Abstract
Purpose
Salmonellosis in poultry is a serious economic burden. A major concern is the public health hazard caused by consumption of Salmonella-contaminated poultry products. Currently used Salmonella vaccines are ineffective in combating poultry Salmonellosis warranting the need of a potent vaccine, especially an oral vaccine that can elicit robust local intestinal immunity.
Materials and Methods
A Salmonella subunit chitosan nanoparticles (NPs)-based vaccine was prepared that contained immunogenic outer membrane proteins (OMPs) and -flagellin (F) protein (OMPs-F-CS NPs). OMPs-F-CS NPs were administered as an oral vaccine in layer chickens and the resultant humoral and cell-mediated immune responses and localization of NPs were examined using standard detection methods.
Results
We demonstrated targeting of surface F-protein coated chitosan NPs to immune cells when delivered orally to layer chickens, the particles were localized in ileal Peyer’s patches. The OMPs-F-CS NPs vaccinated layer chickens had significantly higher OMPs-specific mucosal IgA production and lymphocyte proliferation response. The candidate vaccine increased the expression of toll-like receptor (TLR)-2, TLR-4, IFN-γ, TGF-ß and IL-4 mRNA expression in chicken cecal tonsils.
Conclusion
Our study demonstrated that the chitosan-based oral Salmonella nanovaccine targets immune cells of chickens and induced antigen-specific B and T cell responses. This candidate oral Salmonella nanovaccine has the potential to mitigate Salmonellosis in poultry.
Introduction
Salmonellosis is a zoonotic disease caused by the Gram-negative enteric bacterium Salmonella. Salmonella enterica serovar enteritidis is a major food-borne pathogen.Citation1 The World Health Organization has estimated that 1.3 billion cases of acute gastroenteritis and diarrhea and three million deaths are due to non-typhoidal Salmonellosis each year.Citation2 Approximately 40,000 cases of Salmonellosis and 2,000 deaths are reported in the United States each year, although the real number may be 30-fold greater.Citation3 Poultry and poultry-derived products are a major source of human Salmonella enteritidis (S. enteritidis) infections.Citation4 Aside from human health concerns, S. enteritidis causes severe economic losses to the poultry industry.Citation5 Thus, effective control of S. enteritidis infection in poultry is required to maintain healthy poultry flocks and prevent human Salmonellosis.Citation6
Vaccination is one of the approaches to control S. enteritidis infectionsCitation7 and both live attenuated and killed Salmonella vaccines are used to accomplish this goal.Citation8–Citation10 However, under field conditions, these vaccines only marginally decrease, rather than eliminate Salmonella colonization and shedding in the chicken intestine.Citation11–Citation13 Live Salmonella vaccines, though effective in inducing immunity, pose risk of reversion to virulence in vivo.Citation14 Killed whole bacterial vaccines provide only partial protection, due to poor immunogenicity and inability to induce cell-mediated immune responses.Citation15 Furthermore, all the available commercial killed Salmonella vaccines must be parenterally injected into each bird making it difficult for farmers and highly stressful to chickens. As an alternative, a potent killed or subunit oral Salmonella vaccine which induces robust mucosal IgA and cell-mediated immune responses is an effective control approach for Salmonellosis in poultry.
The outer membrane proteins (OMPs) of Salmonella are highly immunogenic in chickens.Citation16 When compared to killed bacterial extracts, partially purified OMPs induce better immune response against virulent Salmonella infection.Citation17 Enriched OMPs delivered with a potent adjuvant should elicit immune response and decrease Salmonella shedding in poultry.Citation18 Vaccines designed with Salmonella OMPs also activate professional antigen-presenting cells (APCs) thereby inducing adaptive immunity.Citation19 Salmonella sp. flagellin is a globular surface protein and virulence factor responsible for bacterial movement. Flagellin is a toll-like receptor (TLR)-5 agonist recognized by host innate immune cells. Salmonella attach to mucosal epithelial cells and colonize with the help of flagellin.Citation20 It has been suggested that the host TLR-flagella interactions are important for Salmonella to penetrate the gut epithelial barrier.Citation21
Oral vaccination is easy to deliver and a preferred route of vaccination in the poultry industry. It directly delivers vaccine antigens to gut-associated lymphoid tissues (GALT) and initiates mucosal IgA responses, something not possible by traditional parenteral route of vaccination.Citation22,Citation23 However, orally delivered unprotected vaccine protein antigens are degraded by acidic stomach pH and enteric proteolytic enzymes and frequently do not reach mucosal microfold (M) cells and APCs in the GALT.Citation23,Citation24 Therefore, a novel vaccine delivery and an adjuvant platform are essential to make the effective oral Salmonella vaccine in poultry.Citation22
Biodegradable polymeric nanoparticles (NPs)-based vaccine delivery systems have recently gained increased attention for use in food animals because of the nanoscale size, ideal physicochemical properties, increased surface area, biocompatibility, biodistribution, protection of the cargo antigen from gastric and enteric degradation and abilities to target vaccine to immune cells.Citation25–Citation28 Moreover, orally delivered polymeric nanoparticles vaccines are stable, mucoadhesive and bioavailable for prolonged period at the mucosal surfaces of the gastrointestinal (GI) tract.Citation22
Chitosan is a natural cationic copolymer derived from partial deacetylation of chitin, a component of crustacean and insect shells. Chitosan is composed of randomly distributed N-acetyl glucosamine and D-glucosamine residues with a net-positive charge.Citation29,Citation30 Chitosan is biocompatible and mucoadhesive and thus increases membrane permeability.Citation31 Chitosan has amino and carboxyl groups which form hydrogen bonds with mucus glycoproteins resulting in adhesion of chitosan to intestinal walls.Citation32 Moreover, chitosan interacts directly with intestinal epithelial cell membranes, leads to decrease in trans-epithelial electrical resistance and increased paracellular permeability.Citation33,Citation34 For these reasons, chitosan nanoparticles (CS NPs) have been extensively investigated for mucosal delivery of drugs, peptides and proteins.Citation35 CS NPs elicit robust immune response by activating macrophages and dendritic cells (DCs) and induce secretion of cytokines.Citation36 Moreover, NPs surface-coated with bacterial flagellin, improves mucoadhesive properties in the GI tract.Citation22 In this study, we tested the hypothesis that an orally delivered CS NPs vaccine containing entrapped S. enteritidis OMPs and flagellin (F) and surface coated with F-protein will efficiently reach chicken intestinal Peyer’s patches (PPs), and induce robust mucosal antibody and cell-mediated immune responses to S. enteritidis.
Materials and Methods
Isolation of OMPs
OMPs from S. enteritidis were isolated using Tris-sucrose-EDTA (TSE) buffer as described previouslyCitation37 with few modifications. Briefly, a stationary phase bacterial culture was washed with 10 mM Tris buffer pH 7.5 and the sediment was suspended in TSE buffer pH 8 and incubated on ice for 90 min. The cell suspension was centrifuged at 16,000 ×g for 30 min and the collected supernatant was centrifuged at 100,000 ×g for 60 min. The pellet containing OMPs enriched extract was freeze-dried with 5% sucrose as a cryoprotectant. The protein concentration was estimated using micro BCA protein assay kit (Thermo Scientific, MA) as per the manufacturer’s instructions.Citation38
Isolation of F-Protein
S. enteritidis bacterial culture grown on Trypticase soy agar plates was inoculated into brain heart infusion broth and incubated for 48 h at 37°C without shaking. The cells were washed with PBS pH 7.4 and centrifuged at 7000 ×g for 30 min. The cell pellet was treated with 3M potassium thiocyanate (Sigma, MO) in PBS for 2 h at room temperature under magnetic stirring. Subsequently, the cell suspension was centrifuged at 35,000 ×g for 30 min and the supernatant containing F-protein-enriched extract was dialyzed once against PBS pH 7.4 followed by Milli-Q water and freeze-dried with 5% sucrose as a cryoprotectant. The protein concentration was estimated using micro BCA protein assay kit.
Preparation of OMPs-F-CS NPs
The OMPs-F-CS NPs were prepared by an ionic gelation method as described previouslyCitation39 with some modifications. Briefly, 1.0% (w/v) low molecular weight chitosan (Sigma, MO) solution was prepared by slowly dissolving chitosan in an aqueous solution of 4.0% (v/v) acetic acid under magnetic stirring until the solution became transparent. The pH was adjusted to 4.3 and filtered through a 0.44 µm syringe filter. To prepare OMPs-F-CS NPs, 5 mL of 1% chitosan solution was added to 5 mL of deionized water and incubated with 2.5 mg each of both OMPs and F-protein in PBS pH 7.4. Subsequently, 2.5 mL of 1% (w/v) sodium tripolyphosphate (TPP) (Sigma, MO) in 2.5 mL deionized water was added into the solution and subjected to magnetic stirring, 22 °C. For surface coating, 2.5 mg of F-protein in PBS pH 7.4 was added to the particles and centrifuged at 10,500 ×g for 10 min to collect OMPs-F-CS NPs.
For the ex vivo and in vivo particle tracking study in chicken ileum, empty or surface F-protein coated CS NPs (CS NPs-F) were prepared as above without the vaccine carrier proteins, fluorescently labelled by incubation with 1.25 mg Rhodamine B isothiocyanate (RITC) (Sigma, MO) for 5 min as described previously.Citation22 The formulated NPs were separated by centrifugation at 10,500 ×g for 10 min and freeze-dried with 5% sucrose as a cryoprotectant.
Characterization of OMPs-F-CS NPs
The morphological characteristics of the empty and OMPs-F-CS NPs formulations were visualized under the cold field emission Hitachi S-4700 scanning electron microscopy (FE-SEM). The samples were prepared on aluminum stubs and coated with platinum prior to examination. Formulated empty and OMPs-F-CS NPs mean particle size distribution was analyzed by Zetasizer Nano ZS90 (Malvern Panalytical).
Encapsulation Efficiency
The protein encapsulation efficiency in CS NPs was estimated by an indirect method by determining difference between protein amount found in the vaccine formulation supernatant and initial amount used. The NPs surface-bonded protein was estimated similarly by subtracting the cargo loaded weight from the surface-coated protein. The amount of protein present in the supernatant was measured using the micro BCA protein assay kit.Citation38
Hemolysis Assay to Identify the NPs Toxicity
Fresh 2-mL chicken blood collected in EDTA in a sterile tube was centrifuged at 1000 ×g for 10 min and red blood cells (RBCs) were harvested. The RBCs were washed three times using sterile PBS and suspended in 3-mL PBS, 100-µL RBCs were treated with 250–1000 µg of CS NPs, or as a positive and negative control with Triton x-100 and PBS, respectively. Treated RBCs were incubated at 37°C for 1 h, centrifuged at 12,000 ×g for 10 min and the supernatant containing released hemoglobin was measured at OD 575 nm using the enzyme-linked immunosorbent assay (ELISA) plate reader (Spectramax plus 384, Molecular Devices, CA). The percentage (%) of hemolysis was calculated by the formula: [(sample absorbance − negative control)/(positive control − negative control)] × 100%.Citation40 Treated residual RBCs were suspended and morphological changes were observed under a microscope under 4x magnifications (Invitrogen™ EVOS™ FL Cell Imaging System, WA).
pH Stability Analysis
Stability of CS NPs was evaluated by measuring turbidity of NPs suspension kept at different acidic and alkaline pH conditions over a period of 3 hrs as described previously.Citation38 Briefly, 6 mg of CS NPs was suspended in 4 mL of different pH solutions (3.5, 4.0, 4.5, 5.5, 6.5 and 7.4) and at stipulated time intervals 100-µL aliquots were taken to measure turbidity at OD 405 nm using a spectrophotometer. All measurements were performed in duplicate and the results were expressed in percent reduction in turbidity at different pH conditions over a period of time. The percent reduction was calculated by: initial OD – different time point OD(s)/initial OD x100.
In vitro Splenocytes and Peripheral Blood Mononuclear Cell Uptake Study
On the necropsy day isolated uninfected control group peripheral blood mononuclear cells (PBMCs) and splenocytes were used to check-in vitro uptake of various NPs. PBMCs or splenocytes 5 million cells/well were plated in 24-well cell culture plate, incubated at 39°C in 5% CO2 for attachment. Unattached cells were removed and attached cells treated with 1-mL medium, RITC dye tagged 150 μg/mL CS NPs or CS NPs-F for 4 hr in a 39°C incubator. PBS was used to wash the cells twice followed by RPMI medium containing without phenol red was added. RITC red color dye tagged particle(s) interacting with cells were analyzed by fluorescence microscopy (Olympus IX70) and images were captured at 20× magnification.
Isolated PBMCs (1x106 cells/well) from uninfected control chickens were seeded into a 96-well cell culture plate and incubated at 39°C, 5% CO2 for 12 hrs to identify flagellin dependent CS NPs cell uptake. Attached cells were treated with either medium or 10 μg F-protein or same concentration of F-protein coated CS NPs (CS NPs-F) for 4 h. Incubated cells were fixed with 80% acetone, stained with primary rabbit anti-flagellin antibody (Abcam, MA) followed by secondary Alexa Fluor 488 conjugated goat anti-rabbit IgG antibody (Life Technologies, OR). The cells were washed with PBS and adherent stained cells were examined by fluorescent microscopy (Olympus IX70) and photographed as above.
Ex vivo and in vivo Bioadhesion Study
The GI tract mucosal adhesive properties of RITC-labelled CS NPs-F were analyzed in chicken ileal tissues by fluorescence microscopy.Citation41 For in vivo analyses, healthy layer chickens were orally treated with PBS, 0.6 mg of RITC dye or the same amount of RITC dye-labelled CS NPs or CS NPs-F for 4 hr. At necropsy, approximately 1-cm ileum was removed, incubated in 20% (w/v) sucrose solution for 4 hr and washed thoroughly with PBS. The ileum tissue was imbedded in Tissue-Tek O.C.T. compound (Sakura Finetek, CA) and frozen at −80°C. Five-micrometer sections were prepared using the cryostat (Leica CM1510S, IL). Tissue sections were mounted on poly-L-lysine-precoated glass microslides, stained with 4ʹ,6-Diamidino-2-Phenylindole, Dihydrochloride (DAPI) and visualized under a cell imaging microscope (Invitrogen™ EVOS™ FL Cell Imaging System, WA) at 2x objective. For ex vivo analyses, 10 cm long ileal tissue from healthy chickens was harvested, washed thoroughly with PBS to remove intestinal contents and treated with PBS, 0.6 mg of RITC dye or same amount of RITC-labelled CS NPs-F for 4 hr in PBS at 37°C, washed several times using PBS and processed for imaging as described above.
Experimental Design, Vaccination Schedule, Bacterial Challenge and Interim and Final Sample Collections
Chickens
Specific pathogen-free 1-day-old layer chicks from Mayer Hatchery (OH, USA) were received at the OARDC animal house facility for use in this vaccination and challenge study. All chicks had ad libitum access to water and antibiotic-free food. At 6 weeks of age, chickens were randomly divided into four experimental groups in cages in separate isolation rooms ().
Table 1 Experimental Design Showing Assignment of Layer Chicken Groups
Vaccination(S)
Chickens at the age of 6 weeks were orally vaccinated using an oral gavage needle with soluble OMPs (50 µg) and F-protein (50 µg) in sterile PBS or equivalent amount of respective protein-loaded OMPs-F-CS NPs suspension in 1-mL sterile PBS. The vaccination procedure was repeated twice more at 3-week intervals. Booster vaccination was performed twice using similar dose and route.
Challenge Salmonella Inoculum
The nalidixic acid-resistant pure culture of virulent S. enteritidis (Phage type 13a) stored in glycerol stock was grown in 10-mL tryptic soy broth (TSB) at 37°C without shaking. After 8-h incubation, 100 µL of the bacterial suspension was transferred into 10-mL fresh TSB and incubated overnight at 37°C. One-milliliter bacterial suspension was transferred into 100-mL fresh TSB and incubated at 37°C until the culture OD reached 1.1. Bacteria were washed three times with PBS, serially diluted and plated on Xylose Lactose Tergitol™ 4 (XLT4) agar plate and colony-forming units (CFUs) were determined. Birds were challenged on day post-vaccination (DPV) 63 with 1-mL bacterial suspension containing 1x109 CFU in PBS after overnight fasting using an oral gavage needle. Chickens were euthanized 10 days after bacterial challenge. Birds were maintained, sample collection and euthanasia procedures were strictly followed as per the Standards of the Institutional Laboratory Animal Care and Use Committee and Ethics for Animal Experiments. The present study and all experimental procedures were approved by the Institutional Animal Care and Use Committee at The Ohio State University protocol number: 2016A00000060. Animal experiments were performed as per the recommendations by the Public Health Service Policy, USDA Regulations, National Research Council’s Guide for the Care and Use of Laboratory Animals and the Federation of Animal Science Societies’ Guide for the Care and Use of Agricultural Animals in Agricultural Research and Teaching.
Interim Microbial Sampling
The cloacal swabs were collected on day post vaccination (DPV) 21, 42, 63 and 73 (DPC 10) in 0.5-mL PBS. Cloacal swabs were vortexed, centrifuged at 3000 ×g for 10 min and aliquots were stored at −80°C.
Tissue Sampling at Termination
On the day of necropsy, 10 days after challenge, blood samples for serum were collected from each chicken. The 5-cm small intestine and trachea samples were collected in 2-mL PBS, cut into small pieces, vortexed and centrifuged at 3000 ×g for 10 min; aliquots of supernatants were stored at −80°C. Bile samples were collected with an insulin syringe and aliquots were stored at −80°C. Terminal unclotted blood was collected in sterile EDTA tubes for isolation of PBMCs. Spleen was collected in 5-mL RPMI medium (GE Healthcare Life Sciences, UT) enriched with 10% fetal bovine serum (Sigma), antibiotic-antimycotic (Gibco), sodium pyruvate, 1M HEPES, MEM NEAA and 2- mercaptoethanol (E-RPMI).
Analyses of Antibody Responses
The levels of pre and post-challenge IgA antibodies to Salmonella sp antigens in serum, bile, cloacal swabs, small intestinal and tracheal washes were determined by ELISA. Flat bottom 96-well plates (Greiner Bio-one, NC) were coated with OMPs (7.5 µg/mL) in 0.05 M carbonate-bicarbonate buffer pH 9.6 and incubated 12 hr, 4°C. Plates were washed three times and blocked with 5% (w/v) skim milk powder in PBS Tween-20 (0.05%) (PBST) for 1 hr, 22°C. Plates were washed three times in PBST and pre-diluted serum or bile samples in 2.5% (w/v) skim milk powder or undiluted cloacal swabs, tracheal and small intestinal wash samples were added in duplicate marked wells (50 µL/well) and incubated for 2 hr, 22°C. Plates were washed three times and 50 µL/well of pre-titrated goat anti-chicken IgA conjugated HRP (Gallus Immunotech, NC) (1: 3000 in 2.5% skim milk powder in PBST) secondary antibody was added and incubated for 2 hr, 22°C. Plates were washed three times and 50 µL/well of TMB peroxidase substrate (1:1 mixture of TMB peroxidase substrate and peroxidase substrate solution B) (KPL, MD) was added and the reaction was stopped after color development in 10 to 20 min by adding 1.0 M phosphoric acid. The OD values were measured at 450 nm using the ELISA plate reader. The corrected OD value was obtained by subtracting different treatment group OD from blank control OD. Interferon-gamma (IFN-γ) levels in serum samples collected at DPC 10 were determined using a commercial kit (Cytoset™ Chicken IFN-γ ELISA based kit, Invitrogen, USA).
Lymphocyte Proliferation Assay
PBMCs and splenocytes were isolated using Ficoll-paque plus (GE Healthcare, PA). Briefly, blood was diluted in PBS (1:1 ratio) and an equal volume of Ficoll-paque plus solution was added and the mixture was then centrifuged at 450xg for 25 min, 20°C. Lymphocytes at the interface were collected, washed twice in PBS and suspended in E-RPMI medium. Splenocytes were isolated by teasing splenic tissue through a cell strainer using PBS. Splenocytes were then mixed with an equal volume of Ficoll-paque plus solution and centrifuged at 450 xg for 30 min, 4°C. Splenocytes at the interface were collected, washed twice with PBS and resuspended in E-RPMI medium.
For the cell proliferation assay, PBMCs and splenocytes (1x106 cells/well in 100 µL) were suspended in E-RPMI medium and seeded in triplicate wells of 96 well flat-bottom plates (Greiner bio-one, NC). Cells were treated with OMPs (5 µg/mL in 100 µL) in E-RPMI medium and incubated for 72 h at 39°C, 5% CO2. After incubation, 20 µL of MTS+PMS solution was added and incubated for 4 h at 37°C, 5% CO2. The OD value was taken at 490 nm by the ELISA plate reader. The stimulation index (SI) was calculated by dividing OD value of stimulated cells from OD value of unstimulated control cells of the same chicken. The average SI value of 9 to 10 chickens of each group was compared among vaccine groups.
Quantitative Reverse Transcriptase-PCR (qRT-PCR) Analysis
Total RNA from the cecal tonsils was extracted by using the TRIzol reagent (Invitrogen). The isolated RNA was dissolved in Tris-EDTA (pH 7.5) buffer and concentration was determined by using NanoDrop™ 2000c Spectrophotometer (Thermo Fisher Scientific). The cDNA synthesis was achieved with 1 µg of total RNA using the QuantiTect Reverse Transcription Kit (QIAGEN) according to the manufacturer’s instructions. The mRNA expression of TLR-2, TLR-4, IFN-γ, TGF-ß, IL-4 and the house keeping gene β-actin (Table S1) were analyzed by real-time quantification (7500 Real-Time PCR System, Applied Biosystems, CA) using the iQ™ SYBR® Green Supermix (Bio-Rad, CA). Target gene expression levels were normalized to β-actin and the fold changes were determined by dividing results of the treated samples by the respective untreated control samples.
Statistical Analyses
Data are represented as the mean ± standard error of mean (SEM) of 9 or 10 chickens. Data were analyzed by non-parametric Kruskal–Wallis test, followed by p value difference between the groups were determined by Mann–Whitney testCitation42 using the Graphpad Prism 5 (Graphpad software, CA). A p value less than 0.05 was considered statistically significant.
Results
Characterization of Candidate Nanoparticle Vaccine
As shown in the schematic , protonated cationic chitosan amine groups at pH 4.3 formed electrostatic complex with negative-charged acidic groups of OMPs and F-protein in PBS pH 7.4. In addition, negatively charged cross-linker TPP was used to entrap vaccine antigens in CS NPs. Surface attachment of F-protein on CS NPs was accomplished by electrostatic interaction. By FE-SEM, the CS NPs and OMPs-F-CS NPs were spherical in shape and distributed in the colloidal matrix with minimal aggregation (). The mean particle size distribution of CS NPs and OMPs-F-CS NPs were 380 nm and 517 nm, respectively (). The encapsulation efficiency of CS NPs for Salmonella antigens was 70% and the efficiency of surface conjugation of F-protein was 40%.
Figure 2 Physicochemical characterization of CS NPs and OMPs-F-CS NPs. FE-SEM analysis of (A) CS NPs and (B) OMPs-F-CS NPs. Mean particle size distribution of (C) CS NPs and (D) OMPs-F-CS NPs.
Abbreviations: OMPs, outer membrane proteins; F, flagellin; CS NPs, chitosan nanoparticles; OMPs-F-CS NPs, OMPs and F-protein entrapped and surface F-protein coated CS NPs; FE-SEM, field emission-scanning electron microscope.
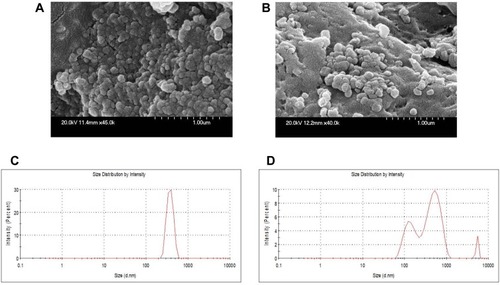
Hemolysis assay is one of the well-accepted methods of biocompatibility analysis for NPs.Citation40 Biocompatibility of CS NPs was analyzed by quantifying the hemolysis of chicken RBCs treated with NPs. There was absence of any hemolysis upon treatment with CS NPs and comparable to PBS control (Figure S1A), while RBCs treated with Triton X-100 were completely lysed (Figure S1B).
NPs form turbidity when dispersed in biological buffer. Turbidity reduction is an indirect way of identifying stability of NPs in various physiological conditions over a period of time.Citation38 Stability of CS NPs under different acidic and alkaline pH conditions was assessed by the turbidity reduction assay. At acidic pH 3.5 after 3 h incubation, CS NPs induced less than 10% turbidity reduction. Increasing the time of incubation from 1 to 3 h, slightly increased the reduction in turbidity at pH 3.5 to 6.5, but the data were not significant. At pH 7.4 the turbidity values were not different at any time point, indicating that CS NPs were stable even after 3 h of incubation at pH 7.4 (Figure S1C).
Uptake of CS NPs-F by Splenocytes and PBMCs in vitro
APCs in splenocytes and PBMCs were used to analyze the uptake of CS NPs-F. Cells treated with RITC fluorescent dye tagged both CS NPs and CS NPs-F showed red fluorescence compared to medium control in a fluorescence microscopy (). The CS NPs-F treated both splenocytes and PBMCs had much higher fluorescence signal and also in more cells compared to CS NPs treatment (). Further to confirm the vaccine antigens uptake by APCs, similarly treated PBMCs with F-protein or CS NPs-F were immunostained for flagellin. A high specific green fluorescence signal was observed in CS NPs-F treated cells compared to soluble F-protein ().
Figure 3 In vitro uptake analysis of CS NPs-F by splenocytes and PBMCs. (A) Splenocytes and (B) PBMCs were treated with either medium, RITC dye tagged CS NPs or RITC dye tagged CS NPs-F for 4 h and observed in the red channel under a fluorescent microscope (20× magnification). (C) PBMCs were treated with either medium, soluble F-protein or CS NPs-F for 4 h, immunostained with flagellin specific antibody and cells were examined in green channel under a fluorescent microscope (20× magnification).
Abbreviations: PBMCs, peripheral blood mononuclear cells; RITC, rhodamine B isothiocyanate; F, flagellin; CS NPs, chitosan nanoparticles; CS NPs-F, surface F-protein coated CS NPs.
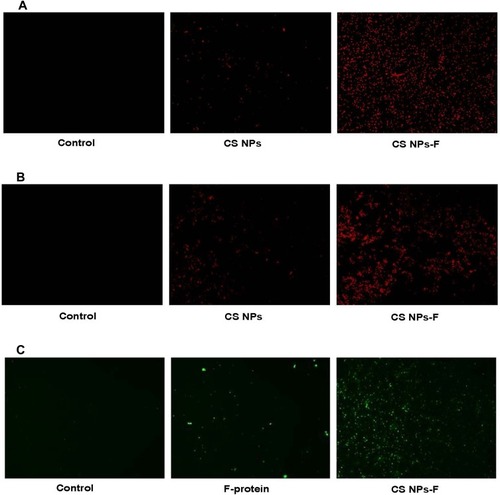
Analysis of Bioadhesion of CS NPs-F by in vivo and ex vivo Analyses
The mucoadhesive nature of CS NPs-F labeled with a fluorescent dye was analyzed in the ileum by fluorescence microscopy. CS NPs-F adhered to the mucosa and entered in the lamina propria and PPs sites of the ileum ( and Figure S2). Only a few CS NPs without the surface-coated F-protein adhered to the mucosa and the epithelial cells (). In an ex vivo experiment, control fluorescent dye RITC-treated ileum had the dye adhered to mucosal epithelial surfaces but did not penetrate the lamina propria whereas CS-NPs-F was taken into the lamina propria (Figure S2).
Figure 4 In vivo analysis of penetration of CS NPs-F in the ileum mucosa of chickens. Layer chickens were orally treated with PBS, RITC dye or RITC-labelled CS NPs or CS NPs-F. Birds were euthanized after 4 h and ileum was harvested, washed, fixed, sectioned, stained with DAPI and visualized under a fluorescent microscope. The pictures were taken using the 2x objective (scale bar: 2000 µm). Indicated arrow marks are: 1 – Lamina propria; 2 – Peyer’s patches site.
Abbreviations: PBS, phosphate-buffered saline; RITC, rhodamine B isothiocyanate; DAPI, 4′,6-diamidino-2-phenylindole; F, flagellin; CS NPs, chitosan nanoparticles; CS NPs-F, surface F-protein coated CS NPs.
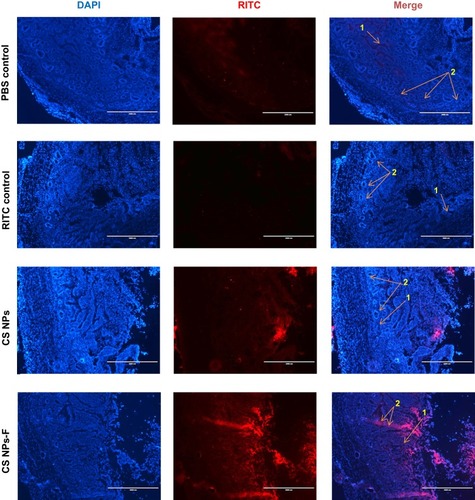
OMPs-F-CS NPs Induced OMPs-Specific Humoral Immune Response
OMPs-specific IgA antibody response was analyzed in serum, cloacal swabs, bile and small intestine and tracheal wash samples. OMPs-F-CS NPs vaccinates had increased OMPs-specific IgA antibody levels in cloacal swabs after the first, second and third inoculation compared to the unvaccinated control group (). Specifically, after the second booster a significantly (P < 0.05) increased IgA response was observed when values were compared to both soluble antigens and control groups ().
Figure 5 Pre-challenge OMPs-specific IgA antibody response in chickens vaccinated orally with OMPs-F-CS NPs. Layer chickens were inoculated orally three times with mock PBS (group 1), OMPs and F-protein in soluble form (group 2) or entrapped in nanoparticle (OMPs-F-CS NPs) (group 3). OMPs-specific IgA antibody response in cloacal swab (A-C) was analyzed by ELISA. Each bar is the mean ± SEM of 9 to 10 chickens, and the data were analyzed by non-parametric Kruskal–Wallis test followed by p value differences in between the groups were determined by Mann–Whitney test. Asterisk refers to statistical difference between the two indicated groups (*P < 0.05 and **P < 0.01).
Abbreviations: PBS, phosphate-buffered saline; OMPs, outer membrane proteins; F, flagellin; CS NPs, chitosan nanoparticles; OMPs-F-CS NPs, OMPs and F-protein entrapped and surface F-protein coated CS NPs; ELISA, enzyme-linked immunosorbent assay; SEM, standard error of the mean.
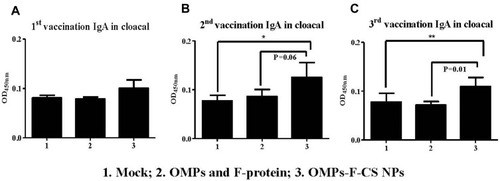
At DPC 10, birds in all the treatment groups had significantly higher (P < 0.05) OMPs-specific IgA antibody response compared to unvaccinated Salmonella-challenged and unchallenged control groups (). Specifically, in OMPs-F-CS NPs-vaccinated chickens, IgA response in serum, bile, cloacal swab, small intestinal and tracheal wash samples were higher when compared to soluble antigens-vaccinated group (). An increase in OMP-specific IgA level was significantly (P < 0.05) greater only in the small intestinal washes of OMPs-F-CS NPs vaccinates compared to both control and soluble antigens vaccinated groups ().
Figure 6 Post-challenge OMPs-specific IgA antibody response in chickens vaccinated orally with OMPs-F-CS NPs. Layer chickens vaccinated orally three times with mock PBS (group 1 & 2), OMPs and F-protein in soluble form (group 3) or entrapped in nanoparticle (OMPs-F-CS NPs) (group 4). Groups 2 to 4 were challenged (Ch) orally with live S. enteritidis, euthanized at DPC 10 and analyzed for: (A) IgA in serum; (B) IgA in bile; (C) IgA in cloacal swabs; (D) IgA in small intestinal wash; and (E) IgA in tracheal wash. Each bar is the mean ± SEM of 9 to 10 chickens, and the data were analyzed by non-parametric Kruskal–Wallis test followed by p value differences in between the groups were determined by Mann–Whitney test. Asterisk refers to statistical difference between two indicated groups (*P < 0.05, **P < 0.01 and ***P < 0.001).
Abbreviations: PBS, phosphate-buffered saline; OMPs, outer membrane proteins; F, flagellin; CS NPs, chitosan nanoparticles; OMPs-F-CS NPs, OMPs and F-protein entrapped and surface F-protein coated CS NPs; ELISA, enzyme-linked immunosorbent assay; DPC, day post-challenge; SEM, standard error of the mean; Ch, challenge.
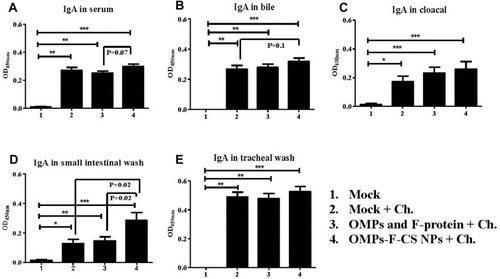
OMPs-F-CS NPs Enhanced OMPs-Specific Cell-Mediated Immune Response
The cell-mediated immune response induced by OMPs-F-CS NPs vaccine was measured by analyzing IFN-γ levels in serum and OMPs-specific lymphocyte proliferation in PBMCs and splenocytes. OMPs-F-CS NPs vaccinates had higher but not significantly increased IFN-γ levels in serum compared to soluble antigens vaccinates (). In PBMCs of OMPs-F-CS NPs vaccinated chickens an increase in lymphocyte stimulation index values were not statistically significant compared to other treatment groups (). However, splenocytes from OMPs-F-CS NPs vaccinates had significantly higher stimulation index values (P < 0.05) compared to control and soluble antigens groups ().
Figure 7 Post-challenge cell-mediated response in chickens vaccinated orally with OMPs-F-CS NPs. Layer chickens were vaccinated, challenged with live S. enteritidis and euthanized at DPC 10 as described in the figure legend 6. (A) Serum was estimated for IFN-γ production by ELISA. On the day of necropsy PBMCs and splenocytes were isolated, restimulated with OMPs to measure antigen-specific cell proliferation stimulation index in (B) PBMCs and (C) Splenocytes by a calorimetric assay. The stimulation index value was calculated by the mean OD of OMPs stimulated proliferation/mean OD of non-stimulated proliferation. Each bar is the mean ± SEM of 9 to 10 chickens, and the data were analyzed by non-parametric Kruskal–Wallis test followed by p value differences in between the groups were determined by Mann–Whitney test.
Abbreviations: OMPs, outer membrane proteins; F, flagellin; CS NPs, chitosan nanoparticles; OMPs-F-CS NPs, OMPs and F-protein entrapped and surface F-protein coated CS NPs; ELISA, enzyme-linked immunosorbent assay; IFN-γ, interferon gamma; PBMCs, peripheral blood mononuclear cells; DPC, day post challenge; SEM, standard error of the mean; Ch, challenge.
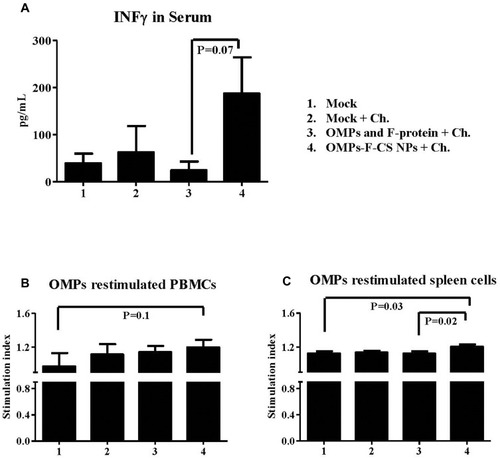
OMPs-F-CS NPs Increased TLRs, Th1 and Th2 Cytokines Gene Expression
The ability of OMPs-F-CS NPs to induce the expression of important immune response-related genes in vaccinated chickens was analyzed by quantitating TLR-2 and TLR-4, and cytokines Th1 (IFN-γ), immunoregulatory (TGF-ß) and Th2 (IL-4) mRNA levels in the cecal tonsils. We observed an increased TLR-2 mRNA in OMPs-F-CS NPs vaccinated birds, whereas control-challenged and soluble antigens-vaccinated birds had relatively downregulated mRNA levels (). OMPs-F-CS NPs vaccinated birds had an increased, but not statistically significant TLR-4 mRNA levels compared to other treatment groups (). Interferon-gamma mRNA expression levels in OMPs-F-CS NPs vaccinates were significantly (P < 0.05) higher when compared to soluble antigens group (). TGF-β expression was comparable in all the experimental groups (). OMPs-F-CS NPs vaccination had significantly (P < 0.05) increased the expression of IL-4 mRNAs compared to mock-challenge birds, while it was downregulated in soluble antigens group ().
Figure 8 TLRs and cytokines mRNA expression profiles in the cecal tonsils of chickens. Layer chickens were vaccinated, challenged with live S. enteritidis and euthanized at DPC 10 as described in the figure legend 6. The gene expression levels in the cecal tonsils- was analyzed by quantitative RT-PCR. The relative mRNA expression levels of (A) TLR-2; (B) TLR-4; (C) IFN-γ; (D) TGF-ß; and (E) IL-4 were normalized to the expression of ß-actin. Each bar is the mean ± SEM of 9 to 10 chickens, and the data were analyzed by non-parametric Kruskal–Wallis test followed by p value differences in between the groups were determined by Mann–Whitney test.
Abbreviations: OMPs, outer membrane proteins; F, flagellin; OMPs, outer membrane proteins; F, flagellin; CS NPs, chitosan nanoparticles; OMPs-F-CS NPs, OMPs and F-protein entrapped and surface F-protein coated CS NPs; RT-PCR, reverse transcription polymerase chain reaction; TLRs, toll-like receptors; IFN-γ, interferon gamma; TGF-ß, transforming growth factor beta; IL, interleukin; SEM, standard error of the mean; Ch, challenge.
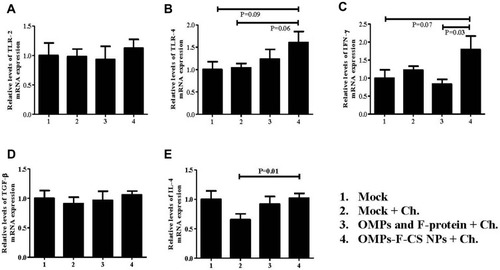
Discussion
Virulent Salmonella spp. enters the human food chain primarily as a poultry contaminant. In the avian GI tract, M cells associated with the PPs sample and process the foreign antigens and trigger specific immune responses.Citation43 The goal of oral vaccination is to target antigens to mucosal M cells and activate immune cells in PPs and local draining lymph nodes, thereby mimicking the natural infection process.Citation44 A chitosan particle-based vaccine containing immunogenic Salmonella antigens (OMPs and F-protein) and surface coated with F-protein was formulated to target M cells in chicken ileal PPs. The extracted antigens (OMPs and F-protein) analyzed by SDS-PAGE showed expected proteins corresponding to molecular weight were reported earlier.Citation45
Studies have established that the size and shape of NPs determine their biological properties.Citation46 The formulated CS NPs and OMPs-F-CS NPs were roughly 500-nm, the particle size of 500 nm or more are likely taken up via clathrin-independent macropinocytosis and/or phagocytosis.Citation47 In vitro studies established that spherical NPs are internalized by DCs and deliver loaded antigens.Citation48 We determined that encapsulating the vaccine proteins in CS NPs increased their size and altered surface conformation as reported previously.Citation49 The protein encapsulation efficiency of CS NPs is mainly dependent on charge of the immunogenic protein. Every protein has an isoelectric point (pI), a pH at which it carries zero net charge. Increasing the pH above the pI value confers a net negative protein charge.Citation50 In this study, Salmonella antigenic OMPs (pI 4.2–5.8)Citation51 and F-protein (pI 5.3)Citation52 were dissolved in PBS pH 7.4 to make them negatively charged. Cationic chitosan polymer (pI 6.5) is highly protonated in lower acidic environments and forms electrostatic complexes with the negative charged protein antigens.Citation50 As in previous reports,Citation50,Citation53 the formulated OMPs-F-CS NPs had high protein encapsulation efficiency.
The formulated CS NPs were biocompatible with chicken RBCs as shown by the hemolysis assay. The TPP crosslinked CS NPs are biocompatible in chicken embryos.Citation54 The CS NPs remained stable in acidic pH conditions. Stable NPs protect entrapped vaccine proteins from enzymatic degradation at acidic pH in the GI tract.Citation55 Chitosan particles are stable in acidic (pH 2) environment, protect antigens in the GI tract and serve as a suitable carrier for oral vaccine delivery.Citation56 Since the CS NPs are stable, they protect attached proteins cargo during the freeze-drying process, reduces aggregation and keeps the particles dispersed in suspension.Citation54
Flagellin directly interacts with myeloid DCs through TLR-5 and activates other TLR-5- expressing cells by the bystander process and indirectly activate spleen DCs.Citation57,Citation58 A number of different immune cells including monocytes, macrophages, DCs and lymphocytes possess the TLR-5 receptor.Citation58,Citation59 The TLR-5 receptor is also expressed on epithelial cells and, for this reason, flagellin is considered as a potent mucosal adjuvant.Citation58,Citation60 Surface coating of NPs with specific ligand(s) enhances targeted delivery of antigens to gut receptors. Salmonella adheres to the epithelial mucosa and enter M cells of the follicle associated epithelium (PPs) in the small intestine. S. enteritidis flagellin has been previously shown to adhere M cells in PPs.Citation22 When compared to the behavior of uncoated NPs, flagellin-coated NPs are readily uptaken by ileal PPs M cells by passing through mucus layer.Citation41,Citation61 Polyanhydride NPs surface-coated with flagellin mimic natural Salmonella colonization in the rat GI tract.Citation41 In an in vitro study using an M cell line, Ovalbumin (OVA)-loaded CS NPs are successfully adsorbed and processed by M cells.Citation62 In this study, CS NPs-F showed specific fluorescence signal in treated PBMCs and splenocytes. The ileum of chickens orally inoculated with CS NPs-F demonstrated uniform distribution in the lamina propria and PPs consistent with a previous study in rats.Citation41 This data indicate that the formulated CS NPs-F were adsorbed by chicken immune cells, attached to the intestinal epithelial mucosa and passed through epithelial lining before found in the lamina propria and PPs. Positively charged amino groups of CS NPs interact with negatively charged sialic acid residues in the mucousal epithelial layer. This permits temporary relaxation of tight junction proteins thereby facilitating transport of NPs across the epithelial barrier.Citation63
Protection against Salmonella colonization and shedding is a complex process involving efficient communication between innate and adaptive immune cells.Citation64 In the experiments reported here, birds vaccinated and challenged with a high dose of virulent Salmonella (1x103 CFUs more than the infection dose),Citation65 was designed to determine the mechanism(s) of vaccine efficacy in terms of boosting the Salmonella-specific memory T and B cell responses. As expected, OMPs-F-CS NPs induced higher levels of mucosal IgA response in birds compared to soluble antigens vaccination. Importantly, the levels of mucosal IgA responses were greater than CS NPs vaccine delivery system used in other studies.Citation16,Citation18 The OMPs contain the major immunodominant proteins of Salmonella and an adjuvant-based OMPs delivered parenterally in poultry significantly increases antibody responses to Salmonella.Citation18 Around 500 nm size nanovaccine formulation promotes the humoral immune response.Citation47 The β-galactosidase-loaded CS NPs significantly improve the antigen-specific serum antibody titers and splenocyte CD4+ T cell proliferation in mice.Citation66 Serum antibody titers in mice vaccinated with OVA are significantly increased by CS NPs.Citation67 CS NPs improved the antigen-specific mucosal IgA response in rabbits vaccinated orally.Citation68 Salmonella is both an intracellular and extracellular pathogen and induction of both intestinal IgA and T cell responses are critical for bacterial clearance.Citation69
Our experiments demonstrate that the engineered OMPs-F-CS NPs induces cell-mediated immune response in chickens. Antigen-specific lymphocyte proliferation assay measures cell-mediated immune response against Salmonella in poultryCitation70 and mice.Citation71 OmpA-vaccinated murine splenocytes co-cultured with APCs enhanced T-cell proliferation, Th1 polarization and IFN-γ production.Citation72 Nanosized spherical particles enhance strong Th1 and Th2 immune responses.Citation48 Vaccine antigens encapsulated in CS NPs induce significantly higher lymphocyte proliferation in chickens.Citation39,Citation73 Mice vaccinated with CS NPs containing entrapped OVA significantly increased OVA-induced proliferation of splenocytes.Citation67 Chitosan stimulates maturation of DCs by triggering type I-IFNs and resultant increased T cell responses.Citation74 The TTP-crosslinked CS NPs increase the expression of maturation markers in treated DCs such as MHC-II and costimulatory molecules CD40 and CD86.Citation75 Antigen-loaded CS NPs also enhances the expression of maturation markers (CD40, CD80, CD86 and MHC-II) in vitro in murine DCs.Citation76 Antigen-loaded CS NPs also increases antigen-specific humoral and cell-mediated immune responses in mice.Citation66
The TLRs agonists are potent vaccine adjuvants that act by triggering the production of Th1 and Th2 cytokines, maturation of DCs and inducing antibody and cell-mediated immune responses.Citation22 In this study, OMPs-F-CS NPs vaccination upregulated TLR-4 mRNA expression, indicating the possible pathway of adaptive immune activation. CS NPs activate DCs and upregulate CD80 and CD86 expression through TLR-4 dependent pathway.Citation77 The hydrophobic portion of water-insoluble polymers derived CS NPs is recognized by TLRs on APCs.Citation22 OMPs-F-CS NPs vaccinates had significantly upregulated IFN-γ (Th1 cytokine) mRNA expression with higher antigen-specific lymphocyte proliferation in the spleen. Moreover, in our study an increased expression of Th2 cytokines (IL-4 and TGF-ß) gene expressions were associated with enhanced antibody titers in the intestine. However, soluble proteins reduced IL-4 gene expression. In mice, OVA-loaded CS NPs vaccination upregulated the levels of Th1 (IL-2 and IFN-γ) and Th2 (IL-10) cytokines mRNA expression.Citation67 Chitosan enhances pinocytosis and IFN-γ mRNA expression in macrophage-origin RAW264.7 cells in vitro.Citation78
In summary, an engineered, biocompatible, biologically stable, mucoadhesive and targeted oral Salmonella subunit candidate vaccine (OMPs-F-CS NPs) was developed for use in poultry. Our study also demonstrated the ability of OMPs-F-CS NPs to activate the intestinal mucosal immune system. Investigations are in progress to identify the ability of OMP-F-CS NPs to reduce Salmonella colonization and shedding in vaccinates following dose-dependent challenge infection.
Conclusions
We designed and demonstrated the immune responses to oral CS NPs -based Salmonella subunit vaccine for use in poultry. The candidate vaccine targeted immune cells in the gut elicited Salmonella-specific mucosal IgA, T cell responses and induced the expression of Th1 and Th2 cytokines mRNA expression. This provides evidence that a chitosan-based nanoparticle system is suitable for oral delivery of subunit vaccine antigens to mitigate Salmonellosis and Salmonella shedding in poultry.
Acknowledgment
We thank our animal care unit staff Mr. Patterson Keith and Sidle Jack who provided help in animal studies. We thank Dr. Tea Meulia and Horst Leona and her team at MCIC, OARDC for their help in FE-SEM analysis. Thanks to Sujata Senapati and Dr. Balaji Narasimhan, Iowa State University for their help with particle size analyses. Sincere thanks to Dr. Steven Krakowka for editing the manuscript. This work was partially supported by USDA-NIFA grant and OSU Accelerator award. Salaries and research support were provided by state and federal funds appropriated to OARDC, The Ohio State University.
Disclosure
The authors report no conflicts of interest in this work.
References
- Khan MI, Fadl AA, Venkitanarayanan KS. Reducing colonization of Salmonella Enteritidis in chicken by targeting outer membrane proteins. J Appl Microbiol. 2003;95(1):142–145. doi:10.1046/j.1365-2672.2003.01953.x12807464
- Scallan E, Hoekstra RM, Angulo FJ, et al. Foodborne illness acquired in the United States–major pathogens. Emerg Infect Dis. 2011;17(1):7–15. doi:10.3201/eid1701.P1110121192848
- Fabrega A, Vila J. Salmonella enterica serovar typhimurium skills to succeed in the host: virulence and regulation. Clin Microbiol Rev. 2013;26(2):308–341.23554419
- Humphrey T, Jorgensen F. Pathogens on meat and infection in animals - Establishing a relationship using campylobacter and salmonella as examples. Meat Sci. 2006;74(1):89–97. doi:10.1016/j.meatsci.2006.04.02622062719
- Lee SK, Chon JW, Song KY, Hyeon JY, Moon JS, Seo KH. Prevalence, characterization, and antimicrobial susceptibility of Salmonella Gallinarum isolated from eggs produced in conventional or organic farms in South Korea. Poult Sci. 2013;92(10):2789–2797. doi:10.3382/ps.2013-0317524046429
- Greig JD, Ravel A. Analysis of foodborne outbreak data reported internationally for source attribution. Int J Food Microbiol. 2009;130(2):77–87. doi:10.1016/j.ijfoodmicro.2008.12.03119178974
- Varmuzova K, Faldynova M, Elsheimer-Matulova M, et al. Immune protection of chickens conferred by a vaccine consisting of attenuated strains of Salmonella Enteritidis, typhimurium and infantis. Vet Res. 2016;47(1):94. doi:10.1186/s13567-016-0371-827741950
- Tennant SM, Levine MM. Live attenuated vaccines for invasive Salmonella infections. Vaccine. 2015;33(Suppl 3):C36–41. doi:10.1016/j.vaccine.2015.04.02925902362
- Okamura M, Tachizaki H, Kubo T, et al. Comparative evaluation of a bivalent killed Salmonella vaccine to prevent egg contamination with Salmonella enterica serovars Enteritidis, Typhimurium, and Gallinarum biovar Pullorum, using 4 different challenge models. Vaccine. 2007;25(25):4837–4844. doi:10.1016/j.vaccine.2007.03.00417485152
- Tran TQ, Quessy S, Letellier A, Desrosiers A, Boulianne M. Immune response following vaccination against Salmonella Enteritidis using 2 commercial bacterins in laying hens. Can J Vet Res. 2010;74(3):185–192.20885842
- Kamble NM, Lee JH. Homologous prime-boost immunization with live attenuated Salmonella enterica serovar Senftenberg and its preventive efficacy against experimental challenge with various strains of S. Senftenberg. BMC Vet Res. 2017;13(1):39. doi:10.1186/s12917-017-0960-428143524
- Wolfenden RE, Layton SL, Wolfenden AD, et al. Development and evaluation of candidate recombinant Salmonella-vectored Salmonella vaccines. Poult Sci. 2010;89(11):2370–2379. doi:10.3382/ps.2010-0070220952699
- Sheela RR, Babu U, Mu J, et al. Immune responses against Salmonella enterica serovar enteritidis infection in virally immunosuppressed chickens. Clin Diagn Lab Immunol. 2003;10(4):670–679. doi:10.1128/cdli.10.4.670-679.200312853403
- Lee YJ, Mo IP, Kang MS. Protective efficacy of live Salmonella gallinarum 9R vaccine in commercial layer flocks. Avian Pathol. 2007;36(6):495–498. doi:10.1080/0307945070169127817994329
- Barrow PA. Salmonella infections: immune and non-immune protection with vaccines. Avian Pathol. 2007;36(1):1–13. doi:10.1080/0307945060111316717364505
- Okamura M, Ueda M, Noda Y, et al. Immunization with outer membrane protein A from Salmonella enterica serovar Enteritidis induces humoral immune response but no protection against homologous challenge in chickens. Poultry Sci. 2012;91(10):2444–2449. doi:10.3382/ps.2012-0230322991526
- Prejit, Agarwal RK, Porteen K, et al. Evaluation of recombinant outer membrane protein based vaccine against Salmonella Typhimurium in birds. Biologicals. 2013;41(3):162–168. doi:10.1016/j.biologicals.2013.01.00323462815
- Meenakshi M, Bakshi CS, Butchaiah G, Bansal MP, Siddiqui MZ, Singh VP. Adjuvanted outer membrane protein vaccine protects poultry against infection with Salmonella enteritidis. Vet Res Commun. 1999;23(2):81–90. doi:10.1023/A:100625030125410359152
- Pore D, Mahata N, Pal A, Chakrabarti MK. Outer membrane protein A (OmpA) of Shigella flexneri 2a, induces protective immune response in a mouse model. PLoS ONE. 2011;6(7):e22663. doi:10.1371/journal.pone.002266321818362
- Wang G, Shi B, Li T, et al. Linear antigenic mapping of flagellin (FliC) from Salmonella enterica serovar Enteritidis with yeast surface expression system. Vet Microbiol. 2016;184:20–26. doi:10.1016/j.vetmic.2016.01.00126854340
- Barrow PA, Jones MA, Smith AL, Wigley P. The long view: salmonella–the last forty years. Avian Pathol. 2012;41(5):413–420. doi:10.1080/03079457.2012.71807123025669
- Salman HH, Irache JM, Gamazo C. Immunoadjuvant capacity of flagellin and mannosamine-coated poly(anhydride) nanoparticles in oral vaccination. Vaccine. 2009;27(35):4784–4790. doi:10.1016/j.vaccine.2009.05.09119539576
- Kim SY, Doh HJ, Jang MH, Ha YJ, Chung SI, Park HJ. Oral immunization with Helicobacter pylori-loaded poly(D, L-lactide-co-glycolide) nanoparticles. Helicobacter. 1999;4(1):33–39. doi:10.1046/j.1523-5378.1999.09046.x10352085
- Vasserman Y, Pitcovski J. Genetic detoxification and adjuvant-activity retention of Escherichia coli enterotoxin LT. Avian Pathol. 2006;35(2):134–140. doi:10.1080/0307945060059787316595306
- Dhakal S, Hiremath J, Bondra K, et al. Biodegradable nanoparticle delivery of inactivated swine influenza virus vaccine provides heterologous cell-mediated immune response in pigs. J Control Release. 2017;247:194–205. doi:10.1016/j.jconrel.2016.12.03928057521
- Ulery BD, Nair LS, Laurencin CT. Biomedical applications of biodegradable polymers. J Polym Sci B Polym Phys. 2011;49(12):832–864.21769165
- Piskin E. Biodegradable polymers as biomaterials. J Biomater Sci Polym Ed. 1995;6(9):775–795. doi:10.1163/156856295X001757772566
- Sankar R, Karthik S, Subramanian N, Krishnaswami V, Sonnemann J, Ravikumar V. Nanostructured delivery system for Suberoylanilide hydroxamic acid against lung cancer cells. Mater Sci Eng C Mater Biol Appl. 2015;51:362–368. doi:10.1016/j.msec.2015.02.03725842146
- Samal SK, Dash M, Van Vlierberghe S, et al. Cationic polymers and their therapeutic potential. Chem Soc Rev. 2012;41(21):7147–7194. doi:10.1039/c2cs35094g22885409
- Bowman K, Leong KW. Chitosan nanoparticles for oral drug and gene delivery. Int J Nanomedicine. 2006;1(2):117–128. doi:10.2147/nano.2006.1.issue-217722528
- Illum L. Nanoparticulate systems for nasal delivery of drugs: a real improvement over simple systems? J Pharm Sci. 2007;96(3):473–483. doi:10.1002/jps.2071817117404
- Wang JJ, Zeng ZW, Xiao RZ, et al. Recent advances of chitosan nanoparticles as drug carriers. Int J Nanomedicine. 2011;6:765–774. doi:10.2147/IJN.S1729621589644
- Schipper NG, Varum KM, Artursson P. Chitosans as absorption enhancers for poorly absorbable drugs. 1: influence of molecular weight and degree of acetylation on drug transport across human intestinal epithelial (Caco-2) cells. Pharm Res. 1996;13(11):1686–1692. doi:10.1023/A:10164448080008956335
- Dodane V, Amin Khan M, Merwin JR. Effect of chitosan on epithelial permeability and structure. Int J Pharm. 1999;182(1):21–32. doi:10.1016/S0378-5173(99)00030-710332071
- van der Lubben IM, Verhoef JC, Borchard G, Junginger HE. Chitosan for mucosal vaccination. Adv Drug Deliv Rev. 2001;52(2):139–144. doi:10.1016/S0169-409X(01)00197-111718937
- Koppolu B, Zaharoff DA. The effect of antigen encapsulation in chitosan particles on uptake, activation and presentation by antigen presenting cells. Biomaterials. 2013;34(9):2359–2369. doi:10.1016/j.biomaterials.2012.11.06623274070
- Quan S, Hiniker A, Collet JF, Bardwell JC. Isolation of bacteria envelope proteins. Methods Mol Biol. 2013;966:359–366.23299746
- Reboucas Jde S, Irache JM, Camacho AI, et al. Development of poly(anhydride) nanoparticles loaded with peanut proteins: the influence of preparation method on the immunogenic properties. Eur J Pharm Biopharm. 2012;82(2):241–249. doi:10.1016/j.ejpb.2012.06.01422782031
- Zhao K, Chen G, Shi XM, et al. Preparation and efficacy of a live newcastle disease virus vaccine encapsulated in chitosan nanoparticles. PLoS ONE. 2012;7(12):e53314. doi:10.1371/journal.pone.005331423285276
- Sankar R, Ravikumar V. Biocompatibility and biodistribution of suberoylanilide hydroxamic acid loaded poly (DL-lactide-co-glycolide) nanoparticles for targeted drug delivery in cancer. Biomed Pharmacother. 2014;68(7):865–871. doi:10.1016/j.biopha.2014.07.01525107842
- Salman HH, Gamazo C, Campanero MA, Irache JM. Salmonella-like bioadhesive nanoparticles. J Control Release. 2005;106(1–2):1–13. doi:10.1016/j.jconrel.2005.03.03315970347
- Ross K, Adams J, Loyd H, et al. Combination nanovaccine demonstrates synergistic enhancement in efficacy against influenza. ACS Biomater Sci Eng. 2016;2(3):368–374. doi:10.1021/acsbiomaterials.5b00477
- Miller H, Zhang J, Kuolee R, Patel GB, Chen W. Intestinal M cells: the fallible sentinels? World J Gastroenterol. 2007;13(10):1477–1486. doi:10.3748/wjg.v13.i10.147717461437
- Azizi A, Kumar A, Diaz-Mitoma F, Mestecky J. Enhancing oral vaccine potency by targeting intestinal M cells. PLoS Pathog. 2010;6(11):e1001147. doi:10.1371/journal.ppat.100114721085599
- Renu S, Markazi AD, Dhakal S, et al. Surface engineered polyanhydride-based oral Salmonella subunit nanovaccine for poultry. Int J Nanomedicine. 2018;13:8195–8215. doi:10.2147/IJN.S18558830555234
- Morachis JM, Mahmoud EA, Almutairi A. Physical and chemical strategies for therapeutic delivery by using polymeric nanoparticles. Pharmacol Rev. 2012;64(3):505–519. doi:10.1124/pr.111.00536322544864
- Xiang SD, Scholzen A, Minigo G, et al. Pathogen recognition and development of particulate vaccines: does size matter? Methods. 2006;40(1):1–9. doi:10.1016/j.ymeth.2006.05.01616997708
- Kumar S, Anselmo AC, Banerjee A, Zakrewsky M, Mitragotri S. Shape and size-dependent immune response to antigen-carrying nanoparticles. J Control Release. 2015;220(Pt A):141–148. doi:10.1016/j.jconrel.2015.09.06926437263
- Satzer P, Svec F, Sekot G, Jungbauer A. Protein adsorption onto nanoparticles induces conformational changes: particle size dependency, kinetics, and mechanisms. Eng Life Sci. 2016;16(3):238–246. doi:10.1002/elsc.v16.327478430
- Calvo P, Remuñan-López C, Vila-Jato JL, Alonso MJ. Chitosan and chitosan/ethylene oxide-propylene oxide block copolymer nanoparticles as novel carriers for proteins and vaccines. Pharm Res. 1997;14(10):1431–1436. doi:10.1023/A:10121289072259358557
- Cho Y, Park S, Barate AK, et al. Proteomic analysis of outer membrane proteins in Salmonella enterica Enteritidis. J Microbiol Biotechnol. 2015;25(2):288–295. doi:10.4014/jmb.1410.1005225418479
- Ikeda T, Kamiya R, Yamaguchi S. Excretion of flagellin by a short-flagella mutant of Salmonella typhimurium. J Bacteriol. 1983;153(1):506–510. doi:10.1128/JB.153.1.506-510.19836336739
- Katas H, Hussain Z, Awang SA. Bovine serum albumin-loaded chitosan/dextran nanoparticles: preparation and evaluation of ex vivo colloidal stability in serum. J Nanomater. 2013;9.
- Rampino A, Borgogna M, Blasi P, Bellich B, Cesaro A. Chitosan nanoparticles: preparation, size evolution and stability. Int J Pharm. 2013;455(1–2):219–228. doi:10.1016/j.ijpharm.2013.07.03423886649
- Singh B, Maharjan S, Jiang T, Kang SK, Choi YJ, Cho CS. Combinatorial approach of antigen delivery using m cell-homing peptide and mucoadhesive vehicle to enhance the efficacy of oral vaccine. Mol Pharm. 2015;12(11):3816–3828. doi:10.1021/acs.molpharmaceut.5b0026526394158
- Gao P, Xia G, Bao Z, et al. Chitosan based nanoparticles as protein carriers for efficient oral antigen delivery. Int J Biol Macromol. 2016;91:716–723. doi:10.1016/j.ijbiomac.2016.06.01527287772
- Salazar-Gonzalez RM, Srinivasan A, Griffin A, et al. Salmonella flagellin induces bystander activation of splenic dendritic cells and hinders bacterial replication in vivo. J Immunol. 2007;179(9):6169–6175. doi:10.4049/jimmunol.179.9.616917947692
- Hajam IA, Dar PA, Shahnawaz I, Jaume JC, Lee JH. Bacterial flagellin-a potent immunomodulatory agent. Exp Mol Med. 2017;49(9):e373. doi:10.1038/emm.2017.17228860663
- Vicente-Suarez I, Brayer J, Villagra A, Cheng F, Sotomayor EM. TLR5 ligation by flagellin converts tolerogenic dendritic cells into activating antigen-presenting cells that preferentially induce T-helper 1 responses. Immunol Lett. 2009;125(2):114–118. doi:10.1016/j.imlet.2009.06.00719555720
- Honko AN, Mizel SB. Mucosal administration of flagellin induces innate immunity in the mouse lung. Infect Immun. 2004;72(11):6676–6679. doi:10.1128/IAI.72.11.6676-6679.200415501801
- Agueros M, Areses P, Campanero MA, et al. Bioadhesive properties and biodistribution of cyclodextrin-poly(anhydride) nanoparticles. Eur J Pharm Sci. 2009;37(3–4):231–240. doi:10.1016/j.ejps.2009.02.01019491010
- Slutter B, Plapied L, Fievez V, et al. Mechanistic study of the adjuvant effect of biodegradable nanoparticles in mucosal vaccination. J Control Release. 2009;138(2):113–121. doi:10.1016/j.jconrel.2009.05.01119445980
- Artursson P, Lindmark T, Davis SS, Illum L. Effect of chitosan on the permeability of monolayers of intestinal epithelial cells (Caco-2). Pharm Res. 1994;11(9):1358–1361. doi:10.1023/A:10189671169887816770
- Nagarajan AG, Balasundaram SV, Janice J, Karnam G, Eswarappa SM, Chakravortty D. SopB of Salmonella enterica serovar Typhimurium is a potential DNA vaccine candidate in conjugation with live attenuated bacteria. Vaccine. 2009;27(21):2804–2811. doi:10.1016/j.vaccine.2009.02.09219428891
- Van Immerseel F, De Buck J, Pasmans F, et al. Intermittent long-term shedding and induction of carrier birds after infection of chickens early posthatch with a low or high dose of Salmonella enteritidis. Poult Sci. 2004;83(11):1911–1916. doi:10.1093/ps/83.11.191115554070
- Zaharoff DA, Rogers CJ, Hance KW, Schlom J, Greiner JW. Chitosan solution enhances both humoral and cell-mediated immune responses to subcutaneous vaccination. Vaccine. 2007;25(11):2085–2094. doi:10.1016/j.vaccine.2006.11.03417258843
- Wen ZS, Xu YL, Zou XT, Xu ZR. Chitosan nanoparticles act as an adjuvant to promote both Th1 and Th2 immune responses induced by ovalbumin in mice. Mar Drugs. 2011;9(6):1038–1055. doi:10.3390/md906103821747747
- Saeed MI, Omar AR, Hussein MZ, Elkhidir IM, Sekawi Z. Development of enhanced antibody response toward dual delivery of nano-adjuvant adsorbed human Enterovirus-71 vaccine encapsulated carrier. Hum Vaccin Immunother. 2015;11(10):2414–2424. doi:10.1080/21645515.2015.105291826186664
- Berthelot-Herault F, Mompart F, Zygmunt MS, Dubray G, Duchet-Suchaux M. Antibody responses in the serum and gut of chicken lines differing in cecal carriage of Salmonella enteritidis. Vet Immunol Immunopathol. 2003;96(1–2):43–52. doi:10.1016/S0165-2427(03)00155-714522133
- Okamura M, Lillehoj HS, Raybourne RB, Babu U, Heckert R. Antigen-specific lymphocyte proliferation and interleukin production in chickens immunized with killed Salmonella enteritidis vaccine or experimental subunit vaccines. Avian Dis. 2003;47(4):1331–1338. doi:10.1637/609614708979
- Sood S, Rishi P, Vohra H, Sharma S, Ganguly NK. Cellular immune response induced by Salmonella enterica serotype Typhi iron-regulated outer-membrane proteins at peripheral and mucosal levels. J Med Microbiol. 2005;54(Pt 9):815–821. doi:10.1099/jmm.0.46042-016091431
- Lee JS, Jung ID, Lee CM, et al. Outer membrane protein a of Salmonella enterica serovar Typhimurium activates dendritic cells and enhances Th1 polarization. BMC Microbiol. 2010;10:263. doi:10.1186/1471-2180-10-26320950448
- Jiang L, Qian F, He X, et al. Novel chitosan derivative nanoparticles enhance the immunogenicity of a DNA vaccine encoding hepatitis B virus core antigen in mice. J Gene Med. 2007;9(4):253–264. doi:10.1002/(ISSN)1521-225417397104
- Carroll EC, Jin L, Mori A, et al. The vaccine adjuvant chitosan promotes cellular immunity via DNA sensor cGAS-STING-dependent induction of Type I interferons. Immunity. 2016;44(3):597–608. doi:10.1016/j.immuni.2016.02.00426944200
- Mofazzal Jahromi MA, Karimi M, Azadmanesh K, Naderi Manesh H, Hassan ZM, Moazzeni SM. The effect of chitosan-tripolyphosphate nanoparticles on maturation and function of dendritic cells. Comp Clin Path. 2014;23(5):1421–1427. doi:10.1007/s00580-013-1799-0
- Han HD, Byeon Y, Jang JH, et al. In vivo stepwise immunomodulation using chitosan nanoparticles as a platform nanotechnology for cancer immunotherapy. Sci Rep. 2016;6:38348. doi:10.1038/srep3834827910914
- Villiers C, Chevallet M, Diemer H, et al. From secretome analysis to immunology: chitosan induces major alterations in the activation of dendritic cells via a TLR4-dependent mechanism. Mol Cell Proteomics. 2009;8(6):1252–1264. doi:10.1074/mcp.M800589-MCP20019279042
- Wu N, Wen ZS, Xiang XW, Huang YN, Gao Y, Qu YL. Immunostimulative activity of low molecular weight chitosans in RAW264.7 macrophages. Mar Drugs. 2015;13(10):6210–6225. doi:10.3390/md1310621026437419