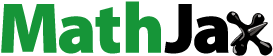
Abstract
Background
Nanoliposomes are designed as carriers capable of packaging drugs through passive targeting tumor sites by enhanced permeability and retention (EPR) effects. In the present study the biodistribution, pharmacokinetics, micro single-photon emission computed tomography (micro-SPECT/CT) image, dosimetry, and therapeutic efficacy of 188Re-labeled nanoliposomes (188Re-liposomes) in a C26 colonic peritoneal carcinomatosis mouse model were evaluated.
Methods
Colon carcinoma peritoneal metastatic BALB/c mice were intravenously administered 188Re-liposomes. Biodistribution and micro-SPECT/CT imaging were performed to determine the drug profile and targeting efficiency of 188Re-liposomes. Pharmacokinetics study was described by a noncompartmental model. The OLINDA|EXM® computer program was used for the dosimetry evaluation. For therapeutic efficacy, the survival, tumor, and ascites inhibition of mice after treatment with 188Re-liposomes and 5-fluorouracil (5-FU), respectively, were evaluated and compared.
Results
In biodistribution, the highest uptake of 188Re-liposomes in tumor tissues (7.91% ± 2.02% of the injected dose per gram of tissue [%ID/g]) and a high tumor to muscle ratio (25.8 ± 6.1) were observed at 24 hours after intravenous administration. The pharmacokinetics of 188Re-liposomes showed high circulation time and high bioavailability (mean residence time [MRT] = 19.2 hours, area under the curve [AUC] = 820.4%ID/g*h). Micro-SPECT/CT imaging of 188Re-liposomes showed a high uptake and targeting in ascites, liver, spleen, and tumor. The results were correlated with images from autoradiography and biodistribution data. Dosimetry study revealed that the 188Re-liposomes did not cause high absorbed doses in normal tissue but did in small tumors. Radiotherapeutics with 188Re-liposomes provided better survival time (increased by 34.6% of life span; P < 0.05), tumor and ascites inhibition (decreased by 63.4% and 83.3% at 7 days after treatment; P < 0.05) in mice compared with chemotherapeutics of 5-fluorouracil (5-FU).
Conclusion
The use of 188Re-liposomes for passively targeted tumor therapy had greater therapeutic effect than the currently clinically applied chemotherapeutics drug 5-FU in a colonic peritoneal carcinomatosis mouse model. This result suggests that 188Re-liposomes have potential benefit and are safe in treating peritoneal carcinomatasis of colon cancer.
Introduction
Colorectal cancer is one of the most common types of cancer in men and women. Its prognosis depends on the extent of the disease and only about 40% of patients with colorectal cancers are diagnosed at the early stage.Citation1 At this stage, surgical tumor resection is the main method of treatment and the 5-year survival rate of patients can reach 90%. If the cancer has progressed, there is lymph node involvement and development of hematogenous metastases, so that prognosis becomes poor.Citation2 Peritoneal dissemination is considered the terminal stage in colorectal cancer. “Peritoneal carcinomatosis” means extensive or widespread dissemination of tumor nodules inside the abdomen. Certain sites in the abdomen are more prone to implantation of tumor cells, including the greater omentum, the hepatic hilum, the mesentery, the pelvis, and the peritoneum covering the diaphragm.Citation3,Citation4 Peritoneal carcinomatosis is also the most common cause of malignant ascites; the production and leakage of fluid from the malignant cells causes exudation of extracellular fluid into the peritoneal cavity, in turn causing discomfort, pain, and symptoms that diminish the quality of life for cancer patients.Citation5
The treatment of peritoneal or distant metastasis from colorectal cancer remains a challenging problem.Citation2 For several decades, regimens based on 5-fluorouracil (5-FU) have been the first choice for primary or adjuvant chemotherapy for colorectal cancer.Citation6,Citation7 5-FU belongs to the family of drugs called “antimetabolites” and works through noncompetitive inhibition of thymidylate synthase. In clinical standard care for first-line therapy, patients with metastatic colorectal cancer receiving systemic chemotherapy of 5-FU and leucovorin have a median survival that is increased from approximately 6 months to about 12 months.Citation7 In recent years, a number of new treatments have become available for patients with advanced colorectal cancer. The effectiveness of these drugs has been compared with that of 5-FU in clinical studies.Citation2,Citation8 However, no effective therapy for peritoneal metastasis is yet available, though novel agents remain under investigation. In preclinical drug development, 5-FU also serves as the comparative object to evaluate the anticancer efficacy for colon cancer.Citation9,Citation10
Several therapeutics based on nanoparticles have been successfully introduced for cancer treatment. The first generation of nanoparticles is primarily based on liposomes and polymer-drug conjugates.Citation11 Nanoliposomes are double-membrane lipid vesicles capable of packaging drugs for various delivery applications. Nanopegylated liposomes can evade the reticuloendothelial system and remain in the circulation for prolonged periods, improving tumor targeting and efficacy in animal models. Nanopegylated liposomes provide passive targeting because of nanoliposome accumulation in tumors by means of the enhanced permeability and retention (EPR) effect through leaky tumor vasculature.Citation12,Citation13 Preclinical studies have shown that cytotoxic agents entrapped in pegylated liposomes tend to accumulate in tumors.Citation14,Citation15 A prominent example is pegylated liposomal doxorubicin, which has been applied in patients with acquired immune deficiency syndrome–related Kaposi’s sarcoma (ARKS), as well as ovarian, breast, and prostate carcinomas.Citation16–Citation18
Preclinical studies of tumor therapy with radionuclide-liposome conjugates or liposome-mediated radiotherapeutics have been reported.Citation19 Rhenium-188 (188Re) is a radionuclide used for imaging and therapeutic dual applications due to its short physical half-life of 16.9 hours with 155 keV gamma emissions for imaging, and its 2.12 MeV β emission with a maximum tissue penetration range of 11 mm for tumor therapeutics. In addition, 188Re can be obtained from commercial nuclear generators, which makes it convenient for routine research and clinical use.Citation20
In this study, the biodistribution, pharmacokinetics, and micro single-photon emission computed tomography (micro-SPECT/CT) imaging of intravenous injection of 188Re-liposomes were investigated in a C26 colonic peritoneal carcinomatosis mouse model. The therapeutic efficacy of 188Re-liposomes was compared with that of 5-FU. The experimental goals were to demonstrate the potential applications of nanotargeted 188Re-liposomes via an intravenous route for internal radiotherapy of peritoneal carcinomatosis and ascites.
Materials and methods
Materials
Pegylated liposomes (Nano-X) were provided by Taiwan Liposome Company (Taipei, Taiwan). The tungsten-188 (188 W)/188Re generator was purchased from Oak Ridge National Laboratory (Oak Ridge, TN). Elution of the 188 W/188Re generator with normal saline provided solutions of carrier-free 188Re as sodium perrhenate (NaReO4). N,N-bis(2-mercaptoethyl)-N′,N′-diethylethylenediamine (BMEDA) were purchased from ABX (Radeberg, Germany). The C26 murine colon carcinoma cell line was obtained from the American Type Culture Collection (Manassas, VA). Stannous chloride (SnCl2) was purchased from MERCK (Darmstadt, Germany). The PD-10 column was purchased from GE Healthcare (Uppsala, Sweden). All other chemicals were purchased from MERCK.
Cell culture and animal tumor ascites model
C26 murine colon carcinoma cell line was obtained from the American Type Culture Collection (Manassas). It was grown in Roswell Park Memorial Institute medium– 1640 supplemented with 10% (v/v) fetal bovine serum, 2 mM L-glutamine at 37°C in 5% CO2. Male BALB/c mice were obtained from the National Laboratory Animal Center, Taipei, Taiwan. Mice were housed in a controlled environment, with food and water provided ad libitum. One hundred and seventy-one mice (6–8 weeks old) were inoculated intraperitoneally with 2 × 105 C26 cells in 500 μL phosphate-buffered saline. Mice were sacrificed by CO2 asphyxiation at the desired time points after tumor inoculation. The abdominal cavity was carefully inspected and all ascites and tumor nodules were meticulously collected and weighed. Animal protocols were approved by the Institutional Animal Care and Use Committee at the Institute of Nuclear Energy Research, Taiwan.
Preparation of 188Re-BMEDA-labeled pegylated liposomes (188Re-liposomes)
The pegylated liposomes (Nano-X) were prepared according to the method described by Tseng et al.Citation21 The lipid compositions of liposome contain hydrogen soybean phosphatidylchloine (HSPC), cholesterol, polyethylene glycol-(1,2-distearoyl-sn-glycero- 3-phosphatidylethanolamine) (PEG-DSPE; molar ratio 3:2:0.3) and ammonium sulfate solution with [250 mM (NH4)2SO4, pH 5.0] in the inner water phase. Pegylated nanoliposomes have an average particle size of about 82.59 nm and contain 13.16 μmole/mL phospholipids.
The method for radiolabeling BMEDA with 188Re was as has been previously described.Citation22 Briefly, BMEDA (ABX), stannous chloride was used as the reductant and glucoheptonate (Sigma-Aldrich, St Louis, MO) was used as an intermediate ligand to make 188Re-SNS/S complexes. Five milligrams of BMEDA were pipetted into a fresh vial. A volume of 0.5 mL of 0.17 mol/L glucohepatonate dissolved in a 10% acetate solution was added, followed by the addition of 120 μL (10 μg/μL) of stannous chloride. After flushing the solution with N2 gas, highly specific activity of 188Re-sodium perrhenate was added. The vial was sealed and heated in an 80°C water bath for 1 hour. The labeling efficiency of 188Re-BMEDA complexes was analyzed by silica gel–impregnated glass fiber sheets using normal saline as the developer.
The Nano-X pegylated liposomes (1 mL) were added to the 188Re-BMEDA (50–250 MBq) solution and incubated at 60°C for 30 minutes. The 188Re-liposomes were separated from free 188Re-BMEDA using a PD-10 column eluted with normal saline. Each 0.5 mL fraction was collected into a tube. The opacity of pegylated liposomes was used to visually monitor the collection of the 188Re-liposomes. The labeling efficiency was determined using the activity in pegylated liposomes after separation, divided by the total activity before separation.
Biodistribution and pharmacokinetic studies
Thirty-five C26 colon peritoneal metastatic carcinoma-bearing mice (22–26 g, average weight 24.6 g) received an intravenous injection of 3.7 MBq/200 μL of 188Re-liposomes with 0.88 μmol phospholipid for biodistribution and pharmacokinetic studies. Mice were kept on a 12-hour day/night cycle and had free access to food and water. For biodistribution study, 30 mice were sacrificed by CO2 asphyxiation, five mice each at 1, 4, 16, 24, 48, and 72 hours after administration. At each time point, the organs of interest were sampled and whole organs collected where possible. The samples were rinsed in saline, blotted dry, weighed, and then counted using a Packard Cobra II Auto-Gamma (PerkinElmer, Inc, Waltham, MA) counter. Samples of the injection of 188Re-liposomes were used as decay correction standards. Data were expressed as percent of the injected dose (ID) per gram of tissue (%ID/g).
For pharmacokinetics, blood samples of 20 to 100 μL were collected at 0.083 (5 minutes), 0.5, 1, 4, 16, 24, 48, 72, 120, and 168 hours from five mice via heart puncture after intravenous injection of 188Re-liposomes (20 μL at 0.083, 0.5, 1, 4, 16, 24 hours; 50 μL at 48, 72, 120 hours; 100 μL at 168 hours). Concentrations of radioactivity in the blood were expressed as %ID/mL. Pharmacokinetic parameters were determined using WinNonlin (v 5.0.1; Pharsight Corp, Mountain View, CA) software. Noncompartmental analysis model 200 (extravascular input) was used with the Log/linear trapezoidal rule. Parameters, including terminal half-life (T1/2λz), Tmax, Cmax, total body clearance (Cl) and area under the curve (AUC) were determined. Pharmacokinetic parameters associated with the terminal phase were calculated using the best-fit method to estimate the terminal half-life.
Micro-SPECT/CT imaging and wholebody autoradiography (WBAR)
For micro-SPECT/CT imaging, three mice received an intravenous injection of 12.95 MBq/200 μL of 188Re-liposomes and 0.88 μmol phospholipid. The mice were anesthetized with 1.5% isoflurine (positioned prone in the scanner) and received micro-SPECT/CT imaging at 72 hours after intravenous injection. SPECT and CT images were acquired using a micro-SPECT/CT scanner (X-SPECT/CT, Gamma Medica, Northridge, CA). The SPECT images were acquired using a parallel hole collimator, with the center of the field of view (FOV) focused on the abdomen of each mouse. The radius of rotation was 1 cm with an FOV of 1.37 cm. The imaging was accomplished using 64 projections at 60 seconds per projection. SPECT imaging was followed by CT image acquisition (X-ray source: 50 kVp, 0.4 mA; 256 projections). The software provided with the X-SPECT/CT scanner was used for the SPECT and CT image reconstruction including the SPECT/CT image fusion. SPECT images were reconstructed to produce image sizes of 56 × 56 × 56 pixels with an image resolution of 1.2 mm. CT images were also reconstructed in image sizes of 512 × 512 × 512 pixels with 0.15 mm image resolution.
The procedure for WBAR has been previously described. Mice were sacrificed by CO2 euthanasia at 72 hours after micro-SPECT/CT imaging and were immediately dipped into liquid nitrogen. The frozen carcasses were then embedded with 2.5% carboxymethyl cellulose and the frozen carboxymethyl cellulose block was attached to the sample stage in the cryo-chamber (−20°C). After resting for 2 hours, the frozen sample was then sectioned (into slices 40 μm thick) using a cryomicrotome (CM 3600; Leica Microsystems GmbH, Wetzlar, Germany) at −20°C. These samples were placed in contact with an imaging plate (BAS-MS 2040; Fuji Photo Film Co) for 5 days. After completing the exposure, the imaging plate was analyzed with a FLA-5100 (Fuji Photo Film Co) reader and the Multi Gauge v 3.0 (Fuji Photo Film Co) software.
Absorbed radiation dose calculations
To estimate mean absorbed doses in humans, the relative organ mass scaling method was used. The mean absorbed dose in various tissues is derived from the radionuclide concentration in tissues/organs of interest, assuming a homogeneous distribution of the radionuclide within any source region. The calculated mean value of %IA/g for the organs in mice was extrapolated to uptake in organs of a 70 kg adult using the formula:
The extrapolated values (%IA) in human organs at 1, 4, 16, 24, 48, and 72 hours were fitted with exponential biokinetic models and integrated to obtain the number of disintegrations in the source organs; this information was entered into the OLINDA|EXM® (v 1.0; Vanderbilt University, Nashville, TN) program. The integrals (MBq-s) for 14 organs, including heart contents (blood), brain, muscle, bone, heart, lung, testes, spleen, pancreas, kidneys, liver, stomach, small intestine, lower intestine, and remainder of the body, were evaluated and used for the dosimetry evaluation.
For excretion study, mice were kept in metabolic cages and urine and feces were collected at various time intervals. Cumulative excretion was calculated as %ID. All feces and urine during the study period were collected for estimation of fraction and biological half-life of elimination. Cumulative excretion curves (urine and feces) were fitted from 0 to 168 hours with a function of the form: ft = f*(1−exp(−λ*t)) using SigmaPlot® (Systat Software Inc, San Jose, CA) software. The ICRP 30 GI Model and Voiding Urinary Bladder Model in OLINDA|EXM were used to estimate the number of disintegrations occurring in the excretory organs. For gastrointestinal (GI) excretion, the fraction (f) entering at the small intestine was entered into the GI model module. For bladder excretion, the fraction passing bladder and biological half-life was entered in the Bladder Model. Activity in the remainder of the body was defined as the total injected activity minus the total excretion at any time (urine and feces) and activity in all individual organs evaluated. This activity was assumed to be uniformly distributed in the “remainder of the body.”
To estimate the absorbed dose for different tumor sizes ranging from 0.5 to 300 g mass, the mean value of total tumor nodules (%IA/g) obtained by mouse biodistribution study was directly fitted with exponential models to calculate the number of disintegrations, which again was input into OLINDA|EXM using the unit density sphere model.
Maximum tolerated dose
The maximum tolerated dose (MTD) was defined as the activity dose below the dose resulting in either the death of any animal in groups of five animals or a body weight loss of more than 20%.Citation23,Citation24 The toxicity radio-therapeutic 188Re-liposome and chemo-therapeutic 5-FU was monitored by MTD study. Fifty male BALB/c mice were randomly grouped into cages of five mice (each mouse weighing 24.3 g on average). Mice were given 14.8, 22.2, 29.6, 37, and 44.4 MBq of 188Re-liposomes and 90, 120, 150, 180, and 210 mg/kg of 5-FU in 200 μL by single intravenous injection, respectively. Mice were weighed twice per week, and the survival rate of the mice was recorded daily. The drug-induced toxicities (lethality and body weight loss) were determined for a minimum of 4 weeks.
Therapeutic efficacy studies
At 7 days after intraperitoneal tumor cell inoculation, three groups of ten mice (each weighing 24.1 g on average) received intravenous injections of 200 μL 188Re-liposomes (80% MTD, 29.6 MBq, and 0.88 μmol phospholipid), 144 mg/kg (80% MTD) 5-FU dissolved in 200 μL normal saline, and 200 μL normal saline (control) respectively. Mice were checked for survival every day, and body weight was measured twice a week. The abdominal cavity was conscientiously inspected. The median survival time was carefully estimated using the Kaplan–Meier method of survival analysis for each treatment group. A Log-rank test was used to compare survival between treatment groups. The median survival time presented in the efficacy studies is calculated as the smallest survival time for which the survivor function is equal to 0.5.
To investigate the effects of 188Re-liposomes on the tumor growth and ascites formation, 16 mice per group were intravenously treated with 188Re-liposomes, 5-FU, and normal saline, respectively (doses as described above) at 7 days after intraperitoneal tumor cell inoculation. Four mice per group were sacrificed by CO2 asphyxiation at 7, 9, 12, and 14 days after tumor inoculation. The abdominal cavity was conscientiously inspected. All ascites and tumor nodules were meticulously collected and weighed.
Statistical analysis
Data were expressed as mean ± standard error of the mean. SPSS 11 (SPSS Inc, Armonk, NY) software was used to perform statistical analysis, and the survival data were estimated by the Kaplan–Meier method and compared by Log-rank test. Values of P < 0.05 were considered significant. The uptake value of drug in a tumor was compared by t-test. Values of P < 0.05 were considered significant.
Results
Biodistribution studies of 188Re-liposomes
The biodistribution of 188Re-liposomes in the peritoneal carcinomatosis mouse model was studied at 1, 4, 24, 48, and 72 hours after intravenous injection and the results are summarized in . The 188Re-liposomes in blood circulation were still at a high level of 11.2% ± 1.17% ID/g at 24 hours postinjection. Most normal organs reached the maximum levels at 4 hours postinjection. Uptakes of 188Reli-posomes in normal organs were observed mainly in the spleen, kidney, and liver. Radioactivity in spleen, kidney, and liver reached the highest levels of 10.4% ± 0.7% ID/g, 9.98% ± 0.45% ID/g, and 10.2% ± 0.57% ID/g, respectively. After systemic administration of 188Re-liposomes, radioactivity localized in ascites appeared at 1 hour and was maintained at higher levels from 15.5% ± 0.74% ID/g at 4 hours to 10.8% ± 1.27% ID/g at 24 hours. The radioactivity in total tumor nodules rapidly accumulated to a high level of 7.23% ± 1.39% ID/g at 4 hours and progressively achieved a maximum of 7.91% ± 2.02% ID/g at 24 hours after intravenous administration of the 188Re-liposomes. According to the anatomical studies, C26 tumor nodules spread throughout the peritoneal cavity, with most nodules appearing around the greater omentum, mesentery, diaphragm, and liver hilum at 10 days after inoculation. The uptakes of 188Re-liposomes in tumor nodules collected from greater omentum, mesentery, and diaphragm were similar. Although the radioactivity in tumor nodules sampled from liver hilum was slightly less, there was no significant difference compared with levels from other implanted sites. The Tu to Mu ratio of 188Re-liposomes reached 30.6 ± 9.3 and 25.8 ± 6.1 at 16 hours and 24 hours after injection, respectively. Very low levels of 188Re-liposome uptake were noted in organs of the central nervous and musculoskeletal systems.
Table 1 Biodistribution of 188Re-liposome after intravenous injection in C26 colonic peritoneal carcinomatosis mice
Pharmacokinetics studies of 188Re-liposome
The radioactivity–time curves from blood versus time points after intravenous injection of 188Re-liposome are shown in . Pharmacokinetic parameters in the peritoneal carcinomatosis mice model were estimated using WinNolin and a noncompartmental model (Rsq = 0.9554), as summarized in . The maximum radioactivity (Cmax) in blood was determined as 47.1% ID/mL at 10 minutes for 188Re-liposomes, which was also the Tmax in the blood. The terminal half-life (T1/2λz) of 188Re-liposomes was 16.3 hours after intravenous injection. The AUCo→∞ of 188Re-liposomes was 820.4% ID/g*h. The Cl of 188Re-liposomes was 0.12 mL/h. The mean residence time (MRTo→∞) of 188Re-liposomes was 19.2 hours. These results revealed that 188Re-liposomes exhibited high bioavailability and that long circulation of nanoliposome therapeutics was achieved in the peritoneal carcinomatosis mice model.
Table 2 Pharmacokinetic parameters of 188Re-liposome uptake after intravenous injection in C26 colonic peritoneal carcinomatosis mice
Micro-SPECT/CT imaging and WBAR
To confirm the specific targeting of tumor sites and ascites of 188Re-liposomes, microSPECT/CT imaging and WBAR were performed. The micro-SPECT/CT images of C26 tumor and ascites-bearing mice (on day 7 after tumor inoculation) acquired at different times after intravenous injection of 188Re-liposomes are shown in . 188Re-liposomes showed slow blood clearances and maintained a relatively higher activity in the abdominal cavity before 24 hours. Micro-SPECT/CT images showed a high uptake and targeting of 188Re-liposomes in ascites and also in the tumor, liver, and feces. WBAR was performed followed by the Micro-SPECT/CT scanning at 72 hours. The WBAR image also confirmed the tumor targeting of microSPECT/CT results as shown in , which is consistent with the biodistribution data ().
Figure 2 (A) The micro single-photon emission computed tomography (micro-SPECT/CT) of 188Re-liposome-treated C26 peritoneal metastatic tumor-bearing mice. The 188Re-liposomes, containing 12.95 MBq of 188Re, were administered to each mouse by intravenous injection. Micro-SPECT images were acquired at 1, 4, 24, 48, and 72 hours after injection. (B) The whole-body autoradiography image was followed by micro-SPECT image acquisition at 72 hours after 188Re-liposome injection, with the animal in the same position. Tumor nodules are indicated by arrows.
Abbreviations: Li, liver; As, ascites; He, heart; Fe, feces; Te, testis; Bl, bladder.
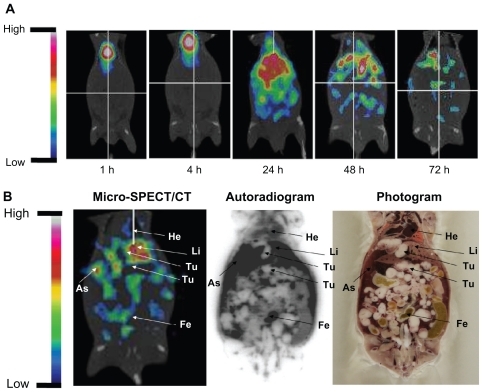
Tissue radiation dose calculations
The radiation-absorbed dose projections for administration of 188Re-liposomes to humans, determined from the residence times in mice, are shown in . The fractions of the 188Re-liposomes excreted in the urinary and GI tract derived from accumulative urine and feces were 12% and 48% ID, respectively. That total excretion was 60% ID and was mostly through feces suggests the importance of hepatobiliary excretion for 188Re-liposomes. Lower doses were found, however, in other important therapy-limiting normal tissues such as the heart, lungs, liver, kidneys, and red marrow with values of 0.40 mSv/MBq, 0.17 mSv/MBq, 0.24 mSv/MBq, 0.20 mSv/MBq, and 0.04 mSv/MBq, respectively. The effective dose appears to be approximately 0.80 mSv/MBq. Tumor radiation absorbed dose calculations are shown in . The unit density sphere model was used for calculation. Results revealed significantly greater absorption in tumor tissue than in normal organs. For a 10 g tumor, the estimated absorbed dose was 65.7 mSv/MBq.
Table 3 Radiation dose estimates for 188Re-liposome in humans
Table 4 Absorbed doses of 188Re-liposomes in sphere tumor derived from C26 peritoneal metastatic tumor
Therapeutic efficacy studies
In MTD study, the maximum dose with weight loss <20% of 188Re-liposomes and 5-FU was determined to be 37 MBq and 180 mg/kg, respectively. In therapeutic efficacy studies, the 80% MTD of each drug was administered in each treatment group. summarizes the therapeutic efficacy for each group receiving different treatments at 7 days after tumor inoculation. The survival curves for different treatment groups are shown in a Kaplan–Meier plot in . Mice were treated at 7 days after tumor inoculation (n = 10 for each group). The median survival time for the control mice, who received normal saline, was 24.3 days (). The median survival times for the mice treated with 188Re-liposomes (29.6 MBq) and 5-FU (144 mg/kg) were 32.8 and 26.7 days, respectively. The results of the median survival time of the radiotherapeutics of 188Re-liposomes were statistically different from the chemotherapeutics of 5-FU (P = 0.03). The therapeutic studies demonstrated better survival time and therapeutic efficacy for mice who received intravenously administered 188Re-liposomes. The P values for the differences among the survival curves of the various treatment groups are shown in .
Table 5 Comparative therapeutic efficacy of different treatments at 7 days after intraperitoneal C26 peritoneal metastatic tumor inoculation
Tumor and ascites inhibition studies
The first macroscopic C26 tumor deposits in the peritoneal cavity were observed 5 days after intraperitoneal inoculation. Tumor deposits were small (1–3 mm in diameter) and mostly located in the upper abdomen in the great omentum and adjacent to the spleen. At 7–10 days after tumor inoculation, C26 tumor nodules had formed throughout the peritoneal cavity, with most nodules appearing in the great omentum, liver hilum, mesenterium, and diaphragm; ascites also formed. All visible tumor nodules and ascites were sampled and weighed during postmortem examination. The weights of the tumors and ascites are presented as the initial weights at 7 days after tumor inoculation for different groups. After 188Re-liposomes and 5-FU were administered at 7 days after tumor inoculation, significantly large inhibitions of hemorrhagic ascites formation and tumor growth were observed in the 188Re-liposome-treated group in comparison with the 5-FU at 9–14 days (ascites: decrease of 83.3% and 44.9% at 14 days for 188Re-liposome-treated group and 5-FU-treated group, respectively; tumor: decrease of 63.4% and 9.1% at 14 days, respectively; P < 0.05) (). To address the inhibition property of 188Re-liposomes in different metastatic sites, tumor nodules were precisely sampled from the greater omentum, mesentery, liver hilum, and diaphragm. 188Re-liposomes had significantly inhibited tumor growths in these implanted sites. Moreover, in comparison with 5-FU, 188Re-liposomes demonstrated greater inhibition of the tumor growths in mesentery and liver hilum (mesentery: decrease of 61.3% and 12.8% at 14 days for 188Re-liposome-treated group and 5-FU-treated group, respectively; liver hilum: decrease of 87.1% and 5.9% at 14 days, respectively; P < 0.05) (). These results demonstrate better therapeutic efficacy in the inhibition of the progression in peritoneal carcinomatosis for mice that received intravenously administered 188Re-liposomes.
Figure 4 The total ascites (A) and tumors (B) were sampled in the tubes and weighed at 0, 2, 4, and 7 days after administration of 188Re-liposomes (29.6 MBq, ▾), 5-FU (144 mg/kg, ●), and normal saline (○), respectively, by single intravenous injection.
Figure 5 The total tumor nodules derived from (A) greater omentum, (B) diaphragm, (C) liver hilum, and (D) mesentery were sampled in the tubes and weighed at 0, 2, 4, and 7 days after administration of 188Re-liposomes (29.6 MBq, ▾), 5-FU (144 mg/kg, ●), and normal saline (○), respectively, by single intravenous injection.
Notes: Data are expressed as mean ± SE M (n = 4 mice/group/time point); *significant difference between 188Re-liposome and normal saline-treated groups; ♦significant difference between 188Re-liposome- and 5-FU-treated groups.
Abbreviation: SEM, standard error of the mean.
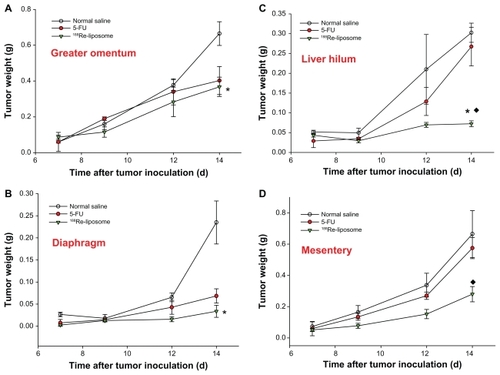
Notes: Data are expressed as mean ± SE M (n = 4 mice/group/time point); *significant difference between 188Re-liposome- and normal saline-treated groups; ♦significant difference between 188Re-liposome- and 5-FU-treated groups.
Abbreviation: SEM, standard error of the mean.
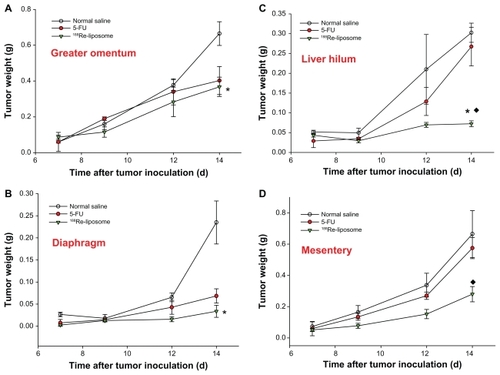

Discussion
Most cases with peritoneal carcionomatosis have no satisfactory response to systemic chemotherapy.Citation8 The main reason for this lack of success is that cytostatic drugs administrated intravenously do not affect tumors of peritoneal metastases with a sufficiently high concentration.Citation25 Little previous research has evaluated the therapeutic efficacy of radionuclide-conjugated liposomes administered intravenously in advanced metastatic colorectal cancer. Colorectal cancer frequently gives rise to the intraperitoneal spread of tumor cells and multiple metastatic tumor nodules are a result of peritoneal carcinomatosis. Initially, these nodules are small volume, with marked neovascularization in the infiltrate sites.Citation26 The tumor-associated vascularization with high permeability is favorable for targeting by liposomal drugs by EPR effect. Therefore, ascertaining the efficacy of passively nanotargeted 188Re-liposomes via the intravenous route in peritoneal carcinomatosis of colorectal origin was worthwhile.
Biodistribution results confirmed the significantly high uptake in tumor tissues and that long blood circulation favored the use of 188Re-liposomes by systemic route in treating peritoneal carcinomatosis. In the authors’ previous report, the biodistribution, and pharmacokinetics of intraperitoneal injected 188Re-liposomes in a peritoneal carcinomatosis mouse model were studied.Citation27,Citation28 Compared with the current results, both administration routes exhibited prolonged retention of 188Re-liposomes within the ascites and tumors. In addition, they achieved the highest uptake of radioactivity in tumor tissues at 24-hours postinjection with a similar level (7.91% ± 2.02% intravenous ID/g versus 6.57% ± 1.71% intraperitoneal ID/g). However, intravenous injection of 188Re-liposomes resulted in faster accumulation in tumor tissues than intraperitoneal injection: 7.23 ± 1.39 ID/g and 3.34 ± 1.24 ID/g at 4 hours after injection, respectively. The level of radioactivity of 188Re-liposomes in blood was maintained at relatively steady high levels for 24 hours after intravenous injection ().
It was noticed that after the redistribution of systemic circulation, more than 10% ID/g was recovered in the ascitic fluid over 4–24 hours. The presence of intravenous-injected 188Re-liposomes in the peritoneal cavity may be associated with increased permeability of the tumor and peritoneal microvasculature. Previous studies with ascitic tumors demonstrate a steady extravasation process of liposomes into the ascitic fluid with gradual release of the drug followed by drug diffusion into the ascitic cellular compartment.Citation29,Citation30 This accumulation process has been shown to be related to the characteristics of the liposomes: small liposomes (with mean diameters of approximately 100 nm) achieve efficient accumulation in the peritoneal cavity in ascitic, tumor-bearing animals after intravenous injection.Citation29 The authors’ results suggest that the peritoneal cavity serves as an appropriate extravascular site for the passive targeting of 188Re-liposomes in peritoneal carcinomatosis. 188Re-liposomes intravenously administered not only rapidly target tumor tissues by EPR effect but also provide an extravasated pool of highly concentrated 188Re-liposomes surrounding the tumor nodules. The prolonged exposure of tumor to 188Re-liposomes by both mechanisms may account for the superior therapeutic effect observed in the experiments reported here.
In the authors’ previous studies, the biodistribution and pharmacokinetic properties of unencapsulated 188Re-BMEDA had been evaluated in a mouse model with C26 colon solid tumor and peritoneal carcinomatosis, respectively.Citation22,Citation27 The results showed no evidence for the accumulation of 188Re-BMEDA in tumors or in any normal organs. The fast blood clearance and excretion of 188Re-BMEDA were observed. Compared with the present study, 188Re-liposomes exhibited significant targeting and retention in the tumor. Pharmacokinetic study showed the slow clearance rate of 188Re-liposomes and the AUC was 4.7-fold greater than that of 188Re-BMEDA. These results suggested that pegylated nanolipsomes have higher bioavailability than 188Re-BMEDA, and the transportation of passive-targeted liposomes loaded with 188Re-BMEDA on tumor sites was achieved.
In vivo and noninvasive molecular imaging such as positron emission tomography (PET), single photon emission computed tomography (SPECT), magnetic resonance imaging, and optical imaging have gradually expanded to drug discovery and development in preclinical studies.Citation31–Citation33 In this study, in vivo direct visualization of tumor and ascites targeting and distribution of 188Re-liposomes were evaluated in peritoneal carcinomatosis mice by micro-SPECT/CT imaging, which provides further information for in vivo tumor and ascites retention (). The information from micro-SPECT/CT imaging is also supported by whole-body autoradiography and biodistribution data. An intraperitoneal ovarian cancer nude rat model was previously studied with in vivo microPET imaging, and the study found that ascites gathered from tumor-bearing rats had higher fludeoxyglucose uptake than the peritoneal fluid collected from control rats, which is similar to the results of the present study.Citation34
Optimized radionuclide therapy for cancer treatment is based on the concept of absorbed dose to the dose limiting normal organ or tissue, and either red marrow or the liver may be the dose-limiting critical organ for radionuclide pegylated nanoliposome therapy.Citation35 Macey and Meredith projected 188Re as the most advantageous of the β emitters for a favorable tumor/marrow ratio.Citation36 In the present authors’ dosimetry study, the absorbed doses to liver, red marrow, and total body with intravenous injection were 0.24, 0.04, and 0.11 mSv/MBq for 188Re-liposomes (), which were lower than those reported by Emfietzoglou et al (of 0.44, 0.12, and 0.15 mSv/MBq, respectively), for an 188Re-labeled small unilamellar vesicle liposome.Citation19 According to the present authors’ dosimetry results, red marrow was regarded as the primary critical organ for 188Re-liposomes. This low-absorbed dose of red marrow for 188Re-liposomes allows the maximized activity of 188Re-liposomes to be administrated for achieving the highest absorbed dose to tumors.
Safra et al report that tumor size is an important prognostic factor for therapeutic response to the liposomal drug doxorubicin in ovarian cancer, which suggests that the tumor volume is clinically relevant for liposome uptake.Citation37 The trend is that the liposome uptake is higher in smaller tumors,Citation38,Citation39 which is consistent with Harrington’s findings in animal tumor models.Citation40 The effect of tumor size on the tumor-absorbed dose of 188Re-liposomes was also calculated using OLINDA|EXM (). The results showed that the tumor-absorbed dose decreased almost ninefold for tumors between 1 and 10 g (588 mSv/MBq and 65.7 mSv/MBq, repectively) and 85-fold with tumor mass changing from 1 to 100 g (588 mSv/MBq and 6.91 mSv/MBq, repectively) for 188Re-liposomes. These results indicate that small tumors could receive a higher absorbed dose than larger tumors and reflect one of the advantages of 188Re-liposomes in treating peritoneal carcinomatosis with multiple small-volume tumor nodules. It is also suggested that 188Re-liposomes may have the potential to be applied in adjuvant treatment combined with cytoreductive surgery to eliminate residual tumor nodules.
Intraperitoneal inoculation of tumor cells in an animal model is a commonly used method to induce peritoneal carcinomatosis.Citation41 The tumor progressive patterns in the mouse model discussed here also mimic the pathological features seen in clinical practice, including preferential seeding to the greater omentum and the development of hemorrhagic ascites.Citation3 The authors’ treatments were administered at 7 days after tumor inoculation to evaluate the therapeutic efficacy in the early stages of peritoneal carcinomatosis. Significantly improved therapeutic efficacy of 188Re-liposomes in treating peritoneal carcinomatosis was revealed in the present study. It was shown that internal radiotherapy with 188Re-liposomes achieved the best curative effects, including extended lifespan (increase of 34.6%; ), reduced production of ascites (decrease of 83.3% at 14 days; ), and inhibited tumor growth (decrease of 63.4% at 14 days; ). These results are significantly better than those seen after treatment with 5-FU chemotherapy and in the vehicle group of normal saline.
It should be noted that significant inhibition of ascites formation was observed in the 188Re-liposome-treated group for the period of peritoneal metastatic progression (). The presentation of ascites in association with colonic carcinoma has been attributed to metastases. However, the large accumulation of ascites is also considered a prominent factor contributing to the large incidence of further spread of tumor implants in peritoneal cavity.Citation3 Therefore, the management of patients with ascites is important, not only for improving quality of life but also for restricting metastatic progression.Citation42 Moreover, 188Re-liposomes exhibited greater antitumor activity than 5-FU in all the metastatic sites collected, especially in the liver hilum and mesentery (). In 188Re-liposome-treated mice, it was found that most of the tumor nodules clustered in the greater omentum, rather than spreading to multiple implanted sites as in the 5-FU-treated group. These observations suggested that the 188Re-liposome treatment is more effective in inhibiting tumor growth and further dissemination in peritoneal carcinomatosis than 5-FU.
Conclusion
The present study demonstrates the advantages of intravenously administrated 188Re-liposomes in treating peritoneal carcinomatosis in a mouse model. The biodistribution, pharmacokinetics, and imaging studies of 188Re-liposomes in the peritoneal carcinomatosis model revealed good tumor and ascites targeting, bioavailability, and localization. The dosimetry study also provides safety information for 188Re-liposomes in further clinical applications. In a therapeutic study, 188Re-liposomes were found to achieve apparent curative ability, including inhibition of the tumor cell proliferation and further dissemination, and malignant ascites formation. The results presented here establish the potential of 188Re-liposomes for the treatment of peritoneal carcinomatosis.
Acknowledgment
The authors thank Dr TY Luo and CJ Liu for providing the rhenium-188.
Disclosure
The authors declare no conflicts of interest in relation to this paper.
References
- EisenbergBDecosseJJHarfordFMichalekJCarcinoma of the colon and rectum: the natural history reviewed in 1704 patientsCancer1982496113111347059938
- GallagherDJKemenyNMetastatic colorectal cancer: from improved survival to potential cureOncology2010783–423724820523084
- CarmignaniCPSugarbakerTABromleyCMSugarbakerPHIntraperitoneal cancer dissemination: mechanisms of the patterns of spreadCancer Metastasis Rev200322446547212884919
- PestieauSRSugarbakerPHTreatment of primary colon cancer with peritoneal carcinomatosis: comparison of concomitant vs delayed managementDis Colon Rectum2000431013411346 discussion 1347–134811052509
- SoodRAscites: Diagnosis and ManagementJIACM2000518189
- HegdeSRSunWLynchJPSystemic and targeted therapy for advanced colon cancerExpert Rev Gastroenterol Hepatol20082113514919072376
- WolpinBMMayerRJSystemic treatment of colorectal cancerGastroenterology200813451296131018471507
- Gomez PortillaACendoyaILopez de TejadaIPeritoneal carcinomatosis of colorectal origin. Current treatment. Review and updateRev Esp Enferm Dig2005971071673716351464
- Novotny-DiermayrVSangthongpitagKHuCYSB939, a novel potent and orally active histone deacetylase inhibitor with high tumor exposure and efficacy in mouse models of colorectal cancerMol Cancer Ther20109364265220197387
- Gil-AdIZolokovALomnitskiLEvaluation of the potential anti-cancer activity of the antidepressant sertraline in human colon cancer cell lines and in colorectal cancer-xenografted miceInt J Oncol200833227728618636148
- PetrosRADeSimoneJMStrategies in the design of nanoparticles for therapeutic applicationsNat Rev Drug Discov20109861562720616808
- AllenTMCullisPRDrug delivery systems: entering the mainstreamScience200430356651818182215031496
- TorchilinVPRecent advances with liposomes as pharmaceutical carriersNat Rev Drug Discov20054214516015688077
- NewmanMSColbernGTWorkingPKEngbersCAmanteaMAComparative pharmacokinetics, tissue distribution, and therapeutic effectiveness of cisplatin encapsulated in long-circulating, pegylated liposomes (SPI-077) in tumor-bearing miceCancer Chemother Pharmacol1999431179923534
- VaageJDonovanDWipffETherapy of a xenografted human colonic carcinoma using cisplatin or doxorubicin encapsulated in long-circulating pegylated stealth liposomesInt J Cancer19998011341379935243
- UldrickTSWhitbyDUpdate on KSHV epidemiology, Kaposi Sarcoma pathogenesis, and treatment of Kaposi SarcomaCancer Lett2011305215016221377267
- Gonzalez MartinASafety profile of trabectedin in combination with liposomal pegylated doxorrubicin in relapsed ovarian carcinoma: considerations for optimal managementInt J Gynecol Cancer20112110 Suppl 1S6821540668
- CobleighMAOther options in the treatment of advanced breast cancerSemin Oncol201138Suppl 2S111621600380
- EmfietzoglouDKostarelosKSgourosGAn analytic dosimetry study for the use of radionuclide-liposome conjugates in internal radiotherapyJ Nucl Med200142349950411337529
- TingGChangCHWangHECancer nanotargeted radiopharmaceuticals for tumor imaging and therapyAnticancer Res200929104107411819846958
- TsengYLHongRLTaoMHChangFHSterically stabilized anti-idiotype immunoliposomes improve the therapeutic efficacy of doxorubicin in a murine B-cell lymphoma modelInt J Cancer199980572373010048974
- ChangYJChangCHChangTJBiodistribution, pharmacokinetics and microSPECT/CT imaging of 188Re-BMEDA-liposome in a C26 murine colon carcinoma solid tumor animal modelAnticancer Res2007274B2217222517695506
- CaoSRustumYMSynergistic antitumor activity of irinotecan in combination with 5-fluorouracil in rats bearing advanced colorectal cancer: role of drug sequence and doseCancer Res200060143717372110919639
- MooreMHirteHWSiuLPhase I study to determine the safety and pharmacokinetics of the novel Raf kinase and VEGFR inhibitor BAY43-9006, administered for 28 days on/7 days off in patients with advanced, refractory solid tumorsAnn Oncol200516101688169416006586
- YonemuraYFujimuraTFushidaSHyperthermo-chemotherapy combined with cytoreductive surgery for the treatment of gastric cancer with peritoneal disseminationWorld J Surg1991154530535 discussion 535–5361891941
- JayneDMolecular biology of peritoneal carcinomatosisCancer Treat Res2007134213317633045
- ChenLCChangCHYuCYBiodistribution, pharmacokinetics and imaging of 188Re-BMEDA-labeled pegylated liposomes after intraperitoneal injection in a C26 colon carcinoma ascites mouse modelNucl Med Biol200734441542317499731
- ChenLCChangCHYuCYPharmacokinetics, micro-SPECT/CT imaging and therapeutic efficacy of 188Re-DXR-liposome in C26 colon carcinoma ascites mice modelNucl Med Biol200835888389319026950
- BallyMBMasinDNayarRCullisPRMayerLDTransfer of liposomal drug carriers from the blood to the peritoneal cavity of normal and ascitic tumor-bearing miceCancer Chemother Pharmacol19943421371468194164
- GabizonAASelective tumor localization and improved therapeutic index of anthracyclines encapsulated in long-circulating liposomesCancer Res19925248918961737351
- MassoudTFGambhirSSMolecular imaging in living subjects: seeing fundamental biological processes in a new lightGenes Dev200317554558012629038
- ChangCHWangHEWuSYComparative evaluation of FET and FDG for differentiating lung carcinoma from inflammation in miceAnticancer Res2006262A91792516619487
- ChangCHJanMLFanKHLongitudinal evaluation of tumor metastasis by an FDG-microPet/microCT dual-imaging modality in a lung carcinoma-bearing mouse modelAnticancer Res2006261A15916616475693
- ZavaletaCLPhillipsWTBradleyYCMcManusLMJerabekPAGoinsBACharacterization of an intraperitoneal ovarian cancer xenograft model in nude rats using noninvasive microPET imagingInt J Gynecol Cancer200717240741717362319
- FisherDRAssessments for high dose radionuclide therapy treatment planningRadiat Prot Dosimetry20031051–458158614527031
- MaceyDJMeredithRFA strategy to reduce red marrow dose for intraperitoneal radioimmunotherapyClin Cancer Res1999510 Suppl3044s3047s10541341
- SafraTGroshenSJeffersSTreatment of patients with ovarian carcinoma with pegylated liposomal doxorubicin: analysis of toxicities and predictors of outcomeCancer20019119010011148564
- HarringtonKJMohammadtaghiSUsterPSEffective targeting of solid tumors in patients with locally advanced cancers by radiolabeled pegylated liposomesClin Cancer Res20017224325411234875
- LimHJMasinDMcIntoshNLMaddenTDBallyMBRole of drug release and liposome-mediated drug delivery in governing the therapeutic activity of liposomal mitoxantrone used to treat human A431 and LS180 solid tumorsJ Pharmacol Exp Ther2000292133734510604968
- HarringtonKJRowlinson-BuszaGSyrigosKNInfluence of tumour size on uptake of 111ln-DTPA-labelled pegylated liposomes in a human tumour xenograft modelBr J Cancer200083568468810944612
- SugarbakerPHObservations concerning cancer spread within the peritoneal cavity and concepts supporting an ordered pathophysiologyCancer Treat Res199682791008849945
- AdamRAAdamYGMalignant ascites: past, present, and futureJ Am Coll Surg20041986999101115194082