Abstract
The use of needles for multiple injection of drugs, such as insulin for diabetes, can be painful. As a result, prescribed drug noncompliance can result in severe medical complications. Several noninvasive methods exist for transdermal drug delivery. These include chemical mediation using liposomes and chemical enhancers or physical mechanisms such as microneedles, iontophoresis, electroporation, and ultrasound. Ultrasound enhanced transdermal drug delivery offers advantages over traditional drug delivery methods which are often invasive and painful. A broad review of the transdermal ultrasound drug delivery literature has shown that this technology offers promising potential for noninvasive drug administration. From a clinical perspective, few drugs, proteins or peptides have been successfully administered transdermally because of the low skin permeability to these relatively large molecules, although much work is underway to resolve this problem. The proposed mechanism of ultrasound has been suggested to be the result of cavitation, which is discussed along with the bioeffects from therapeutic ultrasound. For low frequencies, potential transducers which can be used for drug delivery are discussed, along with cautions regarding ultrasound safety versus efficacy.
Introduction
Several methods exist for improving transdermal drug delivery such as chemical mediation using liposomes or chemical enhancers, and physical mechanisms such as iontophoresis, lasers, electroporation, microneedles and ultrasound (also called sonophoresis or phonophoresis) (CitationPrausnitz 1997; CitationPrausnitz 1999; CitationMontorsi et al 2000; CitationWang et al 2005; CitationNanda et al 2006). Numerous studies of these methods have shown that ultrasound mediated transdermal drug delivery offers promising potential for noninvasive drug administration. However the broad literature on ultrasound drug delivery is not confined only to transdermal applications. A large body of work focuses specifically on delivery to internal organs, but does not cover tissues or gene delivery. Additionally for transdermal work, the reader is also directed to several well written articles for further reading and additional viewpoints on this topic (CitationMitragotri and Kost 2004; CitationPitt et al 2004; CitationBrown et al 2006).
Passive drug delivery across the stratum corneum can be transported with molecules that have a weight less than 500 Da (CitationBrown et al 2006). In general the stratum corneum, which varies in thickness (≈ 10–20 μm) depending on the body location, forms the barrier to drug diffusion. This low permeability is attributed to the outermost skin layer that consists of a compact and organized structure of cells named keratinocites surrounded by lipid bilayers. Ultrasound enhanced transdermal drug delivery offers advantages over traditional injection drug delivery methods which are invasive and painful. Currently few drugs, proteins or peptides have been successfully administered transdermally for clinical applications because of the low skin permeability to these relatively large molecules. However from a research viewpoint, the list of compounds which have been shown to transdermally cross skin via ultrasound is ever increasing. One hypothesis indicates that once the drug has traversed the stratum corneum, the next layer is easier to cross and subsequently the drug can reach the capillary vessels to be absorbed (CitationMitragotri et al 1995b).
Background on ultrasound delivery and biological effects
One of the earliest reports of the interaction between ultrasound and biological tissue can be traced back to the 1920s and the post-World War I experiments of Professor Paul Langévin (CitationGraff 1981). Much of his work centered on the development of pulse-echo sonar for submarine detection, including the observation of bubble formation in the water and the killing of fish from the sound beam. Specific biological changes such as searing of skin, rupturing of red blood cells and lethal effects on mammals were observed by Wood and Loomis with 200–500 kHz high intensity ultrasound (CitationWood and Loomis 1927).
In contrast to ultrasound used for diagnostic imaging, therapeutic ultrasound can be described as a controlled sound wave intended for biological interaction for a curative benefit. Some of the first clinically significant application of this technology was developed by Francis and William Fry in the 1950s (CitationFry et al 1954; CitationFry 1954). Their work was applied to the development of ultrasound devices for noninvasive surgical treatment of neurological disorders including Parkinson disease. Results included both reversible and irreversible biological effects. Other early clinical applications at this same time include the treatment of arthritis using ultrasound with hydrocortisone to relieve pain (CitationFellinger and Schmid 1954).
Thus it is appropriate to divide therapeutic ultrasound into two categories which use ‘low’ intensities and ‘high’ intensities (>5 W/cm2) though this division is not precise (Citationter Haar 1999). High intensity focused ultrasound (HIFU) is an attractive means of noninvasive coagulation of deep tissues using external sources. Current clinical applications of ultrasound include transducer and array designs for the treatment of prostate cancer (CitationGelet et al 2004; Saleh and Smith 2004), liver tumors (CitationLi et al 2004; CitationGignoux et al 2003), breast cancer (CitationJenne et al 2003; CitationWu et al 2004), bladder tumors (CitationWang et al 2003; CitationMadersbacher and Marberger 2003) and uterine fibroids (Chan et al 2002; CitationTempany et al 2003). Low intensity ultrasound has been shown to mediate the transport of drugs across the skin and is clinically used in physical therapy to accelerate or stimulate a normal biological response through deep heating.
Many recent reviews have shown that ultrasound mediated transdermal drug delivery offers promising potential for noninvasive drug administration (CitationTachibana and Tachibana 2001; CitationPitt et al 2004; CitationMitragotri 2005). The working principle of sonophoresis, although not completely understood, has been suggested to be the result of cavitation (CitationMitragotri et al 1995b, Citation1997) although thermal effects can not be entirely discounted. Low frequency ultrasound is capable of generating microbubbles in the water and tissue. Because sound energy is transmitted in a fluid media, the large negative pressures cause violent collapse in bubbles, which results in the formation of water channels within the lipid bilayers. The resulting disorder created in the stratum corneum facilitates the crossing of a hydrophilic drug or molecule.
sets out an overview of the transdermal ultrasound mediated drug delivery literature from the past several years with some commentary on notable contributions. Though not complete, the table lists the delivered compound or drug, its respective molecular weight (Daltons) and the experimental sample (animal) preparation including whether the experiment was performed under in vivo or in vitro conditions. Of major interest is the ability to reduce the research to clinical practice and therefore the reported ultrasound frequency and device is listed. For the table, ultrasound devices can be classified into different categories. The commercially made devices include sonicators (≈20 kHz), ultrasound heating devices intended for therapy (>1 MHz) and pre-fabricated transducers. Very few of the ultrasound devices listed are specifically designed for drug delivery and originally, like the sonicator, had a different intended purpose.
Table 1 A list of transdermally delivered drugs and compounds using ultrasound devices
Many previous investigators using sonophoresis have found enhanced transdermal drug delivery over various frequency ranges using commercial sonicators or therapeutic devices. For example, a 14-fold increase of corticosterone transport has been shown using a 1 MHz therapeutic product used for the treatment of chronic and acute pain (Sonopuls® 463, Sugarland, TX) operating at 1.4 W/cm2 for 24 hours (CitationJohnson et al 1996). Significant transdermal transport of model drugs such as mannitol and inulin (plant starch) has been observed using a 20 kHz commercial sonicator (VCX400, Sonics and Materials, Newtown, CT) (CitationLevy et al 1989). Previous work with high frequency ultrasound (≈1 MHz and at 1–3 W/cm2) to enhance transdermal drug delivery has produced interesting results, which varied from drug to drug (Bommannan et al 1992a, 1992b; CitationMitragotri et al 1997). Pulsed ultrasound at 1 MHz has been used to increase transdermal absorption of indomethacin from an ointment in rats (CitationAsano et al 1997). A combination of chemical enhancers and therapeutic ultrasound (1 MHz, 1.4 W/cm2, continuous wave (CW)) in transdermal drug transport has also been investigated with success (CitationJohnson et al 1996).
A noteworthy difference between high (1–3 MHz) and low (≈20 kHz) frequency ultrasound appears to be that low frequency ultrasound enhances transdermal drug transport 1000 times more than high frequency ultrasound (CitationMitragotri et al 1996). The working hypothesis for the physical mechanism is that low-frequency ultrasound enhances transdermal transport through aqueous channels in the stratum corneum generated by cavitation induced bilayer disordering. However, the mechanism of the enhancement using ultrasound is far from being fully understood (CitationPitt et al 2004). Some researchers have concluded that at 168 kHz using CW ultrasound and at 1.9* × 105 Pa, a new structural state was induced which generated defects in human stratum corneum specimens. They suggest that the dimensions of the defects (20 μm) were large enough to allow the transdermal passage of high molecular weight drug molecules, which normally elude the unenhanced transdermal drug delivery (CitationWu et al 1998).
Past research has demonstrated the possibility of delivering and controlling therapeutic doses of proteins such as interferon gamma and erythropoeitin across human skin using ultrasound (CitationMitragotri et al 1995a). Other researchers have investigated the in vitro penetration and the in vivo transport of flufenamic acid in skin with ultrasound (CitationHippius et al 1998). In the flufenamic acid study, ultrasound exposure was from 5–30 minutes with intensities up to 1.5 W/cm2. Although there was a pronounced effect of ultrasound on the transmembrane absorption of the drug, there was also a rise in temperature up to 4.5 °C. Ultrasound at 1 MHz has also been used to enhance the transdermal absorption of indomethacin studied in rats using intensities from 0.25–1 W/cm2. The researchers reported no significant skin temperature rise and no notable damage to the skin, although damage was noted as the intensity and the time of application of ultrasound increased beyond 1 W/cm2 (CitationMiyazaki et al 1992).
Other researchers have reported noticeable skin damage from ultrasound transdermal drug delivery experiments (CitationWu et al 1998). One group has examined the morphological changes induced in in vitro hairless mouse skin and human skin after ultrasound exposure to transdermal drug delivery systems. The skins were immersed in a commercial ultrasound water tank at 48 kHz and an intensity of 0.5 W/cm2. Skins were compared to control skins under a scanning electron microscope. The researchers found that cells of the stratum corneum of the mouse skin surface were almost completely removed. Furthermore, on some of the the mouse samples, large craterlike pores with a diameter of 100 microns were formed sporadically. However in human skin, the surface exposed to ultrasound showed only slight removal of keratinocytes around the hair follicles. The researchers suggested that the removal of the stratum corneum and other alterations in hairless mouse and human skin may explain the enhancement of transdermal drug penetration (CitationYamashita et al 1997).
Convenient noninvasive methods for transdermal delivery of insulin or similar procedures for glucose sensing has particular public interest due to the increasing problem of diabetes. In the United State alone, approximately 16 million people suffer from diabetes mellitus. From a human and economic perspective, it is one of the most costly diseases (Congressionally Established Diabetes Research Working Group 1999; CitationThe Whitaker Foundation 2004). Management of diabetes often requires painful repetitive blood glucose tests and insulin injections up to three or four times each day. Between injections, blood sugar levels can fluctuate and remain out of balance until the next test or injection, increasing the risk of tissue or organ damage.
Specifically for insulin (), the amount of research for noninvasive insulin delivery is increasing every year. Over a frequency range of 20–105 kHz, enhanced transport in the presence of ultrasound has been shown in both in vitro and in vivo experiments. Many early experiments were performed using either an ultrasound sonicator, ultrasonic bath or commercial transducer. For example investigators have demonstrated effective in vivo transport of insulin at 48 kHz using an ultrasonic bath (CitationTachibana and Tachibana 1991) and at 105 kHz (CitationTachibana 1992) using a commercially obtained transducer. The major drawback so far in exploiting ultrasound for noninvasive drug delivery is the large size and poor mobility of the ultrasound device. Commercial sonicators are large, heavy, table-top devices specifically designed for lysis of cells, catalyzing reactions, creating emulsions or cleaning. A few commercial ultrasound devices do exist, specifically designed for ultrasound drug delivery, such as the SonoPrep® made by the Sontra Medical Corporation (Cambridge MA), which is a large ultrasound device that consists of a power control unit and a hand-held applicator.
Although diabetics have an aversion to injecting insulin they probably hate the more frequent finger-stick for blood glucose samples even more. A number of different techniques for monitoring blood glucose using non-invasive or minimally invasive methods are under investigation including near-infrared spectroscopy, implantable glucose sensors, reverse sonophoresis, reverse iontophoresis and interstitial fluid sampling devices (CitationKost 2002). The latter three techniques extract glucose transdermally and measure glucose in interstitial fluid. Dermal interstitial fluid glucose concentration is highly correlated with the plasma glucose concentration and capillary blood glucose concentration. Thus transdermal extraction of interstitial fluid offers a noninvasive method of obtaining a sample for blood glucose measurements (CitationCantrell et al 2000; CitationKost 2002). Previous results has demonstrated positive results in the use of ultrasound to facilitate the noninvasive extraction of interstitial skin fluids (ISF) for blood glucose monitoring through an electrolytic reaction with glucose sensitive enzymes (CitationKost et al 2000).
Mechanism of ultrasound
Although ultrasound is known to increase transdermal protein delivery (CitationTachibana 1992; CitationMitragotri et al 1995a) the mechanisms of this enhanced transport have not been fully characterized (CitationPitt et al 2004). Bioeffects from ultrasound include the thermal or mechanical (cavitation) mechanism (CitationAIUM 2000). One effect of cavitating ultrasound is its ability to increase permeability of the outer skin layer (stratum corneum), which is thought to be a primary barrier to protein diffusion. Cavitation represents the rapid expansion and collapse of gaseous bubbles in response to an alternating pressure field. Cavitation types can be broken into two non-exclusive categories (CitationFlynn 1982). The first is stable cavitation where the cavity oscillates about its equilibrium radius in response to relatively low acoustic pressures. The second is transient cavitation (also known as inertial cavitation) whereby the equilibrium size varies greatly in response to very few acoustic cycles. During transient cavitation, the rapid, violent collapse of bubbles is associated with high acoustic pressures and temperatures of the order of 1000–2000 K (CitationApfel 1981, Citation1986). Transient cavities are generated in response to high acoustic pressures and/or lower frequencies. The violent hydrodynamic forces () due to a collapsing bubble can cause severe damage within biological media. Indeed free radicals can be produced by this violent phenomena (CitationEdmonds and Sancier 1983; CitationMason and Lorimer 1988).
Figure 1 With transient cavitation the bubble dynamics have two basics stages comprising the initial formations of the cavity followed by the growth and asymmetric violent collapse. The photo shows the jet produced by the collapse of a cavitation bubble at a liquid-solid interface. [Photo courtesy of Dr. Lawrence Crum at the University of Washington.]
![Figure 1 With transient cavitation the bubble dynamics have two basics stages comprising the initial formations of the cavity followed by the growth and asymmetric violent collapse. The photo shows the jet produced by the collapse of a cavitation bubble at a liquid-solid interface. [Photo courtesy of Dr. Lawrence Crum at the University of Washington.]](/cms/asset/a0b38d6b-0f5f-4e80-ab4e-63e4f7e108ce/dijn_a_24585_f0001_b.jpg)
There exists several definitions for the threshold for transient cavitation in terms of such physical parameters as acoustic pressure, frequency or bubble radius. For diagnostic ultrasound, one (of many) definitions of the cavitation onset is the peak rarefractional pressure divided by the square root of the frequency (CitationApfel and Holland 1991). From previous research, the measured cavitation pressure amplitude in dog thigh muscle in vivo was found to depend linearly on frequency with a slope of 5.3 MPa/MHz (CitationHynynen 1990). Compared to the kilohertz range, ultrasound in the megahertz range also produces cavitation although much higher pressures are required to exceed the cavitation threshold. Beyond the threshold, cavitation has been shown to disrupt cells and damage tissue (CitationDalecki et al 1996; CitationMiller et al 1996). Mechanical bioeffects in tissues with gas bodies include lung hemorrhage in mice, rats, monkeys and pigs (CitationAIUM 2000). Cavitation has also been shown with diagnostic ultrasound levels (CitationApfel 1986; CitationRoy et al 1990) which has motivated the introduction of the mechanical index to identify a threshold pressure for the onset of inertial cavitation (CitationAIUM-NEMA 1996). Even in the absence of well-defined gas bodies, there exist non-thermal bioeffects due to ultrasound, which are known to occur in the absence of excessive heating or evidence of cavitation bubbles. In this situation, the mechanism is in the form of radiation force or torque or acoustic streaming (CitationBeyer 1997).
The dynamics of acoustic cavitation in liquid alone differ considerably to cavitation at liquid-solid interfaces. Ultimately acoustic bubble dynamics are quite complex and beyond this overview (CitationApfel 1981; CitationLeighton 1994; CitationHamilton and Blackstock 1998). Determining the threshold and energy from a cavitation event is difficult under the best conditions. Researchers in ultrasound try to follow three experimental rules with respect to cavitation: understand the liquid including impurities, understand the sound field, and know when something happens (CitationApfel 1981). The first rule refers to the cavitation threshold while the second rule relates to accurate measurements of the acoustic field. The third relates to observable cavitation events or secondary related information. Rule two deals with a commentary regarding information which could have been included in but was intentionally omitted. Though many of the papers listed in report an ultrasound intensity, the drawback is that much of the literature gives a value but does not specify details of the exposimetry such as spatial (average, peak) or temporal (average, peak) values. To report the determination of an acoustic field, it is essential to supply enough information, such as calibrated hydrophones, dissolved gas concentration, anechoic conditions, etc., so that intensity experiments can be repeated by others. Therefore accurate and precise evaluation of acoustic fields should follow exposimetry and dosimetry procedures previously recognized in the ultrasound literature (CitationSchafer et al 1990; CitationLewin and Ziskin 1992; CitationAIUM-NEMA 1996; CitationLewin et al 2003). Without such information, comparing the intensity of enhanced transport between many of the drug delivery publications, or determining potential bioeffects, is impossible.
Future of transdermal drug delivery
Use of transdermal drug delivery techniques has the most practical clinical application for medications which need to be injected multiple times either daily or weekly. Though infrequent, other injectable drug avoidance situations could include the use of needles on infants, children and pets or under harsh conditions (battlefield or first responder) where needles are not feasible. As seen in , many previous researchers who have successfully used acoustic energy for drug delivery have used commercial sonicators or off-the-shelf transducers. These large industrial devices are impractical for a feasible and transportable drug delivery device. Much of the previous ultrasound transdermal dry delivery research has focused on low frequencies primarily because commercial sonicators were designed to operate only at one frequency. To bring this research to clinical practice will require more investigation into the optimal frequency and intensity of each particular drug. As with diagnostic ultrasound imaging, drug delivery using ultrasound requires a delicate balance between safety and efficacy and requires careful scientific study.
Other recent reviews on drug delivery state similar views to those expressed here, for example, “small-sized low-frequency transducers need to be developed so that patients can wear them” (CitationPitt et al 2004). Although there are several possible low frequency transducer designs that can be used in a drug delivery application, such as the low frequency flextensional resonators (CitationStansfield 1990), tonpilz transducers (CitationWilson 1988), or “thickness”-type resonators (CitationShung et al 1992), the “cymbal” transducer design is a good choice for a portable device. This Class V flextensional transducer has a thickness of less than 2 mm, weighs less than 3 g, resonates between 1 and 100 kHz depending on geometry, and has a large scale manufacturing cost of less than $5.00/unit (CitationNewnham et al 1991, Citation1994; CitationDogan et al 1997; Newnham, 1998; CitationTressler et al 1998). With the low profile cymbal design, high frequency radial motions of the ceramic translates into low frequency displacement motions through the cap covered cavity. If the diameter of the ceramic is increased (ie, a larger single element), then the frequency of the transducer decreases towards a lower range. If the diameter increased, the capsule depth of the flextensional design also needs to increase thereby increasing the thickness and slightly increasing the profile. Cymbals can be arranged into multi-element array designs () since this can increase the effective aperture of ultrasound area with respect to skin area. Some research indicates that the delivery dose increases with ultrasound exposure area (CitationSmith et al 2003). Interestingly the cymbal design originates from underwater research for naval applications and current research is underway to incorporate existing battery technology in the miniaturization of portable power for both insulin delivery and glucose sensing (CitationLee et al 2004, Citation2005).
Figure 2 The future of practical noninvasive drug delivery may be in the use of novel transducers or ceramics for producing ultrasound. One example is the cymbal transducer made of piezoelectric material PZT-4 operated at a frequency of 20 kHz. The light-weight, low-profile array was constructed using cymbal transducers which were connected in parallel, and encased in URALITE® polymer. The dimensions of the array were 37 × 37 × 2 mm3 and is comparable in size to a US quarter; it weighed less than 22 g.
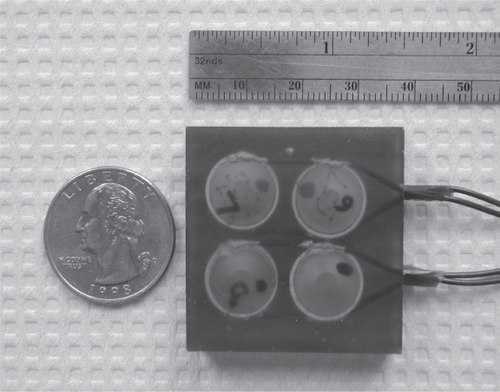
In general the future for noninvasive drug delivery is encouraging. Exploiting transdermal ultrasound drug delivery beyond the feasibility stage will require the cooperation of medical doctors and engineers so that the technology aids the construction of a clinical device. As with diagnostic ultrasound, the bioeffects and safety of each device needs to be carefully monitored, because it will not matter how much of any drug can be transported if the skin is burned, damaged or the procedure is painful.
Acknowledgements
This work was supported by the Department of Defense Technologies for Metabolic Monitoring Award Number W81XWH-05-1-0617.
Reference
- AIUM2000Mechanical bioeffects from diagnostic ultrasound: AIUM consensus statementsJ Ultrasound in Medicine1968168
- AIUM-NEMA1996Standard for real-time display of thermal and mechanical acoustic output indices on diagnostic ultrasound equipmentLaurel, MDAmerican Institute of Ultrasound in Medicine National Electrical Manufacturers Association
- ApfelRE1981Acoustic Cavitation (chapter)Methods of experimental physicsEdmondsPDNew YorkAcademic Press
- ApfelRE1986Possibility of microcavitation from diagnostic ultrasoundIEEE Trans Ultrason Ferroelectr Freq Contr UFFC-3313942
- ApfelREHollandCK1991Gauging the likelihood of cavitation from short-pulse, low duty cycle diagnostic ultrasoundUltrasound Med Biol17179852053214
- AsanoJSuishaFTakadaM1997Effect of pulsed output ultrasound on the transdermal absorption of indomethacin from an ointment in ratsBiol Pharm Bull20288919084890
- BeyerRT1997Nonlinear AcousticsSewickley, PAAcoustical Society of America Publications
- BommannanDMenonGKOkuyamaH1992Sonophoresis. II. Examination of the mechanism(s) of ultrasound-enhanced transdermal drug deliveryPharm Res9104371409375
- BommannanDOkuyamaHStaufferP1992Sonophoresis. I. The use of high-frequency ultrasound to enhance transdermal drug deliveryPharm Res9559641495903
- BoucaudAGarrigueMAMachetL2002Effect of sonication parameters on transdermal delivery of insulin to hairless ratsJ Control Release81113911992684
- BoucaudAMachetLArbeilleB2001In vitro study of low-frequency ultrasound-enhanced transdermal transport of fentanyl and caffeine across human and hairless rat skinInt J Pharm228697711576769
- BoucaudATessierLMachetLTransdermal delivery of insulin using low frequency ultrasoundProceedings of the IEEE 2000 Ultrasonics SymposiumSan Juan Porto Rico200014536
- BrownMBMartinGPJonesSA2006Dermal and transdermal drug delivery systems: current and future prospectsDrug Deliv131758716556569
- CagnieBVinckERimbautS2003Phonophoresis versus topical application of ketoprofen: comparison between tissue and plasma levelsPhys Ther Aug8370712
- CancelLMTarbellJMBen-JebriaA2004Fluorescein permeability and electrical resistance of human skin during low frequency ultrasound applicationJ Pharm Pharmacol5611091815324479
- CantrellJTMcArthurMJPishkoMV2000Transdermal extraction of interstitial fluid by low-frequency ultrasound quantified with 3H2O as a tracer moleculeJ Pharm Sci891170910944382
- DaleckiDRaemanCHChildSZ1996A test for cavitation as a mechanism for intestinal hemorrhage in mice exposed to a piezoelectric lithotripterUltrasound Med Biol2249368795176
- DarrowHSchulthiesSDraperD1999Serum Dexamethasone Levels After Decadron PhonophoresisJ Athl Train343384116558583
- DoganAUchinoKNewnhamRE1997Composite piezoelectric transducer with truncated conical endcaps “cymbal”IEEE Trans Ultrason, Ferroelect, Freq Contr44597605
- EdmondsPDSancierKM1983Evidence for free radical production by ultrasonic cavitation in biological mediaUltrasound Med Biol963596322402
- FellingerKSchmidJKlinikAN1954Therapie des Chronischen (transl. Clinical experience/practise about the therapy of the chronic (illness)Gelenkreumatismus (transl Articular Rheumatism)54952
- FlynnHG1982Generation of transient cavities in liquids by microsecond pulses of ultrasoundJ Acoust Soc Amer72192632
- FryWJ1954Intense ultrasound: a new tool for neurological researchJ Ment Sci100859613152523
- FryWJMosbergWBarnardJW1954Production of focal destructive lesions in the central nervous system with ultrasoundJ Neurosurg11471813201985
- GeletAChapelonJYPoissonnierL2004Local recurrence of prostate cancer after external beam radiotherapy: early experience of salvage therapy using high-intensity focused ultrasonographyUrology63625915072864
- GignouxBMScoazecJYCurielL2003High intensity focused ultrasonic destruction of hepatic parenchymaAnn Chir128182512600324
- GraffKFMasonWPThurstonRN1981A History of Ultrasonics
- HamiltonMBlackstockD1998Nonlinear acousticsSan Diego, CAAcademic Press
- HippiusMUhlemannCSmolenskiU1998In vitro investigations of drug release and penetration – enhancing effect of ultrasound on transmembrane transport of flufenamic acidInt J Clin Pharmacol Ther36107119520159
- HsiehYL2006Effects of ultrasound and diclofenac phonophoresis on inflammatory pain relief: suppression of inducible nitric oxide synthase in arthritic ratsPhys Ther86394916386061
- HynynenKH1990The threshold for thermally significant cavitation in dog’s thigh muscle in vivoUltrasound Med Biol17157692053212
- JenneJWDivkovicGRastertR2003Focused ultrasound surgery. Basics, current status, and new trendsRadiologe438051214605696
- JohnsonMEMitragotriSPatelA1996Synergistic effects of chemical enhancers and therapeutic ultrasound on transdermal drug deliveryJ Pharm Sci8567098818988
- KoekePUParizottoNACarrinhoPM2005Comparative study of the efficacy of the topical application of hydrocortisone, therapeutic ultrasound and phonophoresis on the tissue repair process in rat tendonsUltrasound Med Biol313455015749557
- KostJ2002Ultrasound-assisted insulin delivery and noninvasive glucose sensingDiabetes Technol Ther44899712396743
- KostJMitragotriSGabbayRA2000Transdermal monitoring of glucose and other analytes using ultrasoundNat Med63475010700240
- KostJPliquettUMitragotriS1996Synergistic effect of electric field and ultrasound on transdermal transportPharm Res1363388710759
- KozanogluEBasaranSGuzelR2003Short term efficacy of ibuprofen phonophoresis versus continuous ultrasound therapy in knee osteoarthritisSwiss Med Wkly133333812923684
- KushnerJBlankschteinDLangerR2004Experimental demonstration of the existence of highly permeable localized transport regions in low-frequency sonophoresisJ Pharm Sci9327334515389675
- LeeSNayakVDoddsJ2005Ultrasonic mediated glucose measurements in vivo using the cymbal arrayUltrasound Med Biol319717715972203
- LeeSNewnhamRESmithNB2004Short Ultrasound Exposure Times for Noninvasive Insulin Delivery in Rats using the Light Weight Cymbal ArrayIEEE Transactions on Ultrasonics, Ferroelectrics and Frequency Control5117680
- LeeSSnyderBNewnhamRE2004Noninvasive ultrasonic trans-dermal insulin delivery in rabbits using the light-weight cymbal arrayDiabetes Technol Ther68081515684633
- LeightonT1994Acoustic BubbleSan Diego, CAAcademic Press
- LevyDKostJMeshulamY1989Effect of ultrasound on transdermal drug delivery to rats and guinea pigsJ Clin Invest83207482498396
- LewinPZiskinM1992Ultrasonic ExposimetryBoca Raton, FLCRC Press
- LewinPABarrie-SmithNIdeM2003Interlaboratory acoustic power measurementJ Ultrasound Med222071312562125
- LiCXXuGLJiangZY2004Analysis of clinical effect of high-intensity focused ultrasound on liver cancerWorld J Gastroenterol1022010415259065
- MadersbacherSMarbergerM2003High-energy shockwaves and extracorporeal high-intensity focused ultrasoundJ Endourol176677214622487
- MasonTJLorimerJP1988Sonochemistry: Theory, Applications and Uses of Ultrasound in ChemistryWest Sussex, U.KEllis Horwood Limited
- MerinoGKaliaYNgado-CharroMB2003Frequency and thermal effects on the enhancement of transdermal transport by sonophoresisJ Control Release88859412586506
- MillerMWMillerDLBraymanAA1996A review of in vitro bioeffects of intertial ultrasonic cavitation from a mechanistic perspectiveUltrasound Med Biol221131549123638
- MitragotriS2005Healing sound: the use of ultrasound in drug delivery and other therapeutic applicationsNat Rev Drug Discov42556015738980
- MitragotriSBlankschteinDLangerR1995Ultrasound-mediated trans-dermal protein deliveryScience26985037638603
- MitragotriSBlankschteinDLangerR1996Transdermal drug delivery using low-frequency sonophoresisPharm Res13411208692734
- MitragotriSBlankschteinDLangerR1997An explanation for the variation of the sonophoretic transdermal transport enhancement from drug to drugJ Pharm Sci86119029344179
- MitragotriSColemanMKostJ2000Analysis of ultrasonically extracted interstitial fluid as a predictor of blood glucose levelsJ Appl Physiol89961610956339
- MitragotriSColemanMKostJ2000Transdermal extraction of analytes using low-frequency ultrasoundPharm Res174667010870992
- MitragotriSEdwardsDABlankschteinD1995A mechanistic study of ultrasonically-enhanced transdermal drug deliveryJ Pharm Sci846977067562407
- MitragotriSFarrellJTangT2000Determination of the threshold energy dose for ultrasound-induced transdermal drug transportJ Control Release63415210640579
- MitragotriSKostJ2000Low-frequency sonophoresis: a noninvasive method of drug delivery and diagnosticsBiotechnol Prog164889210835253
- MitragotriSKostJ2004Low-frequency sonophoresis: a reviewAdv Drug Deliv Rev5658960115019748
- MiyazakiSMizuokaHKohataY1992External control of drug release and penetration. VI. Enhancing effect of ultrasound on the transdermal absorption of indomethacin from an ointment in ratsChem Pharm Bull402826301464116
- MontorsiFSaloniaAGuazzoniG2000Transdermal electromotive multi-drug administration for Peyronie’s disease: preliminary resultsJ Androl21859010670523
- MorimotoYMutohMUedaH2005Elucidation of the transport pathway in hairless rat skin enhanced by low-frequency sonophoresis based on the solute-water transport relationship and confocal microscopyJ Control Release1035879715820406
- MutohMUedaHNakamuraY2003Characterization of transdermal solute transport induced by low-frequency ultrasound in the hairless rat skinJ Control Release921374614499192
- NandaANandaSGhilzaiNM2006Current developments using emerging transdermal technologies in physical enhancement methodsCurr Drug Deliv32334216848725
- NewnhamREXuQCYoshikawaSinventors. 1991Transformed stress direction acoustic transducer. 4,999,819.
- NewnhamREXuQCYoshikawaSinventors. 1994Metal-electroactive ceramic composite actuators. 5,276,657.
- PaliwalSMenonGKMitragotriS2006Low-frequency sonophoresis: ultrastructural basis for stratum corneum permeability assessed using quantum dotsJ Invest Dermatol126109510116528354
- ParkSRJangKWParkSH2005The effect of sonication on simulated osteoarthritis. Part I: effects of 1 MHz ultrasound on uptake of hyaluronan into the rabbit synoviumUltrasound Med Biol311551816286032
- PittWGHusseiniGAStaplesBJ2004Ultrasonic drug delivery – a general reviewExpert Opin Drug Deliv1375616296719
- PrausnitzMR1997Reversible skin permeabilization for transdermal delivery of macromoleculesCrit Rev Ther Drug Carrier Syst14455839450177
- PrausnitzMR1999A practical assessment of transdermal drug delivery by skin electroporationAdv Drug Deliv Rev35617610837689
- RosimGCBarbieriCHLancasFM2005Diclofenac phonophoresis in human volunteersUltrasound Med Biol313374315749556
- RoyRAMadanshettySIApfelRE1990An acoustic backscattering technique for the detection of cavitation produced by microsecond pulses of ultrasoundJ Acoust Soc Amer87245182373791
- SalehKYSmithNB2005Two-dimensional ultrasound phased array design for tissue ablation for treatment of benign prostatic hyperplasiaInt J Hyperthermia2073114612311
- SantoianniPNinoMCalabroG2004Intradermal drug delivery by low-frequency sonophoresis (25 kHz)Dermatol Online J102415530314
- SchaferMKyaynakTLewinPDesign of a minature in vivo shock wave hydrophone. 10121990 Ultrasonics Symposium, 1990, Proceedings, IEEE 1990. 16236
- ShungKKSmithMBTsuiB1992Principles of medical imagingSan DiegoAcademic Press
- SmithNBLeeSMaioneE2003Ultrasound mediated transdermal transport of insulin through in vitro human skin using novel transducer designsUltrasound Med Biol29311712659919
- StansfieldD1990Underwater electroacoustic transducersBath, UKBath University Press
- SundaramJMelleinBRMitragotriS2003An experimental and theoretical analysis of ultrasound-induced permeabilization of cell membranesBiophys J84308710112719239
- TachibanaK1992Transdermal delivery of insulin to alloxan-diabetic rabbits by ultrasound exposurePharm Res995241438012
- TachibanaKTachibanaS1991Transdermal delivery of insulin by ultrasonic vibrationJ Pharm Pharmacol4327011676740
- TachibanaKTachibanaS2001The use of ultrasound for drug deliveryEchocardiography18323811415505
- TangHBlankschteinDLangerR2002Effects of low-frequency ultrasound on the transdermal permeation of mannitol: comparative studies with in vivo and in vitro skinJ Pharm Sci9117769412115805
- TangHMitragotriSBlankschteinD2001Theoretical description of transdermal transport of hydrophilic permeants: application to low-frequency sonophoresisJ Pharm Sci905456811288100
- TempanyCMStewartEAMcDannoldN2003MR imaging-guided focused ultrasound surgery of uterine leiomyomas: a feasibility studyRadiology22689790512616023
- ter HaarG1999Review: Therapeutic ultrasoundEur J Ultrasound93910099161
- TeraharaTMitragotriSKostJLangerR2002Dependence of low-frequency sonophoresis on ultrasound parameters. distance of the horn and intensityInt J Pharm235354211879737
- TezelAMitragotriS2003Interactions of inertial cavitation bubbles with stratum corneum lipid bilayers during low-frequency sonophoresisBiophys J8535021214645045
- VTezelAPaliwalSShenZ2005Low-frequency ultrasound as a transcutaneous immunization adjuvantVaccine233800715893617
- TezelASensAMitragotriS2003Description of transdermal transport of hydrophilic solutes during low-frequency sonophoresis based on a modified porous pathway modelJ Pharm Sci3819312532387
- TezelASensATuchschererJ2001Frequency dependence of sonophoresisPharm Res18169470011785688
- The Whitaker Foundation2003Biomedical Engineering and the Fight Against Diabetes, Annual ReportArlington, VAThe Whitaker Foundation
- TiwariSBPaiRMUdupaN2004Influence of ultrasound on the percutaneous absorption of ketorolac tromethamine in vitro across rat skinDrug Deliv11475115168791
- TresslerJFCaoWUchinoK1998Finite element analysis of the cymbal-type flextensional transducerIEEE Trans Ultrason, Ferroelect, Freq Contr4513639
- varez-RomanRMerinoGKaliaYN2003Skin permeability enhancement by low frequency sonophoresis: lipid extraction and transport pathwaysJ Pharm Sci9211384612761803
- WangGMYangYFSunLA2003An experimental study on high intensity focused ultrasound combined with mitomycin treatment of bladder tumorZhonghua Wai Ke Za Zhi4189790014728828
- WangYThakurRFanQ2005Transdermal iontophoresis: combination strategies to improve transdermal iontophoretic drug deliveryEur J Pharm Biopharm601799115939232
- WeimannLJWuJ2002Transdermal delivery of poly-l-lysine by sonomac-roporationUltrasound Med Biol2811738012401388
- WilsonOB1988An introduction to the theory and design of sonar transducersLos Altos, CAPeninsula Publishing
- WoodRWLoomisAL1927The physical and biological effects of high frequency sound waves with great intensityPhil Mag441736
- WuFWangZBChenWZ2004Extracorporeal high intensity focused ultrasound ablation in the treatment of 1038 patients with solid carcinomas in China: an overviewUltrason Sonochem111495415081972
- WuJChappelowJYangJ1998Defects generated in human stratum corneum specimens by ultrasoundUltrasound Med Biol24705109695274
- YamashitaNTachibanaKOgawaK1997Scanning electron microscopic evaluation of the skin surface after ultrasound exposureAnat Rec247455619096784
- YangJHKimDKYunMY2006Transdermal delivery system of triamcinolone acetonide from a gel using phonophoresisArch Pharm Res29412716756087
- ZhangIShungKKEdwardsDA1996Hydrogels with enhanced mass transfer for transdermal drug deliveryJ Pharm Sci Dec8513126