Abstract
Introduction
Stem cell therapies for neurodegenerative diseases such as Parkinson’s disease (PD) are intended to replace lost dopaminergic neurons. The basis of this treatment is to guide the migration of transplanted cells into the target tissue or injury site. The aim of this study is an evaluation of the homing of superparamagnetic iron oxide nanoparticles (SPIONs) labeled adipose-derived stem cells (ADSC) by an external magnetic field in a rat model of PD.
Methods
ADSCs were obtained from perinephric regions of male adult rats and cultured in a DMEM medium. ADSC markers were assessed by immunostaining with CD90, CD105, CD49d, and CD45. The SPION was coated using poly-L-lysine hydrobromide and transfection was determined in rat ADSC using the GFP reporter gene. For this in vivo study, rats with PD were divided into five groups: a positive control group, a control group with PD (lesion with 6-HD injection), and three treatment groups: the PD/ADSC group (PD transplant with ADSCs transfected by BrdU), PD/ADSC/SPION group (PD transplant with ADSCs labeled with SPION and transfected by GFP), and the PD/ADSC/SPION/EM group (PD transplant with ADSCs labeled with SPION and transfected by GFP induced with external magnet).
Results
ADSCs were immunoreactive to fat markers CD90 (90.73±1.7), CD105 (87.4±2.9) and CD49d (79.6±2.6), with negative immunostaining at the hematopoietic stem cell marker (CD45: 1.4±0.4). The efficiency of cells with SPION/PLL was about 96% of ADSC. The highest number of GFP-positive cells was in the ADSC/SPION/EM group (54.5±1.3), which was significantly different from that in ADSC/SPION group (30.83±3 and P<0.01).
Conclusion
Transfection of ADSC by SPION/PLL is an appropriate protocol for cell therapy. External magnets can be used for the delivery and homing of transplanted stem cells in the target tissue.
Introduction
Parkinson’s disease (PD) is the second most common neurodegenerative disease of the central nervous system (CNS) and is characterized by a progressive loss of neurons.Citation1,Citation2 The loss and death of dopaminergic neurons at the substantia nigra pars compacta (SNc) leads to symptoms such as resting tremor, bradykinesia, rigidity, and cognitive dysfunction.Citation1,Citation3 This decrease is associated with dopamine deficiency and is treated by two strategies.Citation2,Citation4 The first treatment is generally through dopamine replacement therapy (L-DOPA)Citation5 although these drugs cannot prevent the loss of dopaminergic neurons and provides a temporary decrease of symptoms, but long-term administration can lead to serious side effects, such as dyskinesia.Citation5,Citation6 The second treatment strategy consists of replacing damaged neurons via transplantation of dopamine neurons.Citation7,Citation8 Several types of stem cell, including embryonic mesencephalic progenitors,Citation9 neural stem cells (NSCs),Citation10 embryonic stem cells (ESC),Citation11 mesenchymal stem cells (MSCs),Citation12 pluripotent stem cells (iPSCs),Citation13 and hematopoietic stem cells have been transplanted into the brain with the goal of promoting the improvement of PD.Citation14
Among these cellular sources, adipose-derived stem cells (ADSC) are an accessible and autologous source for neural lineage in cell therapy.Citation15–Citation17 ADSCs have multipotency, are capable of self-renewing, and can differentiate into types of mesenchymal lineage and transdifferentiate into neurons and glial-like cells.Citation18,Citation19 Research has shown transdifferentiated ADSCs into neuron-like cells with selectively expressed nestin and neuronal molecules.Citation18–Citation20 Similar results have been reported in functional transdifferentiated ADSCs into motor neuron-like cells (MNLC). MNLC showed immunostaining of their processes with ChAT and synaptophysin and their potential for load and unload FM1-43 fluorochrome and synapsed with myotube cells.Citation21 Other research has confirmed the potential of ADSCs for differentiation to a Schwann cell phenotype.Citation22 Therefore, neuron-like cells derived from adult stem cells, such as ADSC, can be used to replace damaged cells in patients suffering from Alzheimer’s disease, amyotrophic lateral sclerosis (ALS), Huntington’s disease and Parkinson’s disease.Citation23–Citation25
Nanotechnologies and nanostructures are widely used in human medical applications, including imaging and delivery of gene or therapeutic drugs to tissues.Citation26,Citation27 The studies have shown that even the cell organelle distribution of drugs can be improved by drug-loaded nanoparticles. These nanoparticles enter tissues and are taken up by cell structure.Citation28–Citation30 One nanoparticle is the Super Paramagnetic Iron Oxide Nanoparticle (SPION), which has been used as a nanomaterial for radiological diagnostic procedures in vivo.Citation31,Citation32 In particular, a new role of these different forms of SPIONs may be in cell technology and expression of genes.Citation33
To reduce the accumulation of SPION in tissues, biopolymers are used to promote biocompatibility. Recent studies have shown that SPION used with poly-L-lysine hydrobromide (PLL) can aid in the transfecting of stem cells.Citation33,Citation34 Moreover, the results of the previous studies showed an increased migration and integration capacity of SPION labeled MSCs to the damaged region with an external magnetic field.Citation35,Citation36 The efficiency of cell migration in myocardial infarction rat modelsCitation37 and olfactory-injured mouse modelsCitation38 increased about 6.4-fold and 3-fold, respectively, with an external magnet close to the injury site. Since migrating and transferring transplanted cells into the injured area is one of the most important issues reported in the process of cell therapy, the purposeful use of new methods for transferring and maintaining cells in the injured areas is of high clinical importance. The migration of transplanted cells into the injured area is raised as the homing phenomenon. In this study, it has been tried to establish cellular focus in the injured area by using magnetic absorption technique of iron nanoparticles and to study the viability of the transplanted cells in relation to the behavioral process.
The purpose of this study is the use of ADSCs for cell therapy in a rat model of PD to evaluate the neuroprotection and homing of superparamagnetic iron oxide nanoparticles (SPIONs) labeled ADSCs by magnetic attraction in PD.
Materials and Methods
Rat ADSC Isolation
ADSC was harvested from male Sprague-Dawley rats (200–250 g) obtained from the animal house at the Razi Institute. The isolation of ADSC was done according to Darvishi et al. Briefly, perinephric and hypogastric fat was separated from rats and washed and then incubated and digested in 0.075% collagenase type I (Sigma Company) and DMEM with agitation (30 min at 37°C). This suspension was neutralized though 10% FBS into DMEM (GIBCOBRL, Eggenstein, Germany) and then filtered through a 100μm nylon filter (Falcon Company). After centrifuging for 10 mins at 1000 rpm, the plate of cells was cultured in a T25 flask containing DMEM, 10% FBS, 100 U/mL penicillin and 100μg streptomycin for 7 days at 37°C. After the fourth passage, the ADSCs were evaluated using primary antibodies against CD90, CD105, CD49d, and CD45. The multipotency of the ADSCs was examined using osteogenic, adipogenic and chondrogenic differentiation.Citation21 In addition, 500,000 cells were transferred to 6 well plates to study the differentiation ability of ADSC into neuron-like cells and cultured with 2 mL DMEM/F12 medium containing 2% B27, 20 ng/mL bFGF and 20 ng/mL EGF. After 7 days, neurospheres were formed and then cultured with 10% FBS and transferred to 12 well plates for confirmation of nerve markers. In order to perform immunocytochemical techniques, mouse anti-NF-68 monoclonal antibody, mouse anti-Nestin monoclonal antibody and mouse anti-sox2 monoclonal antibody, mouse anti-Neun monoclonal antibody were used as primary antibody and mouse anti- FITC-conjugated was used as secondary antibody all related to Millipore. At this stage, PI was used for staining the nucleus to differentiate the nucleus.
Preparation of SPION
SPION was synthesized according to Albukhaty et al. Briefly, 1.0 mL of 2M FeCl2 and 4.0 mL 1M FeCl3 were mixed in a 100 mL beaker under force shaking, and then 50mL of 1M NH3 was added slowly in solution by the co-precipitation method. The precipitate was accomplished with tetramethyl ammonium hydroxide 25% with vigorous stirring for 3 mins to suspend the solids in the solution. The powder was weighed and dissolved with distilled deionized water using ultrasound for 5 mins.Citation39
ADSC Labeled by SPION/PLL and Transfection with GFP
For the production of SPION/PLL complex, 1.5 µg /mL of PLL was mixed with 50 µg/mL of SPION and DMEM culture media on a rotating shaker at 600 rpm at 37 ºC for 1h. These cultured media were added to ADSCs in concentration with SPION and PLL at 25µg/mL and 0.75 µg /mL, respectively. After 24h, the complex culture media were removed and replaced by DMEM containing 10% FBS. Also, to track the transfection of GFP, ADSCs labeled SPION/PLL and ADSCs at passage 4 were transferred to 6-well plates and GFP was added and placed on a super magnetic plate for 35 mins. GFP expression was examined using fluorescent microscopy after 48 h.Citation39
Surgical Procedures
Thirty-five adult male rats were divided into five groups (N=7). Group one: positive control (operation without 6-HD injection); group two: PD group (lesion in the medial forebrain bundle (MFB) with 6-HD injection); group three: PD/ADSC group (PD transplant with ADSCs transfected by GFP); group four: PD/ADSC/SPION (PD transplant with ADSCs labeled with SPION and transfected by GFP); group five: PD/ADSC/SPION/EM (PD transplant with ADSCs labeled with SPION and transfected by GFP induced with external magnet). The lesion in the medial forebrain bundle (MFB) was performed by injecting 6-HD solution (4µg 6-HD per µL in 0.01% ascorbic acid, pH = 5 (Sigma)) under anesthesia [ketamine and xylazine (80 mg/kg and 10 mg/kg, respectively)] and aseptic conditions. The 6-HD was injected unilaterally into the right MFB at the following coordinates: AP: −4.0 mm; ML: ±1.5 mm; DV: −8.5 mm; tooth bar: ±0 mm relative to bregma by a Hamilton syringe connect to microinjection.Citation40
Cell Transplantation
Two weeks after stereotaxic surgery and toxin injection, the animals were re-anesthetized as mentioned above, and the skull was re-exposed. The GFP-transfected ADSCs were collected using Accutase (Gibco) from cell culture plates. The transplantation of labeled cells was carried out using a 30-gauge needle and a microinjection pump (Stoelting Co.) at a rate of 25 µL/min. The transplants consisted of 300,000 cells/9µL normal saline at the regions of the treatment site (AP: −4.0 mm; ML: ±1.5 mm; DV: −8.5 mm). The animals in the PD/ADSC/SPION/EM group wore an external magnet (0.32 T) on their skull for 1 week. The histological evaluation took place 6 weeks after the transplantation of cells.
Apomorphine-Induced Rotation
This test was based on the method used by Moayeri et al in 2019.Citation41 In summary, 6-HD injection causes extensive neuronal destruction in the SNc. Two to 4 weeks after surgery, the mice showed opposite rotations in the opposite direction to apomorphine injection. The number of these rotations per time unit is a measure of neuronal destruction severity in the SNc and the effect of the intervention. To perform this test, the mice were first housed in a transparent plexiglass cylinder (28 cm diameter and 38 cm height) and given 5 mins to adapt to the environment. Then, apomorphine hydrochloride 0.5 mg/kg dissolved in saline was injected to the mice and 1 min later the number of rotations to the venom injection site (negative number) or against (positive number) was recorded for 1 hr. Finally, the number of pure rotations of the mice to one side was calculated by the algebraic sum of the numbers obtained.Citation41
Prussian Blue Staining
ADSCs labeled with SPION/PLL complex were seeded on a gelatin-coated coverslip, washed in PBS and fixed in 4% paraformaldehyde in phosphate buffer solution for 15 min. The fixed cells were exposed three times with Prussian blue, then incubated with 5% potassium ferrocyanide (Merck, Germany) for 30 mins. Next, they were incubated in 5% HCL, washed with PBS, and counterstained with safranin stain (Merck, Germany). The evaluation of the intracellular iron oxide was done by a light microscope.Citation39
Statistical Analysis
The ANOVA with Tukey’s multiple comparison was used in reporting the results using SPSS 14. The significance was evaluated for P<0.05.
Ethics Statement
This study was carried out in accordance with the recommendations of International Council for Laboratory Animal Science (ICLAS) and was approved by the Ethical Committee of the Shefa Neuroscience Research Center.
Results
Rat ADSC Characterization
shows ADSC immunoreactivity to the following markers: mesenchymal stem cell (CD90: 90.73±1.7), fat-derived mesenchymal stem cell (CD105: 87.4±2.9) and fat cell-specific (CD49d: 79.6±2.6, while shows negative immunostaining at the hematopoietic stem cell marker (CD45: 1.4±0.4). The expression of CD90, which was highest, is consistent with other markers (P≤0.05, )
Moreover, separated ADSCs in the fourth passage are homogenous compared with the primary culture. show the osteogenic, chondrogenic and adipogenic differentiation of the ADSCs, respectively. The NS is shown in , whereas the characterization of the NSC derived from the NS (NSC marker) is showed in . They were immunoreactive to nestin, NF-160, Sox2 (differentiating neuronal markers) (, respectively). As well as they were negatively immunostained with Neun as differentiated neuron markers ().
Figure 1 Immunolabeling of adipose-derived stem cells (ADSC) with primary antibodies to CD90 (A), CD105 (B), CD49d (C), and CD45 (D), respectively; incubated with a secondary antibody conjugated with FITC (green) and nucleus counterstained with propidium iodide (red).
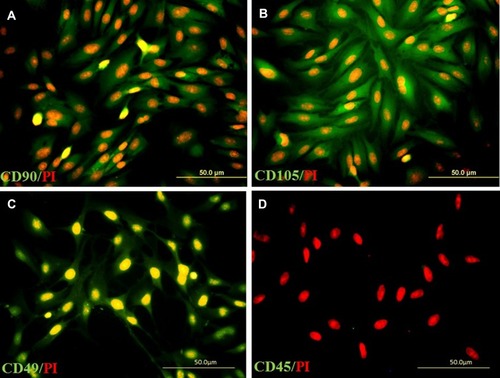
Figure 2 Alizarin red staining demonstrates osteogenic differentiation of ADSC (A). Safranin O staining presents chondrogenic differentiation of the extracellular matrix (B) and Oil red staining indicates Lipogenic differentiation of ADSC (C). White and black arrows indicate the extracellular matrix of osteocyte- and chondrocyte-derived ADSC, respectively, along with a histogram of the percentages of immunoreactive cells to CD105, CD90, CD49d, and CD45. (D) aStatistically different from CD105, CD90 and CD49d.
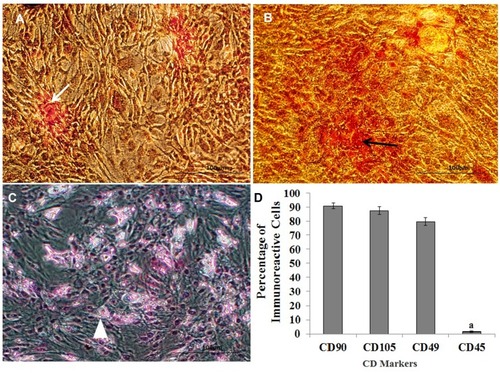
Figure 3 Cultured the Neural Stem Cell (NSC) derived from the Neurospheres (NS), immunostaining, and the percentage of immunoreactive cells to each neural marker. (A) Morphology of the NS (7 days), (B–E): Immunolabeling with the primary antibodies to nestin, NF-160, Sox2, and Neun, respectively, and counterstained with propidium iodide. (F) Histogram of the percentage of immunoreactive cells (NSC) to nestin, NF-160, Sox2, and Neun. aStatistically different from nestin, NF-160, Sox2.
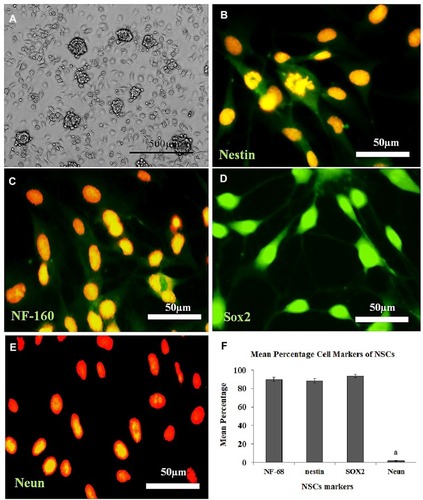
SPIONs Characterization
The finding showed SPIONs were synthesized using co-precipitation of ferric and ferrous chloride. In previous studies, it has been demonstrated that the SPION was about 10 nm in diameter. Evaluation of ADSC after Prussian blue staining showed brown particles in the cytoplasm. The efficiency of label cells with SPION was about 96% of ADSC ().
Figure 4 The morphology of adipose-derived stem cells (ADSC) labeled by Super Paramagnetic Iron Oxide Nanoparticles (SPIONs). (A) ADSCs were fixed in 4% paraformaldehyde and then stained using Prussian blue and counterstained with safranin. (B) shows a high magnification of the arrowhead indicates that the ADSC cytoplasm was covered with iron oxide particles.
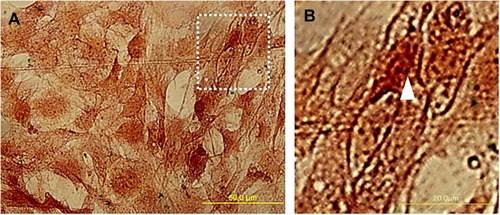
Apomorphine-Induced Rotation
shows the results of the number of rotations affected by the apomorphine injection. In the PD group, the number of rotations in the first, third, and sixth weeks after surgery and cell transplantation were 230.75±2.84, 225.75±3.65 and 222.25±4.2, respectively, so that there was no significant change in the number of rotations in these three tests. However, in the ADSC, ADSC/SPION and ADSC/SPION/EM groups, the number of rotations decreased significantly from week 1 to week 6 (). The comparison between experimental groups at week 6 also showed a significant decrease in rotations in the ADSC/SPION/EM group compared to others (*** and *** show P< 0.05, P<0.01 and P<0.001, respectively).
Figure 5 Diagram of apomorphine-induced rotation test results in test groups shows the test results in the first, third and sixth weeks after cell transplantation in the injury (PD) and treatment groups (ADSC, ADSC/SPION, ADSC/SPION/EM groups), respectively. *, ** and *** show P< 0.05, P<0.01 and P<0.001, respectively.
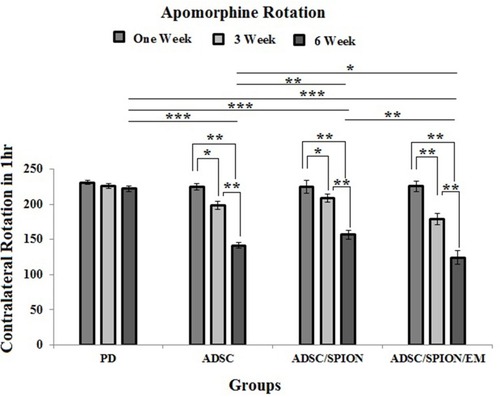
Cell Transplantation
represents the morphology of ADSC label with SPION transfect by GFP and ADSCs labeled by BrdU in a brain section 2 months after transplantation. GFP-positive cells were counted in the substantia nigra (SN) and ventral tegmental area (VTA) in the experimental groups (PD/ADSC, ADSC/SPION, PD/ADSC/SPION/EM groups).
The highest number of GFP-positive cells was found in the ADSC/SPION/EM group, which was significantly different from the number found in the ADSC/SPION group (**P<0.01, ). As well as demonstrates the images of hematoxylin and eosin (H&E) staining of brain histological section for negative control group (without 6-HD injection), PD group, PD treated with ADSCs group, PD treated with ADSCs/SPIONs group, and PD treated with ADSCs/SPIONs/EM group.
Figure 6 The histological section of the brain in the substantia nigra pars compacta. (A and C) show the morphology of transfected ADSC/SPION with GFP (A): ADSC/SPION/EM and (C) ADSC/SPION). (B) shows the ADSC labeled by BrdU, and (D) shows ADSC label by SPION and GFP transfection in an in vitro system for the evaluation of efficiently labeling cells. (E) shows the histogram of the cell viability after transplantation. aStatistically different from ADSC and ADSC/SPION.
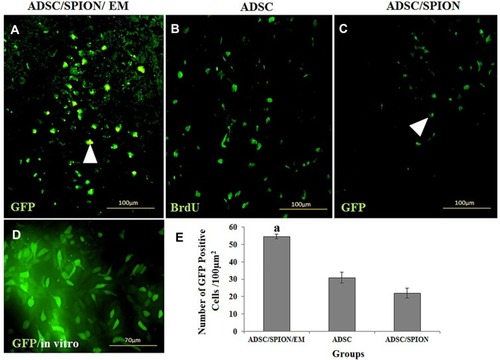
Figure 7 Images of hematoxylin and eosin (H & E) staining of brain sections in SNc and VTA regions in the untreated (PD) groups (A), negative control (B), and ADSC (C), ADSC/SPION (D) and ADSC/SPION/EM (E) test groups. The scale is 100 μm in all images. In addition, Figure (F) shows the number of neural cells counted in SNc and VTA regions with cresyl violet staining. a: Statistically different from PD, ADSC, and ADSC/SPION (P<0.02).
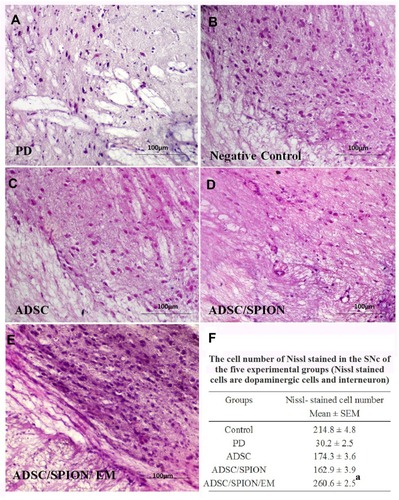
Discussion
Cellular transplantation for the treatment of chronic neurodegenerative diseases such as Alzheimer’s, Huntington’s, and Parkinson’s has been the subject of several preclinical types of research over the past two decades.Citation23–Citation25 These diseases are characterized by progressive loss and depletion of neurons in specific sites of the central nervous system where neural loss caused a lack of the release of neurotransmitters. However, the basic cell treatment in PD is to replace dopaminergic neurons in SN.Citation42 Recent studies have found that several cell types, including MSCs,Citation43 dopaminergic carotid body cells, and fat tissue, induce pluripotent stem cells (iPSCs) and ESCsCitation44–Citation46 that have the potential to differentiate into dopaminergic neuron-like cells. However, the perfect cell sources for neurodegenerative therapy are accessible and abundant. In a previous study, we reported that ADSC could fully differentiate into functional motor-neuron-like cells (MNLC) and express MNLC-specific markers.Citation21 This cell source can also be used autologously to prevent graft rejection and tumorgenesis. In the present study, we used cells from rat ADSCs with the aforementioned abilities for allografts in unilaterally transplanted rats,Citation21,Citation47 with the addition of in vitro labeling of ADSC performed by SPION. Findings showed the ADSCs were immunoreactive to CD90, CD105, and CD49d and negatively immunostained to CD45 as a hematopoietic marker, which is consistent with the results of other studies.Citation21,Citation48 The evaluation of ADSC multipotency is also consistent with our previous research and has the same protocol and differentiated medium.
Migration and integration of transplanted cells into the injured area is known as the homing phenomenon, which is one of the major issues in cell therapy. Cytokines such as CXCR4 induce the migration and homing of MSCs cells.Citation49 There are various techniques to promote stem cell homing. In a study, cell culture was performed in a set of cytokines that affected cell homing, and the findings showed an increase in cell homing in the injured brain region.Citation49 In another study, induction of the surface marker expression (CXCR4-SDF-) that affected cell migration and integration was reviewed and approved.Citation50 In this study, we used SPIONs nanoparticles for directing transplanted cells and homing them to the injury site. In recent years, a variety of nanoparticles have been identified that have been used in drug and gene transfer processes, including platinum, gadolinium-based nanoparticles, gold nanoparticles (AuNPs), iron oxide, cerium oxide nanoparticles, and nanodiamonds.Citation51,Citation52 Kaushik studied a synergistic effect of nanoparticles (AuNPs, MnNPs, and SPIONs) with wgx-50, effective on the inhibition of amyloid-beta 42 (Aβ-42) (Alzheimer’s disease-initiating risk-enhancing protein) and the results showed the inhibitory effect of wgx-50-AuNP on Aβ-42 inhibition.Citation53 However, in another study by this scientist, he compared nanoparticles to SPIONs as suitable candidates for Aβ inhibition compared to four nanoparticles (AuNPs, MnNPs, cobalt and SPIONs) and stated that SPIONs were more efficient than AuNPs because of their energy bands to inhibit Aβ.Citation54
In a study of human neuroprogenitor cells (hNPCs) labeled with a superparamagnetic nanoparticle in traumatic brain injury (TBI), the presence of 0.6 tesla magnet increased homing and retention of cells in the injured area. These findings were in agreement with physiological findings.Citation55 In the present study, the use of SPIONs to label ADSC cells in the external magnet indicates a higher number of GFP-positive cells in the injured area, which is in agreement with the findings of other researchers. However, comparison among GOLD, SPIONs and CeO2 metal nanoparticles by Kaushik in 2018 showed that CeO2 nanoparticle interacted best with α-synuclein and could inhibit its production and could be used as a nanoparticle in Parkinson’s disease.Citation56 On one hand, SPIONs nanoparticles with ROS production can lead to toxicity and affect mitochondrial function, thus affect cell death by the degradation of iron ions and their interaction with hydrogen peroxide. On the other hand, free iron by inducing ROS induces Glycogen synthase kinase 3 inhibitor, which leads to cell migration through the gap between endothelial cells in the injured area by triggering the signaling pathway Akt. In addition, Akt leads to insulin signaling and growth factors via the PI3-Kinase, involved in the process of carbohydrate and protein metabolism as well as transcription, which is effective in cell growth and proliferation.Citation57,Citation58 In a study, Huang showed that a superparamagnetic nanoparticle could promote the growth of human mesenchymal stem cell, by reducing intracellular H2O2 through peroxidase-like activity. Furthermore, the superparamagnetic nanoparticle also accelerates the progression of the cell cycle, which is controlled by free iron released from lysosomal degradation.Citation59 On the other hand, other studies have shown that superparamagnetic nanoparticles have non-cytotoxic effects at doses below 100 µg/mL. In this study, the findings showed that cells transfected with SPIONs acted similarly to viability, proliferation, and differentiation of ADSC cells and these findings are in agreement with the findings obtained by Ankamwar.Citation60,Citation61
A stable magnetite colloid prepared by true purification is done by magnetite separation in ultrapure water. This was the protocol used in our study and is in agreement with other studies.Citation39,Citation62 Albakhaty et al used this technique for in vitro labeling of neural stem cells. Stem cell labeling with magnetic nanoparticles was suggested for clinical applications (stem cell tracking).Citation39
In previous studies, transmission electron microscope data showed that the average size of a nanoparticle of SPION is 10nm, which seems to be enhanced by PLL and this coating due to an increase in zeta potential. However, evidence suggests that the transfection agent promotes the labeling of SPION to stem cells. Our results are consistent with Albakhaty et al, who showed 0.75 µg/mL of PLL for labeling of NSC by SPION at 0.25 µg/mL is adequate.Citation39 This PLL and SPION concentration prevents the toxic effect on the viability of ADSCs. Findings from this protocol are consistent with the results of a former study that used the PLL transfection agent for enhancing efficient labeling.Citation39,Citation63 Thus, ADSC can be labeled for transplantation studies applied with the PLL transfection agent.
Several protocols have been used to promote the efficacy of cell homing.Citation64,Citation65 The in vivo mechanisms related to stem cell homing are poorly understood. Factors involved include cellular iron oxide concentration, saturation, and distribution as well as the external magnetic field.Citation66,Citation67 We found that rats wearing a magnet on their top of the skull for 2 weeks presented an increasing number of SPION-labeled ADSC transfected by GFP after transplantation in damage site in SN and this finding is consistent with the results of the behavioral test. Song et al reported that using an external magnetic field on the skull of a rat after stem cell intravenous injection to increasein the number of SPION-labeled stem cells homing.Citation68 Moreover, Yun et al showed that a magnetic field could increase SPION-labeled MSC homing.Citation38 In addition, MSCs labeled with nanoparticles under the influence of an external magnetic field demonstrated a difference in an olfactory-injury mouse model.Citation69
Conclusion
One of the main advantages of ADSC transfection by SPION/PLL is the potential use of these cells in neurodegenerative cell therapy, as well as cell therapy of PD can be an option for improving the trend of treatment and quality of life for the patients, there are difficulties for homing of transplanted stem cells. External magnets can be used for the delivery and homing of stem cells in the target tissue.
Acknowledgments
The authors are grateful for the support of the Shefa Neurosciences Research Center at Khatam Al-Anbia Hospital, Tehran, Iran, and the Biotechnology and Medicinal Plants Research Center of Ilam University of Medical Sciences.
Disclosure
The authors report no conflicts of interest in this work.
References
- Cui Y, Yang Z, Teasdale RD. The functional roles of retromer in Parkinson’s disease. FEBS Lett. 2018;592(7):1096–1112. doi:10.1002/feb2.2018.592.issue-729210454
- Zhang PL, Chen Y, Zhang CH, Wang YX, Fernandez-Funez P. Genetics of Parkinson’s disease and related disorders. J Med Genet. 2018;55(2):73–80. doi:10.1136/jmedgenet-2017-10504729151060
- Alexoudi A, Alexoudi I, Gatzonis S. Parkinson’s disease pathogenesis, evolution and alternative pathways: A review. Rev Neurol (Paris). 2018;174(10):699–704. doi:10.1016/j.neurol.2017.12.00330131173
- Kordower JH, Burke RE. Disease modification for parkinson’s disease: axonal regeneration and trophic factors. Mov Disord. 2018;33(5):678–683. doi:10.1002/mds.v33.529603370
- Surmeier DJ. Determinants of dopaminergic neuron loss in Parkinson’s disease. FEBS J. 2018;285(19):3657–3668. doi:10.1111/febs.2018.285.issue-1930028088
- Burciu RG, Vaillancourt DE. Imaging of motor cortex physiology in Parkinson’s disease. Mov Disord. 2018;33(11):1688–1699. doi:10.1002/mds.10230280416
- Grayson M. Parkinson’s disease. Nature. 2016;538(7626):S1. doi:10.1038/538S1a27783582
- Tarakad A, Jankovic J. Diagnosis and management of Parkinson’s Disease. Semin Neurol. 2017;37(2):118–126. doi:10.1055/s-0037-160188828511252
- Kirkeby A, Nolbrant S, Tiklova K, et al. Predictive markers guide differentiation to improve graft outcome in clinical translation of hESC-based therapy for Parkinson’s disease. Cell Stem Cell. 2017;20(1):135–148. doi:10.1016/j.stem.2016.09.00428094017
- Gonzalez R, Garitaonandia I, Poustovoitov M, et al. Neural stem cells derived from human parthenogenetic stem cells engraft and promote recovery in a nonhuman primate model of Parkinson’s disease. Cell Transplant. 2016;25(11):1945–1966. doi:10.3727/096368916X69168227213850
- Tian LP, Zhang S, Xu L, et al. Selenite benefits embryonic stem cells therapy in Parkinson’s disease. Curr Mol Med. 2012;12(8):1005–1014. doi:10.2174/15665241280248088022804247
- Chen D, Fu W, Zhuang W, Lv C, Li F, Wang X. Therapeutic effects of intranigral transplantation of mesenchymal stem cells in rat models of Parkinson’s disease. J Neurosci Res. 2017;95(3):907–917. doi:10.1002/jnr.v95.327617772
- Lebedeva OS, Lagarkova MA. Pluripotent stem cells for modelling and cell therapy of Parkinson’s disease. Biochemistry (Mosc). 2018;83(9):1046–1056. doi:10.1134/S000629791809006730472943
- Altarche-Xifro W, Di Vicino U, Muñoz-Martin MI, et al. Functional rescue of dopaminergic neuron loss in Parkinson’s disease mice after transplantation of hematopoietic stem and progenitor cells. EBioMedicine. 2016;8:83–95. doi:10.1016/j.ebiom.2016.04.01627428421
- Minteer D, Marra KG, Rubin JP. Adipose-derived mesenchymal stem cells: biology and potential applications. Adv Biochem Eng Biotechnol. 2013;129:59–71. doi:10.1007/10_2012_14622825719
- Gimble JM, Katz AJ, Bunnell BA. Adipose-derived stem cells for regenerative medicine. Circ Res. 2007;100(9):1249–1260. doi:10.1161/01.RES.0000265074.83288.0917495232
- Mailey B, Hosseini A, Baker J, et al. Adipose-derived stem cells: methods for isolation and applications for clinical use. Methods Mol Biol. 2014;1210:161–181.25173168
- Okubo T, Hayashi D, Yaguchi T, et al. Differentiation of rat adipose tissue-derived stem cells into neuron-like cells by valproic acid, a histone deacetylase inhibitor. Exp Anim. 2016;65(1):45–51. doi:10.1538/expanim.15-003826411320
- Goudarzi F, Tayebinia H, Karimi J, Habibitabar E, Khodadadi I. Calcium: A novel and efficient inducer of differentiation of adipose-derived stem cells into neuron-like cells. J Cell Physiol. 2018;233(11):8940–8951. doi:10.1002/jcp.2682629870058
- Adib S, Tiraihi T, Darvishi M, Taheri T, Kazemi H. Cholinergic differentiation of neural stem cells generated from cell aggregates-derived from Human Bone marrow stromal cells. J Tissue Eng Regen Med. 2015;12(1):43–52. doi:10.1007/s13770-014-0019-6
- Darvishi M, Tiraihi T, Mesbah-Namin SA, Delshad A, Taheri T. Motor neuron transdifferentiation of neural stem cell from adipose-derived stem cell characterized by differential gene expression. Cell Mol Neurobiol. 2017;37(2):275–289. doi:10.1007/s10571-016-0368-x27107758
- Xie S, Lu F, Tao K, et al. Efficient generation of functional Schwann cells from adipose derived stem cells in defined conditions. Cell Cycle. 2017;16(6):841–851. doi:10.1080/15384101.2017.130432828296571
- Bjorklund LM, Pernaute RS, Chung S, et al. Embryonic stem cells develop into functional dopaminergic neurons after transplantation in a Parkinson rat model. Proc Natl Acad Sci U S A. 2012;99(4):2344–2349. doi:10.1073/pnas.022438099
- Wu QY, Li J, Feng ZT, Wang TH. Bone marrow stromal cells of transgenic mice can improve the cognitive ability of an Alzheimer disease rat model. Neurosci Lett. 2007;417(3):281–285. doi:10.1016/j.neulet.2007.02.09217412501
- Silani V, Fogh I, Ratti A, Sassone J, Ciammola A, Cova L. Stem cells in the treatment of amyotrophic lateral sclerosis (ALS). Amyotroph Lateral Scler Other Motor Neuron Disord. 2002;3(4):173–181. doi:10.1080/14660820276083900112710505
- Vaněček V, Zablotskii V, Forostyak S, et al. Highly efficient magnetic targeting of mesenchymal stem cells in spinal cord injury. Int J Nanomedicine. 2012;7:3719–3730. doi:10.2147/IJN.S3282422888231
- Arbab AS, Jordan EK, Wilson LB, et al. In vivo trafficking and targeted delivery of magnetically labeled stem cells. Hum Gene Ther. 2004;15(4):351–360. doi:10.1089/10430340432295950615053860
- Andreas K, Georgieva R, Ladwig M, et al. Highly efficient magnetic stem cell labeling with citrate-coated superparamagnetic iron oxide nanoparticles for MRI tracking. Biomaterials. 2012;33(18):4515–4525. doi:10.1016/j.biomaterials.2012.02.06422445482
- Ahn YJ, Kong TH, Choi JS, Yun WS, Key J, Seo YJ. Strategies to enhance efficacy of SPION-labeled stem cell homing by magnetic attraction: a systemic review with meta-analysis. Int J Nanomedicine. 2019;14:4849–4866. doi:10.2147/IJN.S20491031308662
- Grüll H, Langereis S. Hyperthermia-triggered drug delivery from temperature-sensitive liposomes using MRI-guided high intensity focused ultrasound. J Control Release. 2012;161(2):317–327. doi:10.1016/j.jconrel.2012.04.04122565055
- Mahmoudi M, Shokrgozar MA, Simchi A, et al. Multiphysics flow modeling and in vitro toxicity of iron oxide nanoparticles coated with poly(vinyl alcohol). J Phys Chem C. 2009;113(6):2322–2331. doi:10.1021/jp809453v
- Weissleder R, Stark DD, Engelstad BL, et al. Superparamagnetic iron oxide: pharmacokinetics and toxicity. AJR Am J Roentgenol. 1989;152(1):167–173. doi:10.2214/ajr.152.1.1672783272
- Longmire M, Choyke PL, Kobayashi H. Dendrimerbased contrast agents for molecular imaging. Curr Top Med Chem. 2008;8(14):1180–1186. doi:10.2174/15680260878584902118855704
- Yocum GT, Wilson LB, Ashari P, Jordan EK, Frank JA, Arbab AS. Effect of human stem cells labeled with ferumoxides-poly-L-lysine on hematologic and biochemical measurements in rats. Radiology. 2005;235(2):547–552. doi:10.1148/radiol.235204038315858093
- Wilhelm C, Gazeau F, Bacri HC. Magnetophoresis and ferromagnetic resonance of magnetically labeled cells. Eur Biophys J. 2002;31(2):118–125. doi:10.1007/s00249-001-0200-412012115
- Landázuri N, Tong S, Suo J, et al. Magnetic targeting of human mesenchymal stem cells with internalized superparamagnetic iron oxide nanoparticles. Small. 2013;9(23):4017–4026. doi:10.1002/smll.20130057023766267
- Cheng K, Malliaras K, Li TS, et al. Magnetic enhancement of cell retention, engraftment, and functional benefit after intracoronary delivery of cardiac-derived stem cells in a rat model of ischemia/ reperfusion. Cell Transplant. 2012;21(6):1121–1135. doi:10.3727/096368911X62738122405128
- Yun W, Choi J, Ju H, et al. Enhanced homing technique of mesenchymal stem cells using iron oxide nanoparticles by magnetic attraction in olfactory-injured mouse models. Int J Mol Sci. 2018;19(5):1376. doi:10.3390/ijms19051376
- Albukhaty S, Naderi-Manesh H, Tiraihi T. In vitro labeling of neural stem cells with poly-l-lysine coated super paramagnetic nanoparticles for green fluorescent protein transfection. Iran Biomed J. 2013;17(2):71–76. doi:10.6091/ibj.1114.201323567848
- Ungerstedt U. Postsynaptic supersensitivity after 6-hydroxy-dopamine induced degeneration of the nigro-striatal dopamine system. Acta Physiol Scand Suppl. 1971;367:69–93. doi:10.1111/j.1365-201X.1971.tb11000.x4332693
- Moayeri A, Khalili Z, Darvishi M. Sexually dimorphic effect of Zonisamide on behavioral locomotor activity in a rat model of Parkinson’s disease. Int J Pharm Phytopharm Res. 2019;9(5):19–28.
- Berg DA, Belnoue L, Song H, Simon A. Neurotransmitter-mediated control of neurogenesis in the adult vertebrate brain. Development. 2013;140(12):2548–2561. doi:10.1242/dev.08800523715548
- Danielyan L, Beer-Hammer S, Stolzing A, et al. Intranasal delivery of bone marrow-derived mesenchymal stem cells, macrophages, and microglia to the brain in mouse models of Alzheimer’s and Parkinson’s disease. Cell Transplant. 2014;23(1):S123–S139. doi:10.3727/096368914X68497025302802
- Okano H, Yamanaka S. iPS cell technologies: significance and applications to CNS regeneration and disease. Mol Brain. 2014;7:22. doi:10.1186/1756-6606-7-2224685317
- Yasuhara T, Matsukawa N, Hara K, et al. Transplantation of human neural stem cells exerts neuroprotection in a rat model of Parkinson’s disease. J Neurosci. 2006;26(48):12497–12511. doi:10.1523/JNEUROSCI.3719-06.200617135412
- Pluchino S, Zanotti L, Rossi B, et al. Neurosphere-derived multipotent precursors promote neuroprotection by an immunomodulatory mechanism. Nature. 2005;436(7048):266–271. doi:10.1038/nature0388916015332
- Baer PC, Geiger H. Adipose-derived mesenchymal stromal/stem cells: tissue localization, characterization, and heterogeneity. Stem Cells Int. 2012;2012:812693. doi:10.1155/2012/81269322577397
- Tapp H, Hanley EN Jr, Patt JC, Gruber HE. Adipose-derived stem cells: characterization and current application in orthopaedic tissue repair. Exp Biol Med (Maywood). 2009;234(1):1–9. doi:10.3181/0805-MR-17019109553
- François S, Bensidhoum M, Mouiseddine M, et al. Local irradiation not only induces homing of human mesenchymal stem cells at exposed sites but promotes their widespread engraftment to multiple organs: a study of their quantitative distribution after irradiation damage. Stem Cells. 2006;24(4):1020–1029. doi:10.1634/stemcells.2005-026016339642
- Van der Meeren A, Mouthon MA, Vandamme M, Squiban C, Aigueperse J. Combinations of cytokines promote survival of mice and limit acute radiation damage in concert with amelioration of vascular damage. Radiat Res. 2004;161(5):549–559. doi:10.1667/RR316415161368
- Kandasamy G, Maity D. Recent advances in super paramagnetic iron oxide nanoparticles (SPIONs) for in vitro and in vivo cancer nanotheranostics. Int J Pharm. 2015;496:191–218. doi:10.1016/j.ijpharm.2015.10.05826520409
- Ahmad J, Akhter S, Rizwanullah M, et al. Nanotechnology based theranostic approaches in Alzheimer’s disease management: current status and future perspective. Curr Alzheimer Res. 2017;14(11):1164–1181. doi:10.2174/156720501466617050812103128482786
- Kaushik A, Kumar A, Peng Z, et al. Evaluation and validation of synergistic effects of amyloid-beta inhibitor–gold nanoparticles complex on Alzheimer’s disease using deep neural network approach. J Mater Res. 2019;34(11):1845–1853. doi:10.1557/jmr.2018.452
- Kaushik AC, Kumar A, Dwivedi VD, et al. Deciphering the biochemical pathway and pharmacokinetic study of amyloid βeta-42 with Superparamagnetic Iron Oxide Nanoparticles (SPIONs) using systems biology approach. Mol Neurobiol. 2018;55(4):3224–3236. doi:10.1007/s12035-017-0546-y28478508
- Shen WB, Plachez C, Tsymbalyuk O, et al. Cell-based therapy in TBI: magnetic retention of neural stem cells in vivo. Cell Transplant. 2016;25(6):1085–1099. doi:10.3727/096368915X68955026395573
- Kaushik AC, Bharadwaj S, Kumar S, Wei DQ. Nano-particle mediated inhibition of Parkinson’s disease using computational biology approach. Sci Rep. 2018;8(1):9169. doi:10.1038/s41598-018-27580-129907754
- Apopa PL, Qian Y, Shao R, et al. Iron oxide nanoparticles induce human microvascular endothelial cell permeability through reactive oxygen species production and microtubule remodeling. Part Fibre Toxicol. 2009;6:1. doi:10.1186/1743-8977-6-119134195
- Huang G, Chen H, Dong Y, et al. Superparamagnetic iron oxide nanoparticles: amplifying ROS stress to improve anticancer drug efficacy. Theranostics. 2013;3(2):116–126. doi:10.7150/thno.541123423156
- Huang DM, Hsiao JK, Chen YC, et al. The promotion of human mesenchymal stem cell proliferation by superparamagnetic iron oxide nanoparticles. Biomaterials. 2009;30(22):3645–3651. doi:10.1016/j.biomaterials.2009.03.03219359036
- Gokduman K, Bestepe F, Li L, Yarmush ML, Usta OB. Dose-, treatment- and time-dependent toxicity of superparamagnetic iron oxide nanoparticles on primary rat hepatocytes. Nanomedicine (Lond). 2018;13(11):1267–1284. doi:10.2217/nnm-2017-038729949471
- Ankamwar B, Lai TC, Huang JH, et al. Biocompatibility of Fe(3)O(4) nanoparticles evaluated by in vitro cytotoxicity assays using normal, glia and breast cancer cells. Nanotechnology. 2010;21(7):75102. doi:10.1088/0957-4484/21/7/07510220090199
- Sapet C, Laurent N, de Chevigny A, et al. High transfection efficiency of neural stem cells with magnetofection. Biotechniques. 2011;50(3):187–189. doi:10.2144/00011362821486240
- Hartono SB, Gu W, Kleitz F, et al. Poly-L-lysine functionalized large pore cubic mesostructured silica nanoparticles as biocompatible carriers for gene delivery. ACS Nano. 2012;6(3):2104–2117. doi:10.1021/nn203964322385282
- Tarulli E, Chaudhuri JD, Gretka V, Hoyles A, Morshead CM, Stanisz GJ. Effectiveness of micron-sized superparamagnetic iron oxide particles as markers for detection of migration of bone marrow-derived mesenchymal stromal cells in a stroke model. J Magn Reson Imaging. 2013;37:1409–1418. doi:10.1002/jmri.2389723712844
- Arora S, Jyutika MR, Kishore MP. Nanotoxicology and in vitro studies: the need of the hour. Toxicol Appl Pharmacol. 2012;258:151–165. doi:10.1016/j.taap.2011.11.01022178382
- Hoehn M, Küstermann E, Blunk J, et al. Monitoring of implanted stem cell migration in vivo: a highly resolved in vivo magnetic resonance imaging investigation of experimental stroke in rat. Proc Natl Acad Sci U S A. 2002;99(25):16267–16272. doi:10.1073/pnas.24243549912444255
- Laurent S, Mahmoudi M. Superparamagnetic iron oxide nanoparticles: promises for diagnosis and treatment of cancer. Int J Mol Epidemiol Genet. 2011;2(4):367–390.22199999
- Song M, Kim YJ, Kim YH, Roh J, Kim SU, Yoon BW. Using a neodymium magnet to target delivery of ferumoxide-labeled human neural stem cells in a rat model of focal cerebral ischemia. Hum Gene Ther. 2010;21(5):603–610. doi:10.1089/hum.2009.14420059319
- Marycz K, Kornicka K, Röcken M. Static Magnetic Field (SMF) as a regulator of stem cell fate - new perspectives in regenerative medicine arising from an underestimated tool. Stem Cell Rev Rep. 2018;14(6):785–792. doi:10.1007/s12015-018-9847-430225821