Abstract
Inclusions of lidocaine hydrochloride in cyclodextrins were prepared to obtain stable complexes compatible for association with chlorhexidine in a new gel formulation for use in urogenital applications. Two cyclodextrins, β-cyclodextrin and methyl-β-cyclodextrin, were used for encapsulating lidocaine hydrochloride through solubilization and kneading techniques. The lidocaine–cyclodextrin complexes were characterized by ultraviolet spectroscopy, Fourier transform infrared spectroscopy, differential scanning calorimetry, and X-ray diffraction. The results revealed that the techniques generated good yields of inclusion products that maintained the functional properties of lidocaine. In addition, the inclusion products obtained improved the compatibility of lidocaine hydrochloride with chlorhexidine in solution and a gel formulation. The gel formulation displayed desirable rheological and physicochemical properties. The results presented here are the first description of the inclusion of lidocaine with cyclodextrins, which improves compatibility with chlorhexidine in formulations for simultaneous delivery.
Introduction
The increase in various urogenital procedures, including invasive procedures, has created a need for sterile lubricants that also deliver, simultaneously, a local anesthetic and a bactericide.Citation1–Citation4 Yet the availability in the pharmaceutical market of products with these characteristics is still very low. The British National Formulary describes a gel formulation that includes 2% lidocaine hydrochloride (LID, anesthetic) and 0.25% chlorhexidine gluconate (CHX, bactericide) together, which is available commercially.Citation5 However, the characteristics of these drugs together show evidence of physicochemical incompatibilities, which suggests that this type of formulation is extremely unstable, unless one or both drugs have been modified to avoid such phenomena.Citation6,Citation7
The literature describes several approaches for improving the stability and/or compatibility for different drugs such as solvent selection during formulation and molecular encapsulation processes.Citation8 Cyclodextrins (CDs) are commonly used for encapsulation and comprise a series of α-1,4-linked cyclic oligosaccharides consisting of 6 (αCD), 7 (βCD), 8 (γCD), or more glucose units leading to a cup-like geometry. CDs have a rigid structure with a singular hydrophobic cavity due the absence of hydroxyl groups. Due to their distinctive structure, CDs are able to enhance the solubility, chemical stability, and bioavailability of poorly soluble drugs, while reducing toxicity and controlling the rate of release.Citation9 Moreover, complexing drugs with CD may also: i) enhance drug stability to air and light, ii) affect the availability of topically applied products, either increasing or decreasing their permeability into and through the skin, and iii) delay the beginning of the process of photodegradation.Citation9–Citation11 In comparison with other pharmacotechnical approaches, the performance of CDs often displays greater efficacy for stabilizing drugs and bridging drug incompatibilities in various formulations and, in many situations, represents the only alternative.
We investigated the inclusion of LID into CDs to create a new urogenital gel formulation together with CHX with increased stability and an adequate release profile. Towards that purpose, LID and the CDs β-cyclodextrin (βCD) and methyl-β-cyclodextrin (MβCD) were tested by using solubilization and kneading techniques. The LID–CD inclusion complexes were characterized and the gel formulated with these complexes was evaluated for the in vitro release of LID from the complex, its rheological properties, and other pharmacotechnical properties for characterization of a new formulation for delivery of an anesthetic together with a bactericide.
Material and methods
Materials
Ethanol (95%), butylated hydroxyanisole (BHA) and butylated hydroxytoluene (BHT), Nipagim, polyethylene glycol (PEG) 6000, PEG-15 cocopolyamine, and Natrosol were obtained from DEG (São Paulo, Brazil); the CHX standard (20% w/v) was obtained from Sigma-Aldrich (St Louis, MO); LID was obtained from Nortec Química (Xerém, RJ, Brazil); and βCD and MβCD were obtained from Wacker-Chemie GmbH (Munchen, Germany) and were of a pharmaceutical grade. The purified water used in all solutions preparations was prepared using a Milli-Q system (Millipore, Billerica, MA).
Drug and formulation analysis
The concentration of LID and CHX was determined following the protocols outlined by the Brazilian Pharmacopoeia (1998).Citation12 Other tests used to assess the quality of raw materials followed the guidelines of the Brazilian and British Pharmacopeia monographs.Citation12,Citation13
Apparatus and chromatographic conditions
A high performance liquid chromatograph (HPLC) model Shimadzu LC-10AT was used. The chromatographic conditions were initially based on a study of simultaneous determination of LID and CHX in a triamcinolone acetonide suspension, using reverse phase HPLC.Citation14 During the verification of the best chromatographic conditions to resolve the peaks of each drug using known standards, the mobile phase was varied between 60% and 65% of methanol with triethylamine (0.4%) at pH 3.5 and the detection wavelength was set at 240 nm. Experimental samples were analyzed based on those conditions.
Preparation of standard and sample solution
A working standard solution of LID and CHX in mobile phase (400 μg/mL for LID and 50 μg/mL for CHX) was prepared using drug reference standard from US Pharmacopeia that was accurately weighed and transferred into a 50-mL flask. Mobile phase was added to the mixture and sonicated until the standard dissolved completely (∼5 minutes). Then the flask was filled with mobile phase and filtered through a 0.45 μm membrane filter (Millipore).
For sample preparation, an adequate amount of gel formulation or chemical standard substances were weighed and the amount equivalent to 400 μg/mL of LID and 50 μg/mL for CHX were transferred to a 50 mL volumetric flask. After that, the preparation followed the same procedure described for the standard solution preparation.
Validation of HPLC quantitative determination of LID and CHX
In order to validate the analytical method proposed, the measurement parameters were investigated for linearity, accuracy, precision, and specificity according to the International Conference on Harmonization recommendations.Citation15
Linearity was tested with a range of concentrations (50%, 80%, 100%, 120%, and 150%); 100% corresponded to a concentration of 400 μg/mL for LID and 50 μg/mL for CHX. A calibration curve was generated and the linearity was evaluated by the calculated correlation coefficient and interception value.
Accuracy was evaluated by analyzing 3 independently prepared samples (spiked placebo) of 3 concentrations (80%, 100%, and 120% of the working concentration). Each preparation was measured 3 times. Values between 98% and 102% of recovery were considered acceptable.
Precision of the measurement method was determined by evaluating the repeatability and the intermediate precision of measurements on standards. Repeatability was evaluated using 6 replicated samples of a solution containing drugs at a concentration of 100% of the regular analytical working concentration.Citation15 The intermediate precision was judged by repeating the procedure on 2 different days. The results obtained were analyzed statistically and a RSD value below 2% was considered acceptable.
Specificity was determined with Class VP software, which calculated the peak purity by ratiogram. The specificity was further analyzed by comparing the chromatograms of the various formulations to the placebo chromatogram for evidence of interference from the formulation matrix on the measurements.
Formulation of LID and CHX gel formulations
Initially, LID and CHX were prepared in a range of ethanol solutions (10%, 30%, 50%, 70%, and 90%) to obtain final concentrations of 2.0% w/v for LID and 0.25% w/v for CHX. Complete solubilization of the drugs was observed. The compatibility of the 2 drugs was evaluated by maintaining solutions in a 10-mL beaker under magnetic stirring for 0, 5, 30, and 60 minutes, and 24 hours and observing turbidity. The presence of white precipitate was considered evidence of incompatibility.
The gel formulation preparation was initiated by choosing the ideal excipients based on compatibility with the drugs, especially for CHX. The tests for the carrier solutions were performed according to the procedures described above with a concentration of 0.25% w/v for CHX. After the compatible excipient was determined, the gel was formulated by adding methylparaben and BHA to a final concentration of approximately 40% w/v under magnetic stirring. Then, PEG-15 cocopolyamine was added to a concentration of 2%. After incorporation, hydroethylcellulose (4%) and the remaining water were added. This mixture was kept under magnetic stirring until complete gelation of the polymer and a final gel that was mostly clear with a slight yellow tint due to the presence of hydroxyethylcellulose and PEG-15 cocopolyamine. For a gel containing ethanol, BHA was substituted with BHT at the same concentration.
Preparation of LID–CDs complexes through the solubilization method
To establish the optimal ratio of LID with βCD and MβCD,Citation16–Citation18 stock solutions of the CDs (1.15 g diluted in 100 mL of purified water) and LID (50 mg dissolved in 5 mL solvent) were prepared separately. LID was then added to the vessels containing the CD solution (qs 20 mL) in volumes necessary to reach various CD:drug molar ratios (2:1, 3:1, 4:1, 5:1, 6:1, and 7:1). For control purposes, a “0” point including only the original LID solution without CD was also prepared. All mixtures were stirred for 72 hours at 25°C followed by a time without stirring to allow precipitates to settle, and then decanted through Whatman 42 analytical paper. If no additional precipitate was observed, the inclusion products were frozen at −70°C and lyophilized for further analysis.
Inclusion of LID into CDs by kneading method
The calculated amounts of drug and corresponding CD were weighed, and moistened with a small volume (1 mL) of ethanol:water solution (70:30, v/v). The mixtures were grounded using a ball mill until the mixture reached a granulation aspect.Citation16 The resulting product was further mixed for 30 minutes. The final samples were dried in an oven at 55°C for 30 minutes and stored in desiccators.
Characterization of the inclusion complexes
The concentrations of LID in the CD complex were determined by using an UV spectrometer (Perkin-Elmer, Norwalk, CT). The concentrations in the calibration curve were 2.0, 4.0, 6.0, 8.0, 10.0, and 12.0 μg/mL for LID, with R2 values between 0.995 and 0.998 in all of the studied cases. Fourier transformed infrared spectra for all samples were recorded using KBr pellets (1 mg sample per 300 mg KBr) on a Perkin-Elmer FT-IR Spectrometer Paragon 1000 grating instrument with slow scan and normal slit width: X-ray diffraction patterns of the drug–CD inclusion complex were obtained by using an X-ray Diffractometer (XRD; Rigaku, Tokyo, Japan). The diffraction angle (2θ) of the patterns was recorded from 2° to 10° at 1°/min, with a total scanning speed of 41 minutes. CuKa radiation was used as the X-ray source, which was operated at 40 kV and 30 mA at room temperature and 0.15418 nm wavelength. Differential scanning calorimeter analysis was performed from 0°C to 400°C (10°C/min) with a Perkin-Elmer DSC 7 in an aluminum pan under a nitrogen flow (22.5 mL/min).
Stability studies
The stability of the prepared gels was determined using each of the analytical methodologies previously described for the drug assays and also included pH and rheological proprieties. Samples were stored under 30 ± 2°C and 75% ± 5% RH for 30, 60, and 90 days. The pH of the samples was determined using a Digimed DM21 potentiometer. The rheological study was performed to obtain the physical parameters such as flow index, consistency index, apparent viscosity and thixotropy. Rheological measurements were obtained in an Advanced Rheometer (TA Instruments, New Castle, DE), model 2000, which is a cone and plate viscosimeter with a 60 mm cone and a 2° determination angle. Samples (3.0 g) were placed on the hot plate at 37°C and the cone rotates under adjustable speeds.
In vitro drug release analysis
The release of LID from the CD complexes and the gel formulation was evaluated in vitro using a modified apparatus based on the Franz Cell concept.Citation19,Citation20 An acetate cellulose membrane was mounted on a diffusion system and a sample containing 200 mg of LID was introduced into the donor compartment. Sink conditions were met in all cases and 6 parallel determinations were performed at 37 ± 0.5°C. Aliquots of the acceptor solution were withdrawn after 5, 20, 40, 60, 90, 120, 180, 240, 300, and 360 minutes. A volume of medium equal to that withdrawn was added back following each sampling. The collected fractions were filtered through a 0.45-mm membrane and the filtrate was analyzed by HPLC to quantify the amount of drug diffused into the acceptor solution using a standard curves with a range of 10% to 110% prepared in the same day.
The cumulative amount of drug released through the membrane was plotted as a function of time. The flux values (J) was calculated from the slopes of the linear portion (steady state) of in vitro release curves. Release kinetics were determined by linear regression analysis by applying 3 models: zero order, Higuchi, first order, and Peppas.
Statistical data analysis
One-way ANOVA and Wilcoxon match pair tests were used to analyze all of the data obtained.
Results and Discussion
Methods development and optimization
The isocratic-mode method with UV detection applied in this study was developed for the determination of the active ingredients, LID and CHX, at the 100% level, and its main degradation products, p-chloroaniline and 2,6-dimethylaniline. Towards that purpose, a reversed-phase column C18 (250 mm, 4.0 mm; 5.0 μm) was used and tested with mobile phases consisting of methanol concentrations of 60%, 62%, 63%, and 65% with 0.4% triethylamine solutions at various ratios (65:35; 63:37; 62:38, and 60:40, v/v). The variation in the methanol concentration of the mobile phase significantly modified the CHX retention time, but not the LID retention time, which remained mostly unchanged. An increase in the methanol concentration from 60% to 65% in the mobile phase decreased the CHX retention time from 14.5 to 6.4 minutes. The addition of triethylamine to the mobile phase generated an improved symmetry of the observed chromatographic peaks for both drugs without an alteration in the retention time or the separation of the peaks. Variations in the pH of the mobile phase from 3.0 to 4.0 did not significantly alter the drug retention time, but affected the shape of the peak for CHX. By comparing the chromatographic behavior as peak retention times, theoretical plates, capacity, and tailing factors, the chromatographic conditions described in were considered ideal. Under the preferred conditions, LID displayed a retention time of 4.4 minutes, a theoretical plate of 3961, and an asymmetry of 1.66. For CHX, the retention time was 10.1 minute with a theoretical plate of 6707 and an asymmetry of 1.47. The literature described similar results from the analysis of another gel formulation, but with different excipients.Citation14
Table 1 Chromatographic analysis conditions for chlorhexidine gluconate (CHX) and lidocaine hydrochloride (LID)
Method validation
The selectivity of the assay method for the drug determination and for the presence of CHX degradation products (p-chloroaniline and 2,6-dimethylaniline) in the formulation was accessed by individual chromatograms obtained for each drug with the formulation matrix and revealed an adequate peak purity for the drugs ().
Figure 1 Chromatograms of placebo contaminated with LID, CHX, and degradation products (p-chloroaniline and 2,6-dimethylaniline) A) and the respective 3D UV/Vis spectra B).
Abbreviations: CHX, chlorhexidine gluconate; LID, lidocaine hydrochloride.

The linearity was determined using 5 samples in different concentrations between 50% and 150% from the nominal assay concentration of 400 μg/mL for LID and 50 μg/mL for CHX standard. This procedure was carried out on 2 different days in agreement to the ICH.Citation15 A calibration curve was constructed to calculate the correlation coefficient and intercept value, which was evaluated using a statistic study (ANOVA) and determined to be significant (P < 0.05). The regression equation was obtained by a least squares treatment of the data and the results confirmed the linearity of the data from the method developed (). The homoscedasticity of the calibration curves was tested using Cochran’s test (G = largest variance/sum of variances).Citation21 The G value obtained in this study (0.419) was smaller than the critical value (G = 0.684), indicating that variances were not significantly different.
Table 2 Summary data of linearity for HPLC method
The accuracy was evaluated by the recovery of CHX and LID at 3 different levels (80%, 100%, and 120%), tested 3 times. The individual recoveries ranged from 99.6% to 100.4% for LID and 98.1% to 100.7% for CHX with an RSD < 1.5% in both cases. Therefore, these results indicate the accuracy of the method for determination of both drugs.
The reproducibility of the methods was confirmed by analyzing 3 sample replicates on the same equipment with a concentration that ranged from 80% to 120% of the analytical concentration (100%). The intermediate precision was determined by performing the analysis on 2 different days. The data presented in show that the method has a good repeatability and intermediate precision.
Table 3 Summary data of repeatability and intermediate precision
The detection limit was 2.7 μg/mL for LID and 0.6 μg/mL for CHX, while the quantification limit was 9.0 μg/mL for LID and 2.1 μg/mL for CHX. These values were calculated using calibration curves generated from a range of concentrations between 50% to 150% of each drug (200 to 600 μg/mL for LID and 25 to 75 μg/mL for CHX).
CHX and LID gel formulation development
Initially, the compatibility of CHX and LID together in solution was investigated. CHX and LID are known to be incompatible in the presence of different ions and hydrochlorides leading to precipitation and degradation, but unknown in a simple hydroalcoholic solution. The result of degradation is the formation of various amounts of p-chloroaniline, which was described previously.Citation6,Citation22,Citation23 Ethanol was chosen as the solvent because of its utilization in various gels formulas. The increase of ethanol in the mixture up to 75% did not prevent the precipitation. An immediate precipitation was observed when using 0.5% ethanol and, with a delay up to 24 hours, when using 95% ethanol. Therefore, even in a less polar solvent, which effectively dissolved less LID, incompatibility between the drugs was observed. This observation contradicts the report by Abdelmageed and ColleaguesCitation24 which described a stable relationship within a formulation (trade name Instilliagel®) with morphine sulfate, which was prepared for clinical studies.Citation24 Our results demonstrating an incompatibility between LID and CHX in solvents normally capable of dissolving the drugs individually suggested the need for an alternative approach to prevent the formation of precipitate and a break-down of the drugs.
The alternative approach was to complex one of the drugs into CDs. LID was chosen since it is less soluble and smaller then CHX, increasing the chance of a total inclusion into the CD cavity. The inclusion of CHX and LID into CD has been previously described, but only for the free base and not for the salt forms of these drugs.Citation25,Citation26 To complex the salt form of LID, βCD and MβCD were investigated for their ability to form LID inclusion complexes. The stability constant of the inclusion complex was determined by solubilization method with different concentration of CDs, emphasizing that LID solution absorbance is near to zero, differentiating the inclusion complex obtained. The correlation between the observed absorbance depended on the concentration of βCD added to the LID that was previously solubilized in the medium and was linear with a correlation coefficient of 0.9996. The calculated stability constant (K) of the complexed LID with βCD was 34954.1±995.4 M−1. For the solution using MβCD, the correlation absorbance concentration was also linear with a coefficient of 0.9955. The calculated stability constant of the complex LID–MβCD was 5565.3 ± 819.9 M−1. These values are considered high compared with those previously reported, indicating a stabile complex of LID with both ßCD and MßCD.Citation27 Despite the variations observed, the water solubility observed for both complexes indicates their feasibility in formulating theses complexes as gels at a concentration of 2.0% w/w, which translates to values of 24.95 mg/mL for LID–βCD and 131.56 mg/mL for LID–MβCD at room temperature. The solubility of the inclusion complexes in aqueous medium was lower than LID alone (166.4 mg/mL), which can be seen with the inclusion of very water soluble molecules.Citation26,Citation28–Citation30 In ethanol, the extent of solubility observed ruled out the possibility of obtaining gel formulations of 2.0% w/w for the molecules under study.
The kneading process was also attempted for the inclusion of LID with CDs due to the industrial feasibility of this technique. From the experimental determination of the stability constants, LID was included into βCD and MβCD at a 1:1 CD:drug ratio. The kneading method is described in the literature as an easy procedure, with low cost and high reproducibility.Citation11 Using this technique, the results showed a successful inclusion process with about 100% yield for βCD and MβCD as measured by LID extraction and HPLC analysis.
The XRD patterns of LID demonstrated that the physical mixtures and inclusion complexes in βCD and MβCD had a progressive loss of crystallinity in the complex, which demonstrated the phenomenon of inclusion (). The infrared analysis of the inclusion products showed LID present in the sample. However the infrared spectra of inclusion complex and physical mixture were quite similar. The major difference was the amine bands of LID (1650 and 1550 cm−1) present in the spectrum of physical mixture that disappear in the spectrum of the inclusion complex. The analysis of the inclusion products using DSC revealed the formation of the inclusion complex by the disappearance of the fusion endotherm of LID (127–129°C) with the concomitant maintenance of the dehydration endotherms at around 158°C and 300°C, typical of MβCD (). As reported in the literature, the ethanol competition with the guest molecule could also interfere in the formation of the inclusion complex, which strengthens the preferential use of water as solvent.Citation31
Figure 2 Diffractograms of LID A), physical mixture 1:1 B), inclusion complex of LID–MβCD C), and MβCD D).
Abbreviations: MβCD, methyl-β-cyclodextrin; CHX, chlorhexidine gluconate; LID, lidocaine hydrochloride.
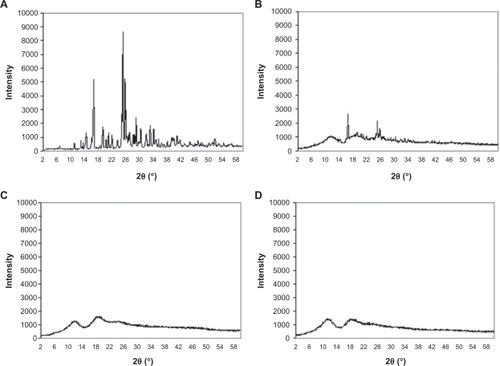
Figure 3 DSC thermogram of MβCD (in pink) and the inclusion complex LID-MβCD 1:1 (in blue).
Abbreviations: LID, lidocaine hydrochloride; MβCD, methyl-β-cyclodextrin.
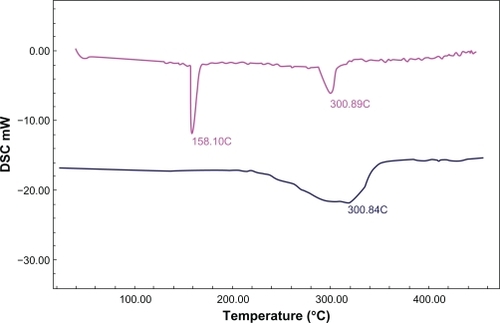
The compatibility of the LID inclusion complexes with CHX was evaluated by the preparation of an aqueous solution of LID at 2.0% w/w or its equivalent within inclusion complexes with βCD or MβCD mixed with CHX at 0.25% w/w. Both complexes of LID showed an absence of turbidity over a 24-hour period. The noncomplexed control, consisting of an aqueous solution of LID in the presence of CHX, displayed turbidity and precipitation within 3 minutes. Over a 10-day period with agitation at room temperature, extensive precipitation was observed in control solutions. Solutions containing LID complexed with CDs maintained equivalent levels of CHX in filtrates after filtration, as seen in . These data clearly demonstrate that the inclusion of LID with CD effectively stabilized the presence of CHX in solution over time and permitted both drugs to be present together.
Table 4 Results of the compatibility study of free drugs in aqueous media comparing inclusion complexes during 10 days
The higher solubility in water observed for the LID complexed with MβCD was the deciding factor to utilize these complexes to formulate a new gel. Three ingredients of each functional category needed for gel formation were tested for their compatibility with the drugs in aqueous solution and their compatibility percentages defined. These complexes were soluble or miscible with the additives, with no turbidity or precipitation observed in the solutions after 10 days under agitation. presents the basic composition proposed for the formulation of the gel.
Table 5 Formula proposed for the new LID and CHX gels and description of the functional classification of each excipient
The final gel presented a slightly yellowish color and an LID content of 98.8% ± 0.04% and a CHX content of 99.6% ± 0.002%. The pH measured was 5.8, close to the optimum pH for a vaginal cream (3.5–4.5). The pH of greater stability for the drugs is between 3 and 6.Citation6,Citation32 An excellent stability was observed for the new formulation (), indicating its strong commercial viability.
Table 6 Assay of drugs in the proposed formulation at time zero and after 6 months
In release studies, purified water was used as the acceptor solution.Citation33–Citation35 The CHX solubility determined in this medium was 200 mg/mL, and for LID 166.4 mg/mL. In order to ensure sink conditions, the volume of acceptor solution was fixed at 20.0 mL. The sink condition was considered satisfactory since the drug concentrations in this medium was at least 10 times smaller than the experimental drug solubility, considering that all drugs in the donor compartment were released. and show the in vitro release profiles over 6 hours using the formulations proposed. The results demonstrated that the drugs were released from the formulation. The release kinetics followed the Higuchi model (r > 0.99). The flux (J) was calculated from the slopes in the linear portion of the curves and was 6.196 ± 0.49 μg/cm2 · min−1 for LID and 1.0 ± 0.06 μg/cm2 · min−1 for CHX.Citation36
Table 7 Cumulative amounts of LID and CHX released from the formulation proposed in function of time in vitro release studies
Figure 4 Amount of lidocaine hydrochloride A) and chlorhexidine gluconate B) released (μg/cm2) versus time (hours) from the proposed formulation (n = 12).

Appropriate rheological properties were observed for the gel proposed, especially in terms of its ability to spread and to coat the urogenital mucosa.Citation37 The rheograms presented in and show that the formulation behaved as a non-Newtonian pseudoplastic fluid. The profiles of the rheograms showed an increase in shear stress with an increasing shear rate (0.1 to 100 s−1) and a reduction of tension by reducing this rate (100 to 0.1 s−1). Both present a nonlinear curve profile with a tendency to decrease the apparent viscosity of the formulation as a function of increasing shear rate. The classification of the formulation as a non-Newtonian fluid determines that the interactions between its components affect the rate of deformation caused by external forces, resulting in no proportionality between the rate (γ) and shear stress (τ), as shown in .Citation38
Figure 5 Rheogram of the proposed formulation showing shear stress versus shear rate rising from 0.1 to 100 s−1 (diamond) and decreasing from 100 to 0.1 s−1 (square).

Figure 6 Rheogram of the proposed formulation showing apparent viscosity versus shear rate (0.1 to 100 s−1).
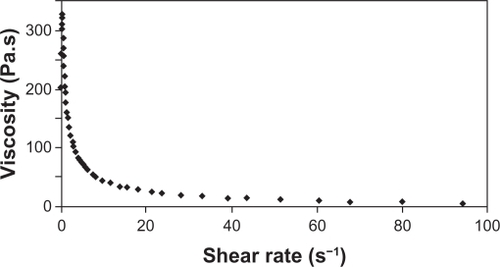
The behavior exhibited by the new formulation corresponds to that obtained by Owen et al testing some commercial vaginal contraceptive gels.Citation39 These gels, when subjected to rheological studies, were characterized as a non-Newtonian pseudoplastic fluid due to reduced viscosity of the formulation prepared against the increase of shear rate. By observing the flow index (n) of the formulation, which indicates the degree of resistance during the fluid flow, we measured a value of 0.4084, which corresponds to a pseudoplastic formulation. The consistency index obtained was 151.4 Pa · sn. These values of flow index and consistency index are consistent with those of the commercial contraceptive gels previously reported.Citation39
The rheograms shown in demonstrate the dependence behavior of elastic modulus (G′) and viscous modulus (G″) in relation to frequency, ranging from 0.1 to 10.0 Hz with strain rate set at 5%, characterized as a pseudogel.
Figure 7 Rheogram of the proposed formulation showing the elastic modulus G′ (diamond) and viscosity modulus G″ (square) versus frequency from 0.1 to 10.0 Hz with a fixed percentage of deformation.
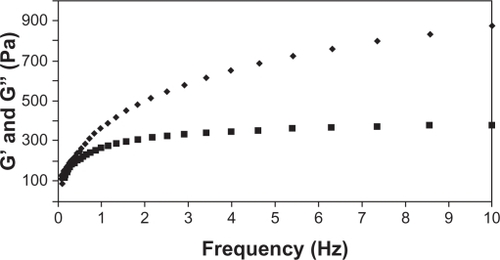
describes the behavior of the apparent viscosity of the formulation over time when the shear rate was fixed at 10.0 s−1. The significant decrease in viscosity during the initial 30 seconds, with a relative standard deviation of 4.52%, and the profile of this constant from the 30-second test with a standard deviation of 1.18%, provided further evidence to characterize the formulation as nonthixotropic.
Conclusion
The analytical methodology presented here was validated for linearity, selectivity, precision, sensitivity, and accuracy to assure its suitablity for use in quantifying the concentrations of LID and CHX in the various formulations and their release from complexes in vitro. We conclude that LID and CHX are incompatible when present together in aqueous media. We circumvented this incompatibility by the inclusion of LID in MβCD, which enabled the formulation of a gel that presents both an analgesic and bactericide simultaneously. The inclusion of 2 mucoadhesive agents, hydroxyethylcellulose and PEG-15 cocopolyamine, creates a novel gel suitable for application to the physiological conditions of the urogenital cavities. The results presented here represent the first description of the inclusion of a salt of lidocaine into CDs, which points to the potential use of this technology in various other pharmacotechnical applications.
Acknowledgements
This work was supported by FAPERJ, CAPES Edital CAPES Nanobiotecnologia 2008 and CNPq. We are grateful to William Provance Jr for English review.
Disclosure
The authors report no conflict of interest in this work.
References
- DohertyDWInstillagel: an anaesthetic antiseptic gel for use in catherizationBr J Nurs19998210911210214141
- MageraJSJrInmanBAElliottDSDoes preoperative topical antimicrobial scrub reduce positive surgical site culture rates in men undergoing artificial urinary sphincter placement?J Urol20071784 Pt 11328133217698144
- CollinsMKPorterKBBrookEJohnsonLWilliamsMJevittCMVulvar application of lidocaine for pain relief in spontaneous vaginal deliveryObstet Gynecol19948433353378058226
- SperlingHBastianHPBoeminghauFLudwigGTerhorstBRübbenHLocal antiseptic therapy for non-gonococcal urethritis: phase II study with InstillagelWorld J Urol 200;201454712088190
- British National Formulary60th edLondon, UKBritish Medical Association and Royal Pharmaceutical Society of Great Britain2010
- SweetmanSCMartindale: The Complete Drug Reference32rd edLondon, UKThe Pharmaceutical Press1999
- MoffatACOssletonMDWidropB3rd edClarke’s Analysis of Drugs and PoisonsLondonPharmaceutical Press2004
- LoweNJShaathNAPathakMA2nd edSunscreens: Developments, Evaluations and Regulatory AspectsNew YorkMarcel Dekker, Inc1997
- UekamaKHirayamaFIrieTCyclodextrin drug carrier systemsChem Rev199829392045207611848959
- RajewskiRAStellaVJPharmaceutical applications of cyclodextrins. In vivo drug deliveryJ Pharm Sci19968511114211698923319
- LoftssonTBrewsterMEPharmaceutical applications of cyclodextrin I. Drug solubilization and stabilizationJ Pharm Sci19968510101710258897265
- Brazilian Pharmacopoeia4th edSão PauloAtheneu1998
- British PharmacopoeiaLondonHer Majesty’s Stationery Office2010
- XuYWongGYSimultaneous determination of lignocaine hydrochloride, chlorhexidine gluconate, and triamcinolone acetonide in suspension by reversed-phase HPLCJ Liq Chromatogr Relat Technol1999221320712091
- International Conference on Harmonization (ICH)Q2B Validation of Analytical Procedures: Methodology US FDA Federal Register,1997 http://www.fda.gov/downloads/Drugs/GuidanceComplianceRegulatoryInformation/Guidances/UCM073384.pdf
- ScaliaSVillaniSScatturinAVandelliMAForniFComplexation of the sunscreen agent, butyl-Methoxidibenzoilmethane with hydroxypropyl β−cyclodextrinInt J Pharm1998175205213
- ScaliaSMolinariACasolariAMaldottiAComplexation of the sun-screen agent, phenylbenzimidazole sulphonic acid with cyclodextrins: effect on stability and photo-induced free radical formationEur J Pharm Sci200422424124915196580
- HiguchiTConnorsKAPhase-solubility techniquesReillyCAdvances in Analytical Chemistry and InstrumentationNew YorkWiley/Interscience1965117212
- KarpanenTJWorthingtonTConwayBRHiltonACElliottTSJLambertPAPenetration of chlorhexidine into human skinAntimicrob Agents Chemother200852103633363618676882
- MoreiraTSSousaVPPierreMBRA novel transdermal delivery system for the anti-inflammatory lumiracoxib: influence of oleic acid on in vitro percutaneous absorption and in vivo potential cutaneous irritationAAPS Pharm Sci Tech2010112621629
- Murillo PulgarínJAAlañón MolinaAAlañónMTDirect determination of naftopidil by non-protected fluid room temperature phosphorescenceAnalyst2001126223423811235109
- BasraniBRManekSSodhiRNSFilleryEManzurAInteraction between sodium hypochlorite and chlorhexidine gluconateBasic Res Tech2007338966969
- RasimickBJNekichMHladekMMMusikantBLDeutschASInteraction between chlorhexidine digluconate and EDTAJ Endod2008341215121513
- AbdelmageedRLabyadNWatsonDGPournamdariMCableCGStanleyEEvaluation of the stability of morphine sulphate in combination with InstillagelJ Clin Pharm Ther200833326327118452413
- FrancoCFPataroALSouzaLCRSantosVRCortésMESinisterraRDIn vitro effects of a chlorhexidine controlled delivery systemArtif Organs200327548649112752214
- TataiAAignerZErösIKataMPreparation and investigation of mixtures containing lidocaine base and β-cyclodextrinJ Incl Phenom Macrocycl Chem2007591–2105113
- MukneAPNagarsenkerMSTriamterene-β-cyclodextrin systems: preparation, characterization and in vivo evaluationAAPS Pharm Sci Tech200451 Article 19.
- ChallaRAhujaAAliJKharRKCyclodextrins in drug delivery: an updated reviewAAPS Pharm Sci Tech200562E329E357
- BrewsterMELoftssonTCyclodextrins as pharmaceuticals solubilizersAdv Drug Deliv Rev200759764566617601630
- CalKCentkowskaKUse of cyclodextrins in topical formulations: pratical aspectsEur J Pharm Biopharm200868346747817826046
- WadeAWellerPJHandbook of Pharmaceutical Excipients3rd edLondonPharmaceutical Press1999
- PowellMFStability of lidocaine in aqueous solution: effect of temperature, pH, buffer, and metal ions on amide hydrolysisPharm Res19874142453508528
- RicciEJLunardiLONanclaresDMAMarchettiJMSustained release of lidocaine from poloxamer 407 gelsInt J Pharm2005288223524415620863
- SenelSIkinciGKasSYousefi-RadASargonMFHincalAAChitosan films and hydrogels of chlorhexidine gluconate for oral mucosal deliveryInt J Pharm2000193219720310606782
- HuangJWongHLZhouYWuXYGradHKomorowiskiRIn vitro studies and modeling of a controlled-release device for root canal therapyJ Control Release2000672–329330710825562
- CostaPLoboJMSModeling and comparison of dissolution profilesEur J Pharm Sci200113212313311297896
- ChangJYOhYKChoiHGKimYBKimCKRheological evaluation of thermosensitive and mucoadhesive vaginal gels in physiological conditionsInt J Pharm2002241115516312086731
- GallegosCFrancoJMRheology of food, cosmetics and pharmaceuticalsCur Op Inter Sci199944288293
- OwenDHPetersJJKatzDFRheological properties of contraceptive gelsContraception200062632132611239620