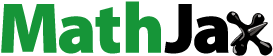
Abstract
To facilitate locomotion and support the body, the skeleton relies on the transmission of forces between muscles and bones through complex junctions called entheses. The varying mechanical and biological properties of the enthesis make healing this avascular tissue difficult; hence the need for an engineered alternative. Cells in situ interact with their environment on the nano-scale which suggests that engineered approaches to enthesis regeneration should include such biologically-inspired nano-scale surface features. The present in vitro study investigated the effects of etching poly-lactic-co-glycolic acid (PLGA) scaffolds to produce nano-topography on the adhesion of fibroblasts and osteoblasts, two integral enthesis cell types. Nano-topography was produced on PLGA by etching the scaffolds in NaOH. Results showed that etching PLGA with NaOH to create nano-scale surface features decreased fibroblast adhesion while it increased osteoblast adhesion; criteria critical for the spatial control of osteoblast and fibroblast adhesion for a successful enthesis tissue engineering material. Thus, the results of this study showed for the first time collective evidence that PLGA can be either treated with NaOH or not on ends of an enthesis tissue engineering construct to spatially increase osteoblast and fibroblast adhesion, respectively.
Introduction
The skeleton relies on the transmission of forces between muscles and bones to facilitate locomotion and support the body. A crucial junction involved in force transmission is the enthesis, which connects a ligament or tendon to bone (). Because the mechanical properties of ligaments and tendons differ substantially from those of the hard bone tissue into which they are inserted, the enthesis exhibits a strain gradient that reduces stress concentrations between the two tissues (CitationBenjamin and Ralphs 2001). The enthesis, the dense fibrous tissue stemming from the ligament or tendon, consists of: a tidemark that separates the dense fibrous cartilage tissue and the calcified fibrous tissue, the calcified cartilage, and bone tissue (). The most critical interface within the enthesis is between the calcified fibrous cartilage (occupied by fibroblasts) and the bone tissue (occupied by osteoblasts) since the differences in their mechanical properties are more extreme than at the other interfaces; the geometry at this junction is also highly irregular which contributes to its complexity (CitationBenjamin and Ralphs 2001). Enthesis avascularity also diminishes its ability to heal completely.
Figure 1 The natural enthesis demonstrating the necessary distribution of osteoblasts and fibroblasts. Tissue engineering scaffolds that are capable of separating these two cells are highly desirable for enthesis applications.
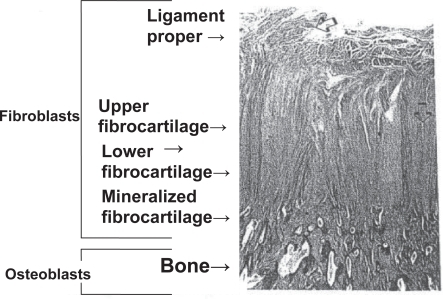
As a result, avulsions, tears, and fractures result in 100,000 surgical anterior cruciate ligament (ACL) reconstructions each year (CitationOwings 1998), many of which are at the enthesis. Instead of mimicking natural methods of soft tissue insertion into bone, current orthopedic repair methods often involve attaching soft allograft or autograft tissue, whose harvesting can lead to donor site morbidity in a manner that contributes to unmanageable stress concentrations, immunological responses and long recovery times (CitationGentleman et al 2006).
While considerable effort has led to the development of tissue-engineered ligaments and potential solutions to heal ligament damage (CitationYoung et al 1998; CitationGentleman et al 2006), few studies have explored the possibility of engineering a complete enthesis replacement (CitationKoike et al 2005). Ideally, a bioscaffold for enthesis repair would encourage the attachment, proliferation and differentiation of fibroblasts, chondrocytes, and osteoblasts and would eventually resorb, allowing normal, functional tissue to take its place. Poly (lactic-co-glycolic) acid (PLGA) is an FDA approved, bio-compatible, and biodegradable polymer that holds potential for enthesis regeneration. Bone cells respond well to porous PLGA scaffolds, suggesting that they can be used to study enthesis tissue engineering applications (CitationOchi et al 2003); however, improvements to PLGA can be made. For example, unmodified PLGA has no innate ability to spatially control fibroblast and osteoblast adhesion required for the creation of successful enthesis materials.
However, it is possible that the spatial adhesion of cells can be effectively influenced through the introduction of micro-scale porosity (CitationService 2000; CitationTienen et al 2005; CitationVitale-Brovarone et al 2005) with nano-scale surface features (CitationThapa et al 2003; CitationMiller et al 2004). Micron-scale porosity in natural orthopedic tissues (CitationService 2000; CitationTienen et al 2005; CitationVitale-Brovarone et al 2005) allows nutrients and cells, including fibroblasts (fibrous tissue forming cells), chondrocytes (cartilage forming cells) and osteoblasts (bone forming cells) to traverse the tissue and perform their respective functions. In addition, cells in natural tissue interact with each other and the extracellular matrix at the nano-scale (CitationThapa et al 2003; CitationMiller et al 2004). For example, integrins and cadherins (proteins that facilitate cell-extracellular matrix and cell-cell interactions, respectively) exert their influence at the nano-scale. Furthermore, ceramics and metals that possess nano-scale dimensions have increased the adhesion, proliferation, differentiation, mechanotransduction and matrix deposition activity by chondrocytes and osteoblasts (CitationWebster et al 1999; CitationWebster et al 2000; CitationMiller et al 2004; CitationPark et al 2005).
What has been less studied is how such cells respond to nanoscale dimensions on porous polymer scaffolds for enthesis applications. Since cells develop naturally in a three-dimensional extracellular matrix environment and polymers present a practical tissue engineering platform, it may be possible to selectively control enthesis cell behavior by altering biomaterial surface topography. Consequently, the present study focused on the adhesion of fibroblasts and osteoblasts to nano-patterned porous PLGA. In particular, we hypothesized that nano-topography would alter the adhesion of both cell types and serve as an initial step in improved bioscaffold development for the enthesis.
Materials and methods
Polymer modification
Porous PLGA scaffolds were fabricated by dissolving a 50/50 weight percent blend of poly (lactic-co-glycolic) acid (PLGA) (Polysciences, Warrington, PA, USA) in chloroform at 45 °C for 20 minutes. Subsequently, salt particles were added (150–250 μm diameter salt particles) to yield a 10:90 polymer:salt ratio by weight. The salt particles were thoroughly dispersed in the mixture by sonicating for 20 minutes. After sonication, the mixture was poured into a 50 mm diameter by 15 mm deep Teflon Petri dish to a depth of 2 mm, cured at room temperature overnight, and vacuum dried for 48 hours. The salt was leached by soaking the scaffolds in deionized water for 3 days, replacing the water every 3 hours. The scaffolds were cut into 5 mm by 10 mm pieces and divided into 2 groups. The first group of scaffolds was left unetched, exhibiting micron-scale topography. Scaffolds in the second group were etched in 1N NaOH for 10 minutes and then rinsed at least 3 times to remove excess etchant in order to generate nano-scale topography.
Porosity measurements
Scaffold porosity was determined by measuring the apparent density of the scaffolds and comparing it to the density of bulk PLGA. Three apparent volume measurements and a single mass measurement were taken on 6 samples each approximately 10 mm by 5 mm (n = 6). Scaffold mass was divided into average scaffold volume for each sample (n = 6) to calculate the apparent density (ρa) of the scaffolds. Scaffold porosity (φ) was calculated by comparing sample apparent density from each scaffold to the true density of PLGA (ρt) (EquationEq. 1). The porosities of the etched and unetched scaffolds were then compared to each other to determine statistical significance.
EquationEquation 1. Formula used to calculate scaffold porosity.
Cell culture
Previously characterized human skin fibroblasts (NIH 3T3) and human osteoblasts (ATCC CRL 11371) with population numbers ranging from 7 to 13 were cultured separately in 100 mm Petri dishes (Corning; Corning, NY, USA) in Dulbecco’s Modified Eagle Medium (DMEM, HyClone; Logan, UT, USA) with 10% fetal bovine serum (FBS) (HyClone; Logan, UT, USA) and 1% penicillin/streptomycin (P/S) under standard culture conditions of 37 °C in humidified 5% CO2/95% air. Culturing a suitable population for these experiments was accomplished by feeding the cells every three days and splitting the populations approximately every six days once the cells achieved confluence.
Cell adhesion assay
The PLGA scaffolds were sterilized by a 30 minute soak in EtOH and overnight UV exposure in a biosafety hood. The sterile scaffolds were affixed to the bottom of a 24 well culture dish (Corning; Corning, NY, USA) using sterile vacuum grease (Dow Corning, Midland, MI, USA). Fibroblasts and osteoblasts were seeded separately onto the NaOH etched and unetched porous PLGA scaffolds at a seeding density of 500,000 cells per scaffold in DMEM supplemented with 10% FBS and 1% P/S under standard cell culture conditions. After 4 hours, unattached cells were removed by aspirating the cell culture media and rinsing 3 times with phosphate buffer solution (PBS). The scaffolds were then moved to a new dish and covered with 2 mL of PBS. The cells were then lysed using three PBS freeze thaw cycles. After the final thaw, a 1-mL sample containing cell lysates from each scaffold was centrifuged at 250 g for 4 minutes. Next, 50 μl of the supernatant from this solution was placed into a 96 well plate to which 50 μl of substrate mix (CytoTox 96; Promega, Madison, WI, USA) was added and then incubated in darkness at 37 °C for 30 minutes. The reaction was arrested by adding 50 μl of stop solution (CytoTox, Promega, Madison, WI, USA) to each well and the light absorbance of the lysates was analyzed at 490 nm using a spectrophotometer (Thermo Electron Corp., Waltham, MA, USA) and Fluoroskan Ascent spectral analysis software (Thermo Electron Corp., Waltham, MA, USA). The number of cells was determined by comparing the absorbance to a calibration curve from cell lysates taken at predetermined cell numbers. An additional scaffold from each group was allocated for SEM analysis of the scaffolds.
Scanning electron microscopy analyses
Scanning electron microscopy (SEM) analysis of etched and unetched scaffolds was performed. Each specimen was critically-point dried in 100% EtOH in a CPD machine (LADD, Williston, VT, USA) and gold sputter coated using a sputter coater (LADD, Williston, VT, USA) in a 100 millitorr vacuum at 5 kV and 6 mA. Scanning electron microscopy (SEM) using a JEOL-840 microscope was performed on the samples under 5kV at several magnifications. Cell morphology on the samples of interest was also evaluated by SEM.
Statistical analyses
Using StatView (SAS Institute, Cary, NC, USA), an analysis of variance (ANOVA) was performed to compare the effects of etched versus unetched PLGA scaffolds on osteoblast and fibroblast adhesion. In addition, another ANOVA was performed in order to determine whether or not the etching process affected porosity. A significance level of 0.05 was used for all analyses.
Results
SEM analyses
SEM images of the unetched and etched porous PLGA scaffolds at 200X showed macro-porosity on both scaffolds and an increased general surface roughness on the NaOH-etched scaffold (). The unetched PLGA exhibited a smooth surface with micron scale pores (). In contrast, the NaOH etched PLGA had a rough, nano-surfaced topography but maintained micron pore sizes (). Pore sizes were 100 to 250 μm in diameter for both unetched and etched PLGA. Etched scaffold porosity (89.9% ± 1.4%) was significantly higher than that of the unetched scaffolds (86.7% ± 2.9%).
Figure 2 (A) 200 × SEM image of unetched PLGA scaffold showing micropores and a nano-smooth surface (Scale bar = 100 μm). (B) 200 × SEM image of NaOH etched PLGA showing micropores and a nano-rough surface (Scale bar = 100 μm). (C) 5000 × SEM image of unetched PLGA showing micron sized pores and a nano-smooth surface (Scale bar = 10 μm). (D) 5000 X SEM image of PLGA showing a nano-rough surface (Scale bar = 10 μm).
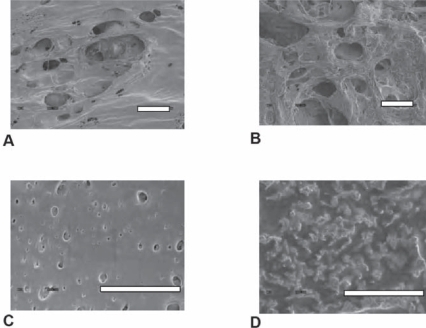
Cells adhesion study
Results of this study also showed that osteoblast adhesion was greater on NaOH etched PLGA scaffolds over unetched PLGA (). In contrast, the nanotopography produced on porous PLGA by etching significantly decreased fibro-blast adhesion compared to unetched scaffolds (). Similar to quantitative results, fibroblasts were more spread on unetched compared to etched PLGA ( and ).
Figure 3 (A) Osteoblast adhesion increased on NaOH-etched, nano PLGA scaffolds compared to unetched PLGA scaffolds (n = 15). (B) Fibroblast adhesion decreased on NaOH etched, nano PLGA scaffolds compared to unetched PLGA scaffolds (n = 3). *Cell adhesion on etched PLGA is significantly different from cell adhesion on unetched PLGA (p = 0.05).
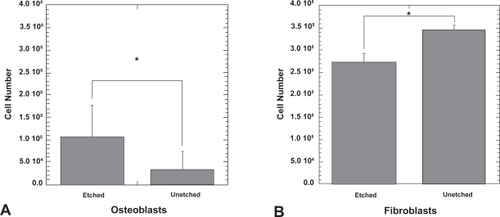
Discussion
The present in vitro study investigated the adhesion of osteoblasts and fibroblasts to unetched and NaOH etched porous PLGA scaffolds. NaOH treating of PLGA produced nano-scale topography (CitationThapa et al 2003) which also increased surface area (CitationThapa et al 2003) and porosity. Increased surface area creates more sites for protein adsorption and focal contact adhesion sites used by cells to anchor onto the polymer. Therefore, increased osteoblast adhesion on the etched (nano) scaffold was expected. The outcome of the osteoblast experiment also supported results from other studies suggesting that since cells interact naturally with nano-scale features in tissues of our body, surfaces with nano-topography will be better suited to cell activities such as adhesion (CitationWebster et al 1999; CitationWebster et al 2000; CitationWebster et al 2001; CitationThapa, Miller et al 2003; CitationThapa et al 2003; CitationWebster et al 2003; CitationMiller et al 2004; CitationPark et al 2005; CitationPattison et al 2005). In particular, scientists have pointed out that nano-phase materials may selectively adsorb vitronectin (CitationWoo et al 2003) to which osteoblasts have a high affinity (CitationWebster et al 2000). Nano etched PLGA may have adsorbed more vitronectin (a hydrophilic protein) since previous studies (CitationThapa et al 2003) have shown that when PLGA is treated with NaOH, ester bonds in PLGA are hydrolyzed to provide for a more hydrophilic surface.
Interestingly, what was not expected, was decreased fibroblast adhesion on NaOH etched, nanostructured PLGA scaffolds. As mentioned, vitronectin (being more hydrophilic, smaller and less dense than other proteins contained in serum such as fibronectin) may have altered adsorption rates which may have allowed it to saturate the etched, nano PLGA surface. In contrast, the lower surface energy of un-etched PLGA may not have been sufficient to preferentially adsorb vitronectin. This is important since fibroblasts have a greater initial affinity to adsorbed fibronectin than vitronectin (CitationSethi et al 2002); accordingly fibroblasts would adhere to fibronectin-rich unetched PLGA surfaces more readily than vitronectin-rich PLGA etched, nano versions. Importantly, although this is speculation at this point and more studies are needed to verify this, previously published reports support greater vitronectin adsorption on NaOH etched, nano PLGA scaffolds (CitationPark et al 2005).
In summary, the enthesis region is a composite tissue that consists of fibroblasts in the fibrocartilage region and osteoblasts in the boney region. Therefore, this study suggests that changes in surface topography and chemistry associated with NaOH etching of PLGA can be used in the design of composite scaffolds to spatially enhance cellular adhesion in distinct regions of an engineered enthesis. In particular, this study suggests that regions of PLGA for an enthesis tissue engineering application which supports fibroblast functions (the fibrocartilage region) should be left untreated while the region which supports osteoblast functions (the boney attachment region) should be treated with NaOH to create nanometer surface roughness.
Acknowledgements
The authors would like to thank Debbie Sherman and Chia Ping Huang for their assistance with SEM. The authors would also like to thank the George Washington Carver Fellowship for funding.
References
- American Academy of Orthopedic Surgeons2003Repair of anterior cruciate ligament (acl) tears [online]Accessed 4 July 2006. URL: www.aaos.org/wordhtml/research/stats/ACLRepairfacts.htm
- BenjaminMRalphsJR2001Entheses—the bony attachments of tendons and ligamentsItal J Anat Embryol1062 Suppl 1151711729950
- GentlemanELivesayGDeeK2006Development of ligament-like structural organization and properties in cell-seeded collagen scaffolds in vitroAnnals of Biomedical Engineering11117111210
- KoikeYTrudelGUhthoffHK2005Formation of a new enthesis after attachment of the supraspinatus tendon: A quantitative histologic study in rabbitsJournal of Orthopaedic Research2361433144015913944
- MillerDCThapaAHaberstrohKM2004Endothelial and vascular smooth muscle cell function on poly(lactic-co-glycolic acid) with nano-structured surface featuresBiomaterials251536114580908
- OchiKChenGUshidaT2003Use of isolated mature osteoblasts in abundance acts as desired-shaped bone regeneration in combination with a modified poly-dl-lactic-co-glycolic acid (PLGA)-collagen spongeJ Cell Physiol1941455312447988
- OwingsMKL1998Ambulatory and inpatient procedures in the United StatesVital Health Stat13119
- ParkGEPattisonMAParkK2005Accelerated chondrocyte functions on naoh-treated PLGA scaffoldsBiomaterials261630758215603802
- PattisonMAWursterSWebsterTJ2005Three-dimensional, nano-structured plga scaffolds for bladder tissue replacement applicationsBiomaterials2615249150015585251
- ServiceRF2000Tissue engineers build new boneScience28954841498150010991738
- SethiKKYannasIVMuderaV2002Evidence for sequential utilization of fibronectin, vitronectin, and collagen during fibroblast-mediated collagen contractionWound Repair and Regeneration10639740812453144
- ThapaAMillerDCWebsterTJ2003Nano-structured polymers enhance bladder smooth muscle cell functionBiomaterials241729152612742731
- ThapaAWebsterTJHaberstrohKM2003Polymers with nano-dimensional surface features enhance bladder smooth muscle cell adhesionJ Biomed Mater Res A67413748314624525
- The Center for the Orthopedics and Sports Medicine2006The center for the orthopedics and sports medicine, arthroscopic acl (surgery) reconstruction [online]http://www.arthroscopy.com/sp05018.htm
- TienenTGHeijkantsRGJCde GrootJH2005Replacement of the kneeAm J Sports Med34647116260465
- Vitale-BrovaroneCVerneEBosettiM2005Microstructural and in vitro characterization of SiO O-Cao-MgO glass-ceramic bioactive scaffolds for bone substitutesJournal of Materials Science: Materials in Medicine161090991716167099
- WebsterTJErgunCDoremusRH2003Increased osteoblast adhesion on titanium-coated hydroxylapatite that forms CaTiO3J Biomed Mater Res A6739758014613247
- WebsterTJErgunCDoremusRH2000Specific proteins mediate enhanced osteoblast adhesion on nanophase ceramicsJ Biomed Mater Res5134758310880091
- WebsterTJSchadlerLSSiegelRW2001Mechanisms of enhanced osteoblast adhesion on nanophase alumina involve vitronectinTissue Eng7329130111429149
- WebsterTJSiegelRWBiziosR1999Osteoblast adhesion on nanophase ceramicsBiomaterials20131221710395391
- WooKMChenVJMaPX2003Nano-fibrous scaffolding architecture selectively enhances protein adsorption contributing to cell attachmentJournal of Biomedical Materials Research Part A67A2531714566795
- YoungRGButlerDLWeberW1998Use of mesenchymal stem cells in a collagen matrix for achilles tendon repairJournal of Orthopaedic Research164406139747780