block-PLACitation16 and one order lower than Tween 80 (ie, 1.4 × 10−2 mg/mL), one of the most commonly used surfactants in particle preparation. The result showed its good surfactivity.
Figure 1 CMC determination of PEG-graft-PLA. (A) Excitation spectra of pyrene from 321 to 340 nm as a function of PEG-graft-PLA concentration in water. (B) Intensity ratio (329/327) of pyrene versus logarithm concentration of PEG-graft-PLA. The CMC was determined by taking the midpoint in the plot of (B), which was 2.0 × 10−3 mg/mL.
Abbreviations: CMC, critical micelle concentration; PEG, polyethylene glycol; PLA, polylactic acid.
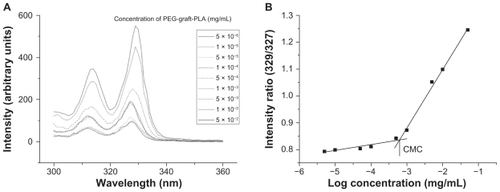
Morphology and nanostructure of NPs
The nude NPs as well as NPs loaded with insulin or naproxen were found to be spherical in structure, as shown in . The representative average particle size was 114.7 nm (for nude NPs), 173 nm (for insulin), and 278.4 nm (for naproxen). The size distribution of NPs was very low (PDI [polydispersity index] was <0.3). The representative average zeta potential was −27.84 mV for nude NPs, −45.21 mV for insulin, and −14.42 mV for naproxen (, original NPs).
Figure 2 Spherical morphology of nude NPs (A) and NPs encapsulated with insulin (B) or naproxen (C) observed via transmission electron microscopy.
Note: Scale bar: 200 nm.
Abbreviation: NP, nanoparticle.
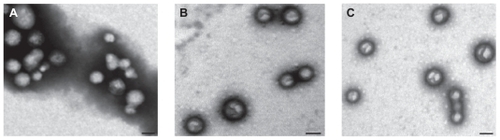
Figure 3 Particle size (top figures), its polydispersity (the value above the column on the top figures) and zeta potential (bottom figures) of nude NPs (A), NPs encapsulated with insulin (B) or naproxen (C), with or without dilution and pH changes, tested by dynamic light scattering.
Abbreviation: NP, nanoparticle.

Stability of NPs under dilution and pH change
The NPs loaded with or without insulin or naproxen were relatively stable after 10× and 100× dilution as well as pH change to 10.5 (), while the NPs remained stable during the acidification, until the pH jumped to 2.0 when they aggregated immediately (). The results suggested the buffering effect of PEG-graft-PLA in aqueous solution.
BSA-resistant property of NPs
There was 95.1% less FITC-BSA adsorbed on nude NPs of PEG-graft-PLA than on nude PLA particles. The results indicated the BSA-resistant property of PEG-graft-PLA NPs and possible longer circulation in the bloodstream.Citation21
Encapsulation and in vitro release of insulin and naproxen
The encapsulation efficiency of insulin and naproxen in NPs of PEG-graft-PLA was >60% and 50%, respectively. About 80% of the loaded insulin and naproxen were released within 24 hours for insulin (tested with Coomassie brilliant blue coloration method) and 160 hours for naproxen (). The burst release of insulin and naproxen within 2 hours was about 27% and 32%, respectively, which may relate to the surface adsorption of medicine on NPs (). We found a slight drop at 4 hours compared with 2 hours in insulin release curve which may be due to the re-adsorption of burst-released insulin onto the NPs while there was less release, or no release at all, of inner insulin from the NPs.
Figure 4 Typical in vitro insulin (A) (tested with Coomassie brilliant blue coloration method) and naproxen (B) release profile from nanoparticles of PEG-graft-PLA.
Abbreviations: PEG, polyethylene glycol; PLA, polylactic acid.
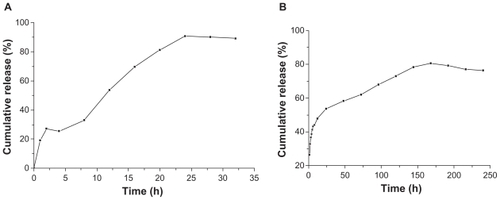
We found that the bioactivity of insulin in PBS solution decreased with time (half-life was about 8 hours), while the bioactivity in NP solution appeared to increase a little and then drop (half-life was about 24 hours) (). The increase within 2 hours was from the burst release, which showed that the burst-released insulin was bioactive. The bioactivity of insulin released from the NPs, calculated by the bioactivity in NPs solution minus the bioactivity in PBS, increased with time (), from which we inferred that the bioactive insulin was released from the NPs for over 24 hours ().
Discussion
Although a number of studies have reported the delivery of single medicines, very few studies have shown the development of self-assembled NPs with one polymer in aqueous solution which could carry both the hydrophobic and hydrophilic medications, particularly the large proteins with sustained bioactivity. PEG-graft-PLA is a kind of amphiphilic polymer with graft PEG structure that is flexible in solution. It contains free amino and carboxyl groups that ionize and interact in water. These features enable it to self-assemble and entrap chemicals via hydrophilic/hydrophobic and electrostatic interaction. Our results demonstrated the good surfactivity of PEG-graft-PLA and its capability to load insulin and naproxen during self-assembly in aqueous medium to receive NPs and realize controlled release. In fact, we also found that the PEG-graft-PLA can encapsulate during the self-assembly and controlled release of many other hydrophobic and hydrophilic chemicals, including sodium fluorescein and fluorescein isothiocyanate in aqueous solution (data not shown). The potential encapsulation of PEG-graft- PLA for the hydrophobic and hydrophilic materials implied that PEG-graft-PLA formed a hydrophobic and hydrophilic inner-layer with a hydrophilic out-layer during self-assembly in aqueous solution, as shown in . Thus, we expected these NPs loaded with different drugs would arrive at the same diseased tissue and realize the combination therapy according to their similar chemical character, particle size, and zeta potential. Of course, it needs further study.
Figure 6 The formation of PEG-graft-PLA NPs when loaded with insulin (A) and naproxen (B).
Abbreviations: PEG, polyethylene glycol; PLA, polylactic acid.

We obtained the spherical, narrowly distributed, and negatively charged NPs loaded with insulin or naproxen by way of self-assembly, with no surfactant and much less of the organic solvent compared with when PLA particles were used. So we would expect the PEG-graft-PLA NPs to be more biocompatible than the PLA particles. The NPs have particle size around 200 nm, zeta potential >−10 mV, and encapsulation efficiency >50%. The first order kinetics with >80% release of insulin and naproxen was realized within 24 hours and 160 hours, respectively, in vitro (). In addition, the NPs of PEG-graft-PLA showed BSA-resistant properties compared with PLA particles. In fact, we have previously demonstrated the BSA resistance of PEG-graft-PLA film compared with PLA film.Citation21 The good protein-resistant property of PEG-graft-PLA NPs may ensure its long circulation in the bloodstream.Citation21 The stability study disclosed that the NPs could be stable after administration in the body and diluted by blood or encountered with neutral (ie, pH 7.0) and basic tissue fluid, eg, intestinal fluid, but may aggregate in acidic fluid, especially in the gastric fluid. Therefore, it is reasonable to expect that the NPs of PEG-graft-PLA could be acidic-environment targeting and used for targeted and controlled drug delivery for stomach diseases and many tumors that have lower pH than normal tissue. We also found the original NP solution loaded with insulin or naproxen was stable at room temperature for at least 1 week.
Many studies have used chitosan with or without other polymers, such as Eudragit L 100-55,Citation25 arabic gum,Citation26 dextran sulfate,Citation27 and alginateCitation28 to prepare NPs with encapsulated insulin owing to its positive charge, and potential mucoadhesive and membrane permeable properties. They achieved particle size of 200–750 nm, zeta potential of −5 to −15 mV, encapsulation efficiency of 40%–85%, entrapment of 3.38%,Citation25 and controlled release of <30 minutesCitation28 and 6 hours.Citation27 A crosslinked dextran,Citation29 insulin-sodium oleate complex-loaded poly(lactide-co-glycolide),Citation30 and polyelectrolyteCitation31 NPs got an encapsulation efficiency of 45%–91.2%, particle size of 100–250 nm, and controlled release with 75%–95% entrapped insulin within 48 hoursCitation29 and 6 hours.Citation31 We have acheived particle size and encapsulation efficiency comparable to the NPs obtained in these studies, but prominent higher zeta potential value even after dilution or pH change to basic conditions (). The high zeta potential hints at the stability of NPs under storage. Citation32 Moreover, the high negative charge of insulin-loaded PEG-graft-PLA NPs favors their long stay in the bloodstream because the surface of blood cells and endothelia are negatively charged.Citation33 Sarmento et al also tested the bioactivity and concentration of insulin released from polysaccharide NPs with ELISA and the Bradford method and found that the relative bioactivity, a comparison of bioactivity with the concentration, remained for up to 24 hours.Citation34 We found the relative concentration of both the pure insulin in PBS and insulin released from NPs decreased with time in 24 hours, even though the latter decreased slower than the former (data not shown). In addition, the storage of raw insulin influenced the loading and release results due to its aggregation. The results we report here were from fresh insulin. Our study demonstrated that insulin released from NPs retained its bioactivity, which makes PEG-graft-PLA NPs more attractive for other therapeutic protein delivery, although more in vivo studies are needed.
Poly(D,L-lactide-co-glycolide),Citation35 poly-caprolactone,Citation36 liposomes,Citation37 and aspartate-based dendrimersCitation38 have been used for naproxen delivery, with particle size ranging from 100 to 723 nm, a loading ratio of up to 98%,Citation35 a sustained 1-month in vivo release,Citation36 and an increased solubility of up to two-fold at pH 6 and 7.Citation38 PEG-block-PLA used for small hydrophobic-medicine delivery gave particle sizes as small as 45 nm,Citation16 loading rate up to 25%,Citation16 controlled release of up to 450 hours,Citation17 and 5000-fold increased solubility.Citation16 We obtained effects comparable with the published naproxen delivery systems and the PEG-block-PLA for small hydrophobic medications.
In conclusion, we have developed PEG-graft-PLA NPs with the potential for encapsulation and controlled release of both hydrophobic and hydrophilic medications. Specifically, they retained the bioactivity of the entrapped protein.
Acknowledgments
PJ thanks NSF of China (No. 10972243&30300084) and “111” Project of China (No. B06023) for supporting this research.
Disclosure
The authors report no conflicts of interest in this work.
References
- KamravaMTsangKYMadanRAKaushalAColemanCNGulleyJLong-term survival and PSA control with radiation and immunotherapy for node positive prostate cancerClin Dev Immunol2009200936391420224813
- TanabeKSuzukiTTokumotoNYamamotoHYoshidaKOhdanHCombination therapy with docetaxel and S-1 as a first-line treatment in patients with advanced or recurrent gastric cancer: a retrospective analysisWorld J Surg Oncol201084020482840
- ChaoMPAlizadehAATangCAnti-CD47 antibody synergizes with rituximab to promote phagocytosis and eradicate non-Hodgkin lymphomaCell201014269971320813259
- BlierPWardHETremblayPLabergeLHébertCBergeronRCombination of antidepressant medications from treatment initiation for major depressive disorder: a double-blind randomized studyAm J Psychiatry201016728128820008946
- GranichRCrowleySVitoriaMHighly active antiretroviral treatment as prevention of HIV transmission: review of scientific evidence and updateCurr Opin HIV AIDS2010529830420543604
- FrokjaerSOtzenDEProtein drugs stability: a formulation challengeNat Rev Drug Discov2005429830615803194
- BartusRTTracyMAEmerichDFZaleSESustained delivery of proteins for novel therapeutic productsScience1988281116111629735031
- PutneySDBurkePAImproving protein therapeutics with sustained-release formulationsNat Biotechnol1998161531579487521
- ClelandJLJohnsonOLPutneySJonesAJSRecombinant human growth hormone poly(lactic-co-glycolic acid) microsphere formulation developmentAdv Drug Deliv Rev199728718410837565
- WuFJinTPolymer-based sustained-release dosage forms for protein drugs, challenges, and recent advancesAAPS PharmSci Tech2009912181229
- ZhangXMcAuleyKBGossenMFATowards prediction of release profiles of antibiotics from coated poly(DL-lactide) cylindersJ Control Release199534175179
- YollesSLeafeTDWoodandJHMeyerFJLong acting delivery systems for narcotic antagonists II: release rates of naltrexone from poly(lactic acid) compositesJ Pharm Sci1975643483491127596
- CaoXShoichetMSDelivering neuroactive molecules from biodegradable microspheres for application in central nervous system disordersBiomaterials19992032933910048405
- BurtHMZhangXToleikisPEmbreeLHunterWLDevelopment of copolymers of poly(D,L-lactide) and methoxypolyethylene glycol as micelles carriers of paclitaxelColloids Surfaces199916161171
- LeeJChoECChoKIncorporation and release behavior of hydrophobic drug in functionalized poly(D,L-lactide)-blockpoly(ethylene oxide) micellesJ Control Release20049432333514744484
- AgrawalSKSanabria-DelongNCoburnJMTewGNBhatiaSRNovel drug release profiles from micellar solutions of PLA-PEG-PLA triblock copolymersJ Control Release2006112647116507325
- YamamotoYNagasakiYKatoYSugiyamaYKataokaKLong-circulating poly(ethylene glycol)-poly(D,L-lactide) block copolymer micelles with modulated surface chargeJ Control Release200177273811689257
- CalicetiPSalmasoSElvassoreNBertuccoAEffective protein release from PEG/PLA nano-particles produced by compressed gas anti-solvent precipitation techniquesJ Control Release20049419520514684283
- ChengJTeplyBASherifiIFormulation of functionalized PLGA–PEG nanoparticles for in vivo targeted drug deliveryBiomaterials20072886987617055572
- WenJKimGJALeongKWPoly(D,L-lactide-co-ethyl ethylene phosphate)s as new drug carriersJ Control Release200392394814499184
- PanJZhaoMLiuYWangBMiLYangLDevelopment of a new poly(ethylene glycol)-graft-poly(D,L-lactic acid) as potential drug carriersJ Biomed Mater Res A20098916016718431784
- PanJWangYQinSZhangBLuoYGrafting reaction of poly(D,L) lactic acid with maleic anhydride and hexanediamine to introduce more reactive groups in its bulkJ Biomed Mater Res B Appl Biomater20057447648015889445
- KwonGNaitoMYokoyamaMOkanoTSakuraiYKataokaKMicelles based on AB block copolymers of poly(ethylene oxide) and poly(β-benzyl L-aspartate)Langmuir19939945949
- GrefRLückMQuellecP‘Stealth’ corona-core nanoparticles surface modified by polyethylene glycol (PEG): Influences of the corona (PEG chain length and surface density) and of the core composition on phagocytic uptake and plasma protein adsorptionColloids Surf B Biointerfaces20001830131310915952
- JelvehgariMZakeri-MilaniPSiahi-ShadbadMRDevelopment of pH-sensitive insulin nanoparticles using Eudragit L100–155 and chitosan with different molecular weightsAAPS Pharm Sci Tech201011312371242
- AvadiMRSadeghiAMMohammadpourNPreparation and characterization of insulin nanoparticles using chitosan and Arabic gum with ionic gelation methodNanomedicine20106586319447202
- SarmentoBRibeiroAVeigaFFerreiraDDevelopment and characterization of new insulin containing polysaccharide nanoparticlesColloids Surf B Biointerfaces20065319320217049214
- SarmentoBRibeiroAVeigaFSampaioPNeufeldRFerreiraDAlginate/chitosan nanoparticles are effective for oral insulin deliveryPharm Res2007242198220617577641
- ChalasaniKKRussell-JonesGJYandrapuSKDiwanPVJainSKA novel vitamin B12-nanosphere conjugate carrier system for peroral delivery of insulinJ Control Release200711742142917239471
- SunSLiangNPiaoHYamamotoHKawashimaYCuiFInsulin-S.O (sodium oleate) complex-loaded PLGA nanoparticles: formulation, characterization and in vivo evaluationJ Microencapsul20102747147820113168
- FanYFWangYNFanYGMaJBPreparation of insulin nanoparticles and their encapsulation with biodegradable polyelectrolytes via the layer-by-layer adsorptionInt J Pharm200632415816716814967
- Zeta Potential-An Introduction in 30 Minutes. Technical Note Available from: http://www.malvern.com/malvern/kbase.nsf/allbyno/KB000734/$file/MRK654-01An Introduction to Zeta Potential v3.pdfAccessed May 4, 2011
- MillerLHPowersKGFinertyJVanderbergJPDifference in surface charge between host cells and malarial parasitesJ Parasitol1973599259274200702
- SarmentoBRibeiroAVeigaFFerreiraDDevelopment and characterization of new insulin containing polysaccharide nanoparticlesColloids Surf B Biointerfaces20065319320217049214
- JavadzadehYAhadiFDavaranSMohammadiGSabzevariAAdibkiaKPreparation and physicochemical characterization of naproxen-PLGA nanoparticlesColloids Surf B Biointerfaces201081249850220719477
- RodriguesMRLanzariniCMRicci-JuniorEPreparation, in vitro characterization and in vivo release of naproxen loaded in poly-caprolactone nanoparticlesPharm Dev Technol2011161122120001271
- PugliaCBoninaFRizzaLEvaluation of percutaneous absorption of naproxen from different liposomal formulationsJ Pharm Sci2010992819282920039387
- OuyangLMaLJiangBLiYHeDGuoLSynthesis of novel dendrimers having aspartate grafts and their ability to enhance the aqueous solubility of model drugsEur J Med Chem2010452705271120171762