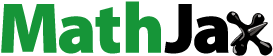
Abstract
Purpose
This research was conducted to assess the biocompatibility of the core–shell Fe3O4@ Au composite magnetic nanoparticles (MNPs), which have potential application in tumor hyperthermia.
Methods
Fe3O4@Au composite MNPs with core–shell structure were synthesized by reduction of Au3+ in the presence of Fe3O4-MNPs prepared by improved co-precipitation. Cytotoxicity assay, hemolysis test, micronucleus (MN) assay, and detection of acute toxicity in mice and beagle dogs were then carried out.
Results
The result of cytotoxicity assay showed that the toxicity grade of this material on mouse fibroblast cell line (L-929) was classified as grade 1, which belongs to no cytotoxicity. Hemolysis rates showed 0.278%, 0.232%, and 0.197%, far less than 5%, after treatment with different concentrations of Fe3O4@Au composite MNPs. In the MN assay, there was no significant difference in MN formation rates between the experimental groups and negative control (P > 0.05), but there was a significant difference between the experimental groups and the positive control (P < 0.05). The median lethal dose of the Fe3O4@Au composite MNPs after intraperitoneal administration in mice was 8.39 g/kg, and the 95% confidence interval was 6.58–10.72 g/kg, suggesting that these nanoparticles have a wide safety margin. Acute toxicity testing in beagle dogs also showed no significant difference in body weight between the treatment groups at 1, 2, 3, and 4 weeks after liver injection and no behavioral changes. Furthermore, blood parameters, autopsy, and histopathological studies in the experimental group showed no significant difference compared with the control group.
Conclusion
The results indicate that Fe3O4@Au composite MNPs appear to be highly biocompatible and safe nanoparticles that are suitable for further application in tumor hyperthermia.
Keywords:
Introduction
In the past two decades, the development and implementation of innovative processes in the field of tumor hyperthermia were being extensively studied because of the innovation of biomedical nanomaterials. Many efforts have been made to design appropriate nanoparticles and nanocomposites for hyperthermia.Citation1,Citation2 Based on the development of composite nanoparticles, core–shell superparamagnetic particles have attracted a broader interest because of their combined advantages of the heteromaterials. Among them, the applications of gold-coated iron oxide nanoparticles are of particular interest because they have not only the magnetism of iron oxide that renders them easily manipulated and heated by an external magnetic field, but also the excellent near-infrared (NIR) light sensitivity and strong adsorptive ability of the Au layer can make them useful in photothermal therapy.Citation3–Citation5 However, it should be noted that, while a considerable number of studies have been done on the synthesis and coating process of gold-coated iron oxide nanoparticles, attention devoted to the biocompatibility for in-vivo biomedical applications to ensure their safe clinical use of this kind of heteromaterial is still limited. The basic criteria for their clinical application are safety and good biocompatibility, which are also important to the industrialization of nanomedicine.Citation6,Citation7
The present authors’ earlier research has shown that Fe2O3 and Fe3O4 magnetic nanoparticles (MNPs) achieve a satisfactory thermal therapeutic effect on cell lines and animal models of liver cancer and cervical cancer in magnetic fluid hyperthermia (MFH), without histological toxicity to non-target organs or tissues.Citation8,Citation9 Furthermore, in this present study highly crystalline, monodispersed core–shell multifunctional Fe3O4@Au composite MNPs were synthesized and used in MFH combined with NIR hyperthermia. The formation of the core–shell structure was achieved in two consecutive steps, seeding the Fe3O4 core followed by coating the gold shell. Well engineered Fe3O4@Au composite MNPs tend to accumulate in the tumor due to the unorganized nature of malignancy vasculature when they are injected into an organ with a tumor, and they have strong responsiveness and low heating effect under alternating magnetic field and NIR laser exposure, which can effectively guide heat to the tumor without damaging the normal tissue.Citation5 Although gold is an inert metal, the effect on normal cell proliferation cannot be underestimated. Moreover, the nature of the composite nanoparticles could remarkably affect their biocompatibility. Thus, the toxicity and biocompatibility of the complex in vitro and in vivo need to be thoroughly evaluated before the material can be applied in hyperthermia research.Citation10 Therefore, the cytotoxicity assay, hemolysis test, micronucleus (MN) assay, and detection of acute toxicity in mice and beagle dogs were introduced to evaluate the biocompatibility of self-assembled Fe3O4@Au composite MNPs in this research.Citation11,Citation12
Materials and methods
Main apparatus and reagents
The transmission electron microscope (TEM) used was an H-600 model (Hitachi, Tokyo, Japan), and the scanning electron microscope (SEM) was a JSM-6360 model (JEOL Ltd, Akishima, Japan). The energy dispersive spectrometer (EDS) was purchased from Thermo NORAN Vantage (Waltham, MA). The laser particle size analyzer and zeta potential analyzer were purchased from Malvern Instruments Ltd (Worcestershire, UK). A 752 prismatic ultraviolet-visible (UV-vis) spectrophotometer was purchased from Shanghai Precision Scientific Instrument Co, Ltd, (Shanghai, China). 3-(4,5-dimethylthiazol-2-yl)-2,5-diphenyltetrazolium bromide (MTT) and dimethyl sulfoxide were obtained from Sigma-Aldrich (St Louis, MO). RPMI-1640 medium was purchased from Gibco-Invitrogen Corp (Carlsbad, CA). Other reagents included ferric chloride, ferrous sulfate, and ammonia water (Hanguang Chemical Reagent Co, Ltd, Shanghai, China), HAuCl4, NH2OH3HCl, and potassium oxalate (Shanghai First Reagent Factory, Shanghai, China), newborn calf serum (Si-Ji-Qing the Biotechnology Co, Ltd, Hangzhou, China), methanol (Zhenxing First Chemical Factory, Shanghai, China), cyclophosphamide (CTX) (Jiangsu Hengrui Medicine Co Ltd, Lianyungang, China), and Giemsa staining solution (Chroma, Bellows Falls, VT). All reagents were of analytical grade.
Cells and animals
Cell line L-929 was purchased from Shanghai Institute of Cell Biology (Shanghai, China). Kunming albino mice, age matched (6 weeks of age) and weight matched (18–22 g), New Zealand rabbits weight matched (2.5 kg), and beagle dogs, age matched (6–8 months of age) and weight matched (7–9 kg), were provided by the experimental animal center of Yangzhou University (Yangzhou, China). All animal experiments were evaluated and approved by the Animal and Ethics Review Committee of Southeast University (Nanjing, Jiangsu Province, China). All animals were maintained in a pathogen-free, air-conditioned environment at 24°C ± 2°C with a standard 12-hour light/12-hour dark cycle. The animals were allowed free access to tap water and food in the form of a standard pellet diet.
Preparation and characterization of Fe3O4@Au composite MNPs
Fe3O4-MNPs were prepared by the technique of chemical coprecipitation as previously published.Citation9 Fe3O4@Au core–shell composite nanoparticles were then synthesized by reduction of Au3+ in the presence of Fe3O4-MNPs, seeding the Fe3O4 core, followed by coating the gold shell. The operating procedure adopted was the following. First, the Fe3O4-MNP solution was diluted to 10 mM in deionized water, and 20 mL was stirred with 20 mL of 1 M sodium citrate for 10 minutes. Next, the solution was diluted with deionized water to 200 mL, and 1 mL of NH2OH3HCl solution of 1 M was added. Then, 1% HAuCl4 (10 mL) was incrementally added dropwise with stirring. A total of three additions (each for NH2OH3HCl and HAuCl4) were performed during the reaction, and the stirring continued for at least 30 minutes after each addition. The clear black solution became brown at first addition and changed to garnet after three iterative additions. TEM and SEM were used to observe the morphological characteristics of Fe3O4@Au composite MNPs. Meanwhile, EDS was employed to assay their composition. A laser particle size analyzer and a zeta potential analyzer were used to detect their average diameter and zeta potential. In addition, the UV-vis spectra were acquired with a UV-vis spectrophotometer, and the magnetization was measured by a vibrating sample magnetometer integrated in a physical property measurement system (PPMS-9; Quantum Design, San Diego, CA) up to 5000 Oe at 300 K.
The cytotoxicity of Fe3O4@Au composite MNPs
MTT assay was used to evaluate cell toxicity.Citation13 The sterile nanoparticles were diffused at 100 mg/mL concentration in RPMI-1640 medium (containing 10% fetal calf serum) for 72 hours at 37°C. After centrifugation at 2500 rpm for 5 minutes, the supernatant was filtered to get leaching liquor of 100% concentration. L929 fibroblast cells were seeded in 96-well plates (6000 cells per well) for 24 hours in RPMI- 1640 medium. Then they were divided into six groups (eight wells per group) and incubated with various concentrations of leaching liquor (100%, 75%, 50%, and 25% leaching liquor), RPMI-1640 medium containing 10% fetal calf serum (negative control) and 0.7% polyacrylamide (positive control) for 48 hours. Inverted microscopy was used to observe general morphological changes of the L929 cells, and then the MTT assay was performed and the optical density (OD) values were measured at 492 nm. The cell relative growth rate (RGR) was calculated as follows:
The toxicity grade is precise when the experimental results show grade 0 and grade 1. If the results show grade 2, the toxicity should be evaluated by both RGR and the morphological changes of cultured cells. The toxicity is uncertain when the results belong to other grades ().
Table 1 RGR and toxicity grade conversion
Hemolysis test of Fe3O4@Au composite MNPs
Blood was obtained from healthy New Zealand rabbits and anticoagulated with potassium oxalate, at a final concentration of 1.0 mg/mL of blood. The Fe3O4@Au composite MNPs were rinsed three times with distilled water, lixiviated with 0.9% saline, with a final concentration reached of 100 mg/mL. 0.9% saline and distilled water were used as the negative control (0% hemolysis) and the positive control (100% hemolysis), respectively. The material detected was divided into three concentration groups, ie, 100, 75, and 50 mg/mL. Each group contained three test tubes, each of which contained either 10 mL leaching liquor of the material, 0.9% saline, or distilled water. Then, 0.2 mL of diluted anticoagulated blood was added to each tube preheated for 30 minutes at 37°C. After incubation for 60 minutes at 37°C, the tubes were centrifugated at 2500 rpm for 5 minutes. Next, the supernatant fluid was assembled, and OD values were measured at 545 nm by UV-vis spectrophotometry. The hemolysis rate (HR) was calculated using the mean OD value for each group as follows:
where Dt is the absorbance of the testing sample, and Dpc and Dnc are the absorbance of the positive control and the negative control, respectively.Citation14,Citation15
MN assay of Fe3O4@Au composite MNPs
The KunMing albino mice (20–22 g) were randomly divided into six groups; there were five females and five males in every group. The animals were then treated with 100 mg/mL Fe3O4@Au composite MNPs (5.00, 3.75, 2.50, and 1.25 g/kg), 0.9% saline (as negative group) and CTX 40 mg/kg (as positive group) respectively. All experimental mice were injected intraperitoneally twice, with a 24-hour interval, and then sacrificed 6 hours after the second administration. The thighbone marrows were extracted for smears, immobilized 5 minutes with methanol, and then stained with Giemsa for 15 minutes. At least 1000 polychromatic erythrocytes (PEC) were counted for each mouse, and the rate of formation of PEC containing MN was calculated. Poisson distribution was used to analyze statistic difference between each group.Citation16
Acute toxicity testing in mice
The KunMing albino mice were randomly assigned into eight groups, with five females and five males in each group. Fe3O4@Au composite MNPs dissolved in 0.9% saline with a concentration of 100 mg/mL were injected intraperitoneally into the mice at dosages of 1.77, 2.51, 3.54, 5.00, 7.06, 9.98, 14.09, and 19.89 g/kg. The general behavior of the mice was observed continuously for 1 hour immediately after injections and then intermittently observed for a period of 24 hours. All mice were further observed for up to 14 days after treatment, and general physical states, including respiration, eating, and movement were monitored. Any signs of toxicity and deaths were recorded. Median lethal dose (LD50) was evaluated by the Karber method.Citation17 The animals were executed after the examination was finished.
Acute toxicity studies in beagle dogs
The animals were randomly divided into two groups: one experimental group and one control group, with three males and three females in each group. Normal parameters including body weight, temperature, appetite, and performance of the dogs were observed and recorded before Fe3O4@Au composite MNPs treatment. Fe3O4@Au composite MNPs dissolved in 0.9% saline with the concentration of 100 mg/mL were administered via liver injection at a dosage of 0.1 g/kg. The control group was injected with the same volume of 0.9% saline. The rate of injection was kept constant (1 mL/min) during each treatment. All animals were carefully observed for an additional 4 weeks, with necessary food and water supply. Various parameters were measured and compared with the values obtained prior to treatment. All animals were then euthanized and various parameters determined after 4 weeks.
Body general parameters
All animals were weighed once every week. The objective signs, including cleanliness, behavior, and food intake were recorded every day.
Blood analysis
Blood samples were collected from the veins of the animals before administration and every week up to 4 weeks after treatment. The pertinent blood indices were determined with routine methods and an autoanalyzer.Citation18
Autopsy study
Four weeks after administration, all animals were sacrificed by exsanguination and dissection. During the process of dissecting, the parenchymatous organs’ color, texture, and lumps were carefully examined. In the meantime, the weight of the heart, liver, spleen, lung, kidney, and brain were measured and recorded. The organ–body index was calculated according to the following formula:Citation19
Histopathological study
Small organ pieces (3–5 mm thick) were fixed in 10% formal-saline (0.9% saline in 10% formaldehyde) for 24 hours and washed in running water for another 24 hours. Samples were dehydrated by passing through 50%, 70%, 90%, and 100% alcohol over a 2-day period, and then cleared in benzene to remove alcohol until the tissues became transparent. This was followed by staining with hematoxylin-eosin and thorough examination using a light microscope.Citation20,Citation21
Statistical analysis
Data were analyzed using SPSS Statistics (v 11.5; IBM Corporation, Somers, NY) software. The statistical significance of differences in mean values between the groups was analyzed using one-way analysis of variance. P-values <0.05 were considered statistically significant.
Results
Characterization of Fe3O4@Au composite MNPs
The self-prepared Fe3O4@Au composite MNPs were approximately spherical, high electron-dense, and uniform in size as observed by TEM and SEM (). Meanwhile, EDS confirmed that the prepared nanoparticles contained the elements of Fe, Au, and O ( inset). The average diameter detected by Malvern laser particle size analyzer was about 35 nm, with a narrow diameter distribution for Fe3O4@Au composite MNPs () and about 20 nm for Fe3O4-MNPs ( inset). And the zeta potential of Fe3O4@Au composite MNPs at pH 7.4 was −23.2 ± 1.8 mV () compared with the zeta potential value of −3.6 ± 1.8 mV for Fe3O4-MNPs ( inset). Moreover, the UV-vis absorption spectra of Fe3O4@Au composite MNPs indicated 612 nm of the absorption peak (, curve b), while the Fe3O4-MNPs absorption spectra were increased with the wavelength decrease in the range 900–300 nm (, curve a). The magnetic hysteresis loops measured at 300 K showed that the saturation magnetization (Ms) value of Fe3O4-MNPs was 75.5 emu/g (, curve a), while the value of Fe3O4@Au MNPs was 51.8 emu/g (, curve b).
Figure 1 Characterization of Fe3O4@Au composite MNPs. (A) Transmission electron microscopy image; (B) scanning electron microscopy image and energy dispersive spectrometry (inset); (C) the average diameter of Fe3O4@Au composite MNPs and Fe3O4-MNPs (inset); (D) the zeta potential of Fe3O4@Au composite MNPs and Fe3O4-MNPs (inset); (E) ultraviolet-visible absorption spectra of Fe3O4@Au composite MNPs (curve b) and Fe3O4-MNPs (curve a); and (F) hysteresis loops of Fe3O4@Au composite MNPs (curve b) and Fe3O4-MNPs (curve a).
Abbreviation: MNPs, magnetic nanoparticles.
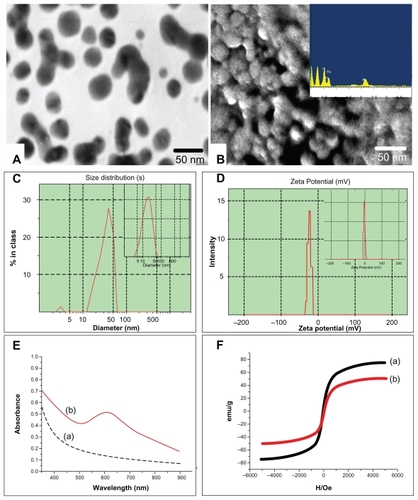
The cytotoxicity of Fe3O4@Au composite MNPs
The morphological changes of L929 cells after treatment with different concentrations of Fe3O4@Au composite MNPs leaching liquor were observed by inverted microscopy. As shown in , the shapes and growth of the treated cells were similar to those of cells in the negative group (). They exhibited normal features, such as clear edges, homogeneous staining, and no cell fragments, while the cells of the positive group became small and globular, and even parts of cells were suspended 48 hours later (). Only small amounts of cells survived. The results of the MTT assay are shown in . According to RGR and toxicity grade conversion table (see ), the toxicity of Fe3O4@Au composite MNPs leaching liquor was classified as grade 1, which is safe to the cells. This was in agreement with the findings from inverted microscopy and demonstrated that Fe3O4@Au composite MNPs did not show cytotoxicity in vitro.
Figure 2 Morphology analysis of L929 cells incubated at different concentrations of Fe3O4@Au composite magnetic nanoparticles leaching liquor. (A) Negative control; (B) 25% leaching liquor; (C) 50% leaching liquor; (D) 75% leaching liquor; (E) 100% leaching liquor; and (F) positive control.
Note: Inverted microscopy, ×100 magnification.
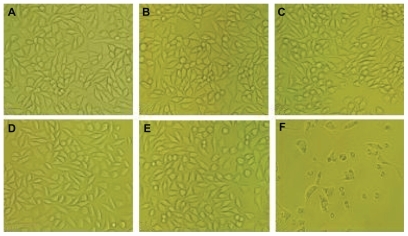
Figure 3 The MTT results of Fe3O4@Au composite magnetic nanoparticles.
Notes: n = 8, mean ± standard deviation. The RGR of L929 cells incubated with 25%, 50%, 75%, and 100% leaching liquor were 0.977, 0.872, 0.849, and 0.826, respectively, which all classified as toxicity grade 1.
Abbreviations: MTT, 3-(4,5-dimethylthiazol-2-yl)-2,5-diphenyltetrazolium bromide; OD, optical density; RGR, relative growth rate.
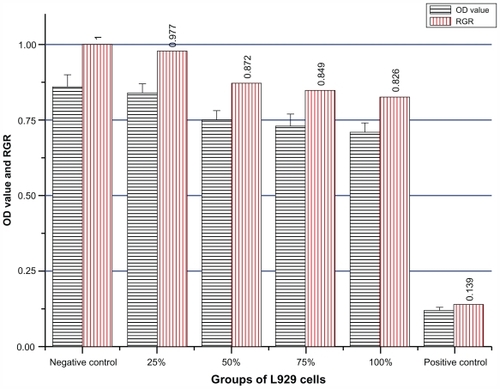
Hemolysis experimental results of Fe3O4@Au composite MNPs
The hemolysis rates at 545 nm are listed in for each experimental group. Hemolysis rates at the different concentrations of Fe3O4@Au composite MNPs were 0.278%, 0.232%, and 0.197%, respectively, far less than 5%, which is the threshold for a hemolytic reaction.Citation14
Table 2 Hemolysis test of Fe3O4@Au composite magnetic nanoparticles
MN results of Fe3O4@Au composite MNPs
In this study, the MN formation rates of 5.00, 3.75, 2.50, and 1.25 g/kg experimental groups, negative control group, and positive control group were 2.5‰, 2.7‰, 2.6‰, 2.3‰, 2.1‰, and 26.2‰ respectively (), showing a significant difference between the experimental groups and the positive control group (P < 0.05). But MN formation rates at different doses of experimental groups showed no statistical difference compared with the negative control group (P > 0.05).
Figure 4 The results of MN test of Fe3O4@Au composite magnetic nanoparticles.
Notes: n = 10. aP > 0.05, MN formation rates of Fe3O4@Au groups compared with negative control; bP < 0.05, MN formation rates between Fe3O4@Au groups and positive control.
Abbreviations: MN, micronucleus; PEC, polychromatic erythrocytes.
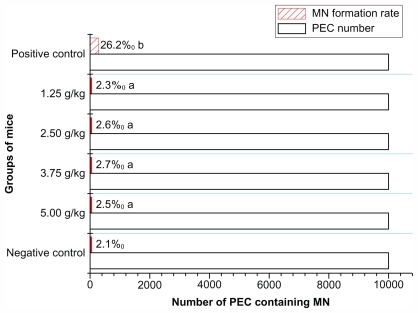
Acute toxicity in mice
Some behavioral changes such as crouching, sluggishness, bradypnea, and slow response to external stimuli were observed among some animals immediately after Fe3O4@Au composite MNP administration. However, some resumed normal activity about 2 hours after the treatment. Interestingly, Fe3O4@Au composite MNP administration of 1.77 g/kg did not bring any notable changes to the animals. The deaths of most mice occurred during the first day after administration (). Mortality rates in the treatment groups were used to calculate the LD50 of Fe3O4@Au composite MNPs, which evaluate short-term toxicity after intraperitoneal administration. The LD50 of the material to the mice was 8.39 g/kg, and its 95% confidence interval (CI) was 6.58–10.72 g/kg from the acute toxicological study.
Table 3 The results of acute toxicity testing of Fe3O4@Au composite MNPs
Acute toxicity in beagle dogs
Effect of Fe3O4@Au composite MNPs on the general behavior
The acute general toxicity test was used to evaluate short-term toxicity after liver administration. Behavioral changes including slobbering, astasia, instability of gait, convulsion, anepithymia, and respiratory depression were not observed among animals in the experimental group. Both control group and experimental group animals displayed normal physical behavior during the 4-week observation period. All animals were weighed once a week. There was no significant difference in body weight values of beagle dogs between the experimental group and the control group (P > 0.05; ).
Figure 5 Effect of Fe3O4@Au composite MNPs administered via liver injection on body weight (kg) in beagle dogs.
Notes: n = 6, mean ± standard deviation. There was no significant difference in body weight values of beagle dogs between the experimental group and the control group at the five time points of before administration and 1, 2, 3, and 4 weeks after administration of Fe3O4@Au composite MNPs (P > 0.05).
Abbreviation: MNPs, magnetic nanoparticles.
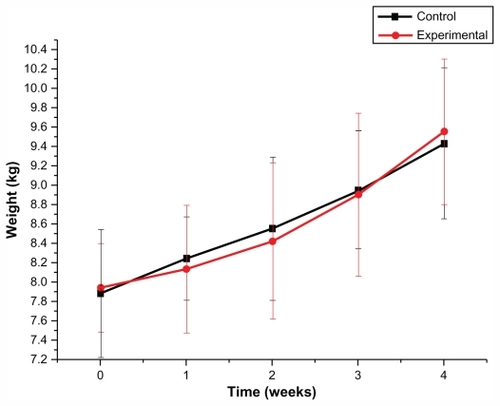
Effect of Fe3O4@Au composite MNPs on the biochemical and hematological parameters
The effect of liver injection of Fe3O4@Au composite MNPs on alanine aminotransferase (ALT), aspartic acid aminotransferase (AST), blood urea nitrogen (BUN), and creatinine (Cr) are presented in and , showing no significant differences between the experimental group and the control group in the data of ALT, AST, BUN, and Cr during the 4 weeks (P > 0.05). Furthermore, no significant differences were observed for hematological parameters between the experimental group and the control group (P > 0.05; detailed data can be seen in ).
Figure 6 Liver function of beagle dogs in 4-week toxicity study of Fe3O4@Au composite MNPs.
Notes: n = 6, mean ± standard deviation. ALT and AST of the experimental group administered with Fe3O4@Au composite MNPs showed no significant difference compared with the control group (P > 0.05).
Abbreviations: MNPs, magnetic nanoparticles; ALT, alanine aminotransferase; AST, aspartic acid aminotransferase.
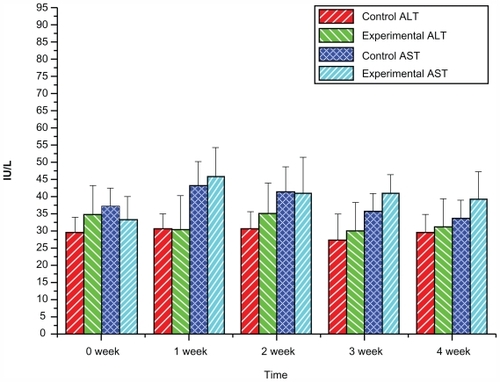
Figure 7 Renal function of beagle dogs in 4-week toxicity study of Fe3O4@Au composite MNPs.
Notes: n = 6, mean ± standard deviation. BUN and Cr of the experimental group administered with Fe3O4@Au composite MNPs showed no significant difference compared with the control group (P > 0.05).
Abbreviations: MNPs, magnetic nanoparticles; BUN, blood urea nitrogen; Cr, creatinine.
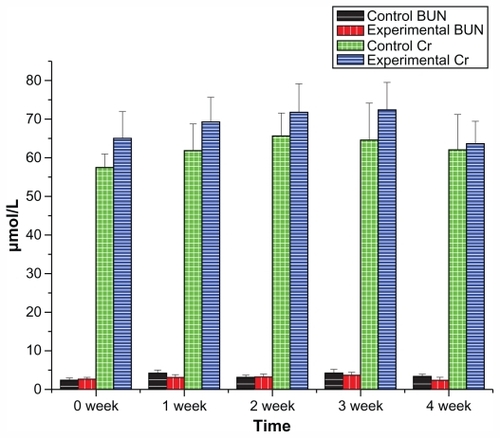
Effect of Fe3O4@Au composite MNPs on the organs
The autopsy study was carried out 4 weeks after the animals were treated with Fe3O4@Au composite MNPs. The organs, including heart, liver, spleen, lung, kidney, brain, adrenal glands, and pancreas, were carefully examined and investigated. No noticeable pathological changes such as swelling, shrinking, nodules, collapse, hard texture, and thickened capsule could be observed by the naked eye. No significant differences in organ weight or organ–body weight indices were found between the experimental group and the control group (P > 0.05; ).
Figure 8 Effect of Fe3O4@Au composite magnetic nanoparticles administered via liver injection on organ body weight indices of beagle dogs after 4 weeks.
Notes: n = 6, mean ± standard deviation. There was no significant difference in organ body weight indices including heart, liver, spleen, lung, kidney, and brain between the experimental group and the control group (P > 0.05).
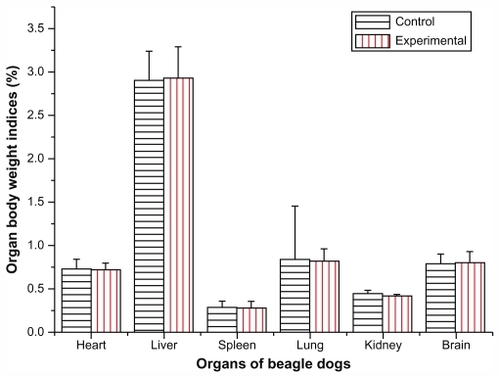
Effect of Fe3O4@Au composite MNPs on histopathological changes
Histopathological investigation was performed after the autopsy study. From the histological sections (), no significant pathological changes were detected in the liver tissue of experimental dogs, except for lots of Fe3O4@ Au composite MNPs taken up by cells around the needle passage and a small area of mechanical damage. Other organs including heart, spleen, lung, kidney, and brain showed no sign of pathological changes such as edema, atrophy, steatosis, liquefaction necrosis, hyalinization, calcification, and hemosiderosis compared with the corresponding organs from the dogs in the control group ().
Figure 9 Effect of Fe3O4@Au composite MNPs administered via liver injection on the tissue of beagle dogs after 4 weeks (hematoxylin-eosin staining, ×100 magnification). (A1) Little impact on the liver of the control group; (A2) Fe3O4@Au composite MNPs were taken up by cells around the needle passage in the liver of the experimental group, indicated by arrows; (B–F) no significant pathological changes were detected in the tissues including heart (B1 and B2), spleen (C1 and C2), lung (D1 and D2), kidney (E1 and E2), and brain (F1 and F2).
Abbreviation: MNPs, magnetic nanoparticles.

Discussion
Recently, the unique structure and magnetic-optical properties of Fe3O4@Au composite MNPs have made them promising materials for a wide range of biological applications such as cell labeling, tracking, imaging, gene delivering, and sensing. Particularly, there are potential applications in the intracellular/interstitial MFH and NIR hyperthermia.Citation22–Citation25 However, there is concern about the potential toxicological effects of them in vitro and in vivo. At present, it is widely recognized that Fe3O4-MNPs are metabolized, stored, and transported through human tissues by proteins including ferritin, transferritin, hemosiderin, and others, such that the resultant iron is incorporated into the iron pool. Many researchers have synthesized Fe3O4-MNPs successfully with satisfactory biocompatibility and explored their biomedical applications.Citation26–Citation28 On the other hand, some researchers reported that bare Fe3O4-MNPs induced the formation of free hydroxyl radical species that reacted with intracellular constituents such as the cell’s endogenous DNA, thus inhibiting cellular function and proliferation.Citation29,Citation30 Moreover, it was found that exposure to dextran- or citric acid-coated Fe3O4-MNPs, of an approximate size of 31–38 nm, resulted in a dose-dependent decrease of HUVEC viability in vitro.Citation31 These results confirm that the toxicity of Fe3O4-MNPs affect the biocompatibility and biosafety in vitro. Meanwhile, it was reported that the gold shell coating on the Fe3O4-MNPs surface improved the biocompatibility for cellular application, particularly at high magnetite loading. The improved biocompatibility may be attributed to the presence of gold shells which prevents the leaching of iron in acidic intracellular degradation process.Citation32 Similarly, the protective properties of the gold coating have also been reported by others.Citation33 However, the literature also reported conflicting data regarding the cytotoxicity of gold nanoparticles in vitro. For example, some researchers demonstrated that different sized gold nanoparticles with varying surface chemistries were not toxic to human cells.Citation34 But others reported that gold nanoparticles demonstrated a size-dependent toxicity for sizes ranging from 0.8–15 nm.Citation35 Moreover, it was discovered that the electrical charge of the nanoparticle played a key role in determining toxicity, with cationic gold nanoparticles displaying moderate toxicity, while their anionic counterparts exhibited no toxic effects.Citation36 Interestingly, Alkilany et al reported that gold nanoparticles bore the same effective charge in the biological media because serum proteins adsorbed to gold nanoparticles, regardless of the initial surface charge, suggesting that physiochemical surface properties of nanomaterials change substantially after coming into contact with biological media. Such changes should be taken into consideration when examining the biological properties or environmental impact of nanoparticles.Citation37 Based on these results, it is imperative to systematically evaluate the biocompatibility of Fe3O4@Au composite MNPs and their toxicity within biological systems, especially in vivo, since results from in-vitro and in-vivo testing may be quite different.
A previous study reported the synthesis strategy involving a hetero-interparticle coalescence of molecularly capped Au nanoparticles (2 nm) and iron-oxide nanoparticles (30–50 nm) as precursors in mixed solution, leading to core–shell nanoparticles. The results showed that gold shell- coated Fe3O4-MNPs existed as aggregates with a broad size distribution, and the longest aggregate dimension varied from 100 nm to 2 μm. However, they exhibited improved biocompatibility in mammalian cells.Citation32 The strategy used in this present study involves sequential formation of the iron-oxide core and gold shell, and the composite particles were prepared by the reduction of Au3+ with hydroxylamine in the presence of Fe3O4-MNPs, with the average diameter of 20 nm as seeds. Compared with other reports,Citation3,Citation32 the Fe3O4@Au composite MNPs in this present study were proved to retain the quasi-spherical shape and uniform size by TEM and SEM. The results of laser particle size analysis illustrated that the average diameter of Fe3O4-MNPs was about 20 nm. After modification of the Fe3O4-MNPs with gold shell, the average diameter of the resulting core–shell Fe3O4@Au composite MNPs was about 35 nm, with a narrow diameter distribution. Thus, the thickness of the gold shell was estimated to be 7–8 nm. However, proper shell thickness is essential to obtain new optical properties and retain enough magnetism characteristics for Fe3O4@Au composite MNPs. UV-vis spectra were therefore obtained to investigate the preparation and the optical properties of the self-prepared Fe3O4@Au composite MNPs. The UV-vis absorption spectra showed that Fe3O4-MNP absorption spectra had no dramatic absorption in the visible region. However, after the Fe3O4-MNPs were coated with gold shell, 612 nm of the absorption peak was remarkably observed owing to the surface plasmon, indicating that the gold shell layer was formed on the surface of the Fe3O4 core, consistent with the observation from other reports.Citation3,Citation4 In contrast to the literature, the Fe3O4@Au composite MNPs in this present study had broader absorption spectra and redshifted maximum absorption wavelength.Citation3,Citation38,Citation39 Such a redshift phenomenon of the plasmon absorption can be understood in terms of plasmon hybridization with the gold shell layer growth. In addition, because the gold shell layer outside the Fe3O4 core forms a rough surface after a coating of three iterations, strong interaction and coupling of the surface plasmon exists between neighboring nanoparticles, which may also contribute to the plasmon resonance redshift.Citation40
Furthermore, the magnetic measurement showed that the Fe3O4@Au composite MNPs in this present study retained superparamagnetic properties with low coercivity and high Ms at room temperature, similar to Fe3O4-MNPs used in MFH.Citation9 The Ms values of Fe3O4-MNPs and Fe3O4@Au composite MNPs were estimated to be 75.5 and 51.8 emu/g, respectively, which are much lower than that of bulk Fe3O4 (Ms = 84 emu/g). Generally, the Ms decreased significantly when the gold shell formed on the surface of Fe3O4 MNPs. This reduction is expected as the surface magnetic order can be affected by structural distortions that cause spin canting.Citation41 However, the Ms of Fe3O4@Au composite MNPs is higher than that from other research.Citation39,Citation40 In addition, the detection of effective surface charge showed that the zeta potential of Fe3O4-MNPs was close to neutral charge (−3.6 ± 1.8 mV) at pH 7.4, the presence of gold shell shifted the zeta potential value to −23.2 ± 1.8 mV. A slightly high negative zeta potential imparted by Fe3O4@Au composite MNPs compared with Fe3O4-MNPs confirms an amount of gold coverage on the magnetite surface, which was in agreement with the literature.Citation32
Next in this present study, the toxicity of self-prepared Fe3O4@Au composite MNPs was investigated through MTT assay, hemolysis test, MN assay, and acute toxicity testing in rodent and Canidae animals. The MTT assay is very often used to evaluate cell proliferation and the viability for biomaterial toxicity.Citation13 In this present experiment, Fe3O4@Au composite MNPs with various concentrations of leaching liquor (100%, 75%, 50%, and 25%) were adjusted to test the cytotoxicity on L929 cells. The morphological observations showed that no significant toxic effects were seen compared with the negative control under the microscope, indicating that Fe3O4@Au composite MNPs have a minimal impact on the cell population as the cells remained viable and continued to proliferate. The toxicity of Fe3O4@Au composite MNPs was classified as grade 1, suggesting that they have good biocompatibility for cellular application, consistent with the literature, which reported negligible impact of Fe3O4@Au with amine functional group on mammalian cells.Citation42
The hemolysis test is mainly used to evaluate the hemocompatibility of materials by detecting hemolyzation of erythrocytes. The erythrocyte membrane integrity may be destroyed by both mechanical injury of the particle surface and the chemical action of soluble remnant molecules, resulting in hemolyzation when Fe3O4@Au composite MNPs are used for thermotherapy. So the extent of hemolysis could be calculated by detecting the absorbance of released free hemoglobin using ultraviolet spectrophotometry.Citation43,Citation44 In this study, hemolysis rates at different concentrations of Fe3O4@ Au composite MNPs were all far less than 5%, which is the threshold for a hemolytic reaction, indicating that Fe3O4@ Au composite MNPs do not cause hemolysis and have good hemocompatibility, which is in agreement with previous studies.Citation11,Citation16
The short-term genetic toxicity was tested by MN assay, which is a rapid detection method of chromosome damage and interference with cell mitosis caused by the biological materials. If the result of this test is negative, it isn’t necessary to perform the carcinogenic test.Citation45 Previously, Wu et al adopted the dosages of 5.00–1.25 g/kg to assess the short-term genetic toxicity of self-assembled Fe3O4-MNPs loaded with daunorubicin (DNR).Citation16 In this study, the MN formation rates of experimental groups with different dosages from 5.00 to 1.25 g/kg showed no statistical difference compared with the negative control group, but a significant difference compared with the positive control group, suggesting Fe3O4@Au composite MNPs have no inherent toxicity to bone-marrow cells in mice. The results are similar to the MN assay of Fe3O4-MNPs and Fe3O4-MNPs loaded with DNR.Citation11,Citation16 Thus, it can be inferred that these nanoparticles do not induce cacogenesis or mutations.
The acute toxicity testing was used to evaluate short-term toxicity after intraperitoneal administration in mice. In tumor thermotherapy, it is crucial to optimize dosing not only for effectively heating tumor tissue or promoting apoptosis of tumor cells, but also for reducing the systemic side effects. The LD50 of Fe3O4@Au composite MNPs administered by intraperitoneal injection was determined to be 8.39 g/kg in mice, and its 95% CI was found to fall in the range of 6.58–10.72 g/kg from the acute toxicological study. In contrast to Fe3O4-MNPs with the LD50 arriving at 7.57 g/kg and Mn0.5Zn0.5Fe2O4-MNPs at 7.19 g/kg, Fe3O4@ Au composite MNPs have a wider safety margin.Citation11,Citation46 These parameters provide critical guidelines for the administration of this material in future studies.
Previous research of pharmacokinetics and tissue distribution in mice showed that Fe3O4-MNPs were mostly taken up in the liver, suggesting the curative effect could be more pronounced for tumors in the liver.Citation47 But the hepatotoxicity following gold salt therapy also has been previously reported, while accumulation of gold particles around hepatic veins, probably within macrophages, was observed, although no abnormal changes on the hematological parameters were observed.Citation48 Therefore, investigated in this present paper was whether Fe3O4@Au composite MNPs could cause short-term systemic toxic reactions during a 4-week period after liver injection in beagle dogs. The dosage of Fe3O4@Au composite MNPs via liver injection was 100 mg/kg, which was also adopted in the acute toxicological observations of Fe3O4-MNPs loaded with DNR.Citation16 Typical toxicological responses such as slobbering, astasia, and anepithymia were not observed. Meanwhile, the body weights of the animals were measured every week, since body weight loss has been used as an indicator of adverse effects of drugs and chemicals.Citation49 There was no significant difference in body weight of beagle dogs after administration of Fe3O4@Au composite MNPs compared with the control group. These results imply that liver injection of Fe3O4@Au composite MNPs made a negligible impact on the general behavior of beagle dogs. Moreover, the levels of ALT, AST, BUN, and Cr did not increase significantly when compared with those of the control group, suggesting that Fe3O4@Au composite MNPs did not cause both liver and kidney injury.
In addition, no abnormal changes of the hematological parameters were observed compared with the control group, showing that the lymphohemopoietic system was not affected by the Fe3O4@Au composite MNPs administered to the beagle dogs. Furthermore, it was demonstrated that Fe3O4@Au composite MNPs did not induce lesions of organs and tissues by both autopsy study and histopathological investigation.
Meanwhile, it is noteworthy that Fe3O4@Au composite MNPs were almost ingested and deposited in the liver because no nanoparticles and hemosiderosis were observed in other organs including heart, spleen, lung, kidney, and brain, confirming the potential application in the intracellular and interstitial MFH combined with NIR hyperthermia on liver tumors with organ targeting and safety to biological systems in general. Thus, summarizing all the experimental results, it can be assumed that Fe3O4@Au composite MNPs do not cause any short-term systemic toxic reactions in beagle dogs with the dosage used in this study. In contrast to the investigation on the biocompatibility of magnetic albumin nanosphere (MAN) in a very recent publication, Fe3O4@Au MNPs indicate desirable biocompatibility in vivo at the high concentration of 100 mg/mL when MAN showed good biocompatibility at the concentration of 5 mg/mL in mice.Citation50
Conclusion
In conclusion, the results of this study show that Fe3O4@Au composite MNPs appear to be highly biocompatible and safe nanoparticles according to the evaluation of toxicity in vivo and in vitro. Considering that some of the Food and Drug Administration-approved MRI contrast agents are made of Fe3O4, Fe3O4@Au composite MNPs have a potential to be used as safe optical and thermal agents, allowing the combination of cancer detection and cancer-specific hyperthermic treatment. The results of this study provide experimental foundation for further clinical research and evaluation of this promising material.
Acknowledgments
This work was supported by the Chinese National 863 Plan (No. 2007AA03Z356), the Chinese National Natural Science Foundation (No. 30770584 and No. 81171452), and the Science and Technology Development Foundation of Nanjing Medical University (No. 08NMUZ015).
Supplementary data
Table S1 Hematological values of beagle dogs in 4-week toxicity study of Fe3O4@Au composite MNPs
Disclosure
The authors report no conflicts of interest in this work.
References
- JordanAScholzRWustPEffects of magnetic fluid hyperthermia (MFH) on C3H mammary carcinoma in vivoInt J Hyperthermia1997135876059421741
- O’NealDPHirschLRHalasNJPayneJDWestJLPhoto-thermal tumor ablation in mice using near infrared-absorbing nanoparticlesCancer Lett200420917117615159019
- WangLLuoJFanQMonodispersed core-shell Fe3O4@Au nanoparticlesJ Phys Chem B2005109215932160116853803
- LiuHLSonnCHWuJHLeeKMKimYKSynthesis of streptavidin- FITC-conjugated core-shell Fe3O4-Au nanocrystals and their application for the purification of CD4+ lymphocytesBiomaterials2008294003401118649937
- JinHKangKAApplication of novel metal nanoparticles as optical/thermal agents in optical mammography and hyperthermic treatment for breast cancerAdv Exp Med Biol2007599455217727246
- KouassiGKIrudayarajJMagnetic and gold-coated magnetic nanoparticles as a DNA sensorAnal Chem2006783234324116689521
- GuptaAKGuptaMSynthesis and surface engineering of iron oxide nanoparticles for biomedical applicationsBiomaterials2005263995402115626447
- YanSZhangDZhengJStudy on the therapeutic effect of Fe2O3 nanometer magnetic fluid hyperthermia on liver cancerChina Journal of Experimental Surgery20042114431446
- DuYZhangDLiuHLaiRThermochemotherapy effect of nanosized As2O3/Fe3O4 complex on experimental mouse tumors and its influence on the expression of CD44v6, VEGF-C and MMP-9BMC Biotechnol200998419804631
- KirkpatrickCJBittingerFWagnerMCurrent trends in biocompatibility testingProc Inst Mech Eng H199821275849611998
- DuYZhangDNiHThe biocompatibility of Fe3O4 magnetic nanoparticles used in tumor hyperthermiaJournal of Nanjing University (Natural Science)200642324330
- YanSZhangDGuNThe biocompatibility study of magnetic nanoparticles containing Fe2O3 used in tumor hyperthermiaJ Southeast Univ (Med Sci Edi)200524812
- PietersRLoonenAHHuismansDRIn vitro drug sensitivity of cells from children with leukemia using the MTT assay with improved culture conditionsBlood199076232723362257305
- SunJGuGZQianYFInfluence of different contact ways and extracting conditions on the hemolytic effect of biomaterialsJ Biomed Eng200320810
- ZhangWYShenYLiNEvaluation of biocompatibility of fiber-reinforced dental compositesMed J Chin PLA200429345347
- WuWChenBChengJBiocompatibility of Fe3O4/DNR magnetic nanoparticles in the treatment of hematologic malignanciesInt J Nanomedicine201051079108421170355
- TwaijHAKeryAAl-KhazrajiNKSome pharmacological, toxicological and phytochemical investigations on Centaurea phyllocephalaJ Ethnopharmacol198392993146677820
- LottJAPractical problems in clinical enzymologyCRC Crit Rev Clin Lab Sci19778277301200402
- GuoQQiQYouQGuHZhaoLWuZToxicological studies of gambogic acid and its potential targets in experimental animalsBasic Clin Pharmacol Toxicol20069917818416918721
- AkdoganMKilinçIOncuMKaraozEDelibasNInvestigation of biochemical and histopathological effects of Mentha piperita L. and Mentha spicata L. on kidney tissue in ratsHum Exp Toxicol20032221321912755472
- Abd-ElhamidHFInvestigation of induced biochemical and histopathological parameters of acetonitril extract of Jatropha carcus in albino ratsJ Egypt Soc Parasitol20043439740615287166
- QiuJDXiongMLiangRPPengHPLiuFSynthesis and characterization of ferrocene modified Fe3O4@Au magnetic nanoparticles and its applicationBiosens Bioelectron2009242649265319230651
- BarrySEChallenges in the development of magnetic particles for therapeutic applicationsInt J Hyperthermia20082445146618608583
- HirschLRStaffordRJBanksonJANanoshell-mediated near-infrared thermal therapy of tumors under magnetic resonance guidanceProc Natl Acad Sci U S A2003100135491355414597719
- SongHMWeiQOngQKWeiAPlasmon-resonant nanoparticles and nanostars with magnetic cores: synthesis and magnetomotive imagingACS Nano201045163517320690598
- DaveSRGaoXMonodisperse magnetic nanoparticles for biodetection, imaging, and drug delivery: a versatile and evolving technologyWiley Interdiscip Rev Nanomed Nanobiotechnol2009158360920049819
- SunJZhouSHouPSynthesis and characterization of biocompatible Fe3O4 nanoparticlesJ Biomed Mater Res A20078033334117001648
- ChengFYSuCHYangYSCharacterization of aqueous dispersions of Fe(3)O(4) nanoparticles and their biomedical applicationsBiomaterials20052672973815350777
- EmeritJBeaumontCTrivinFIron metabolism, free radicals, and oxidative injuryBiomed Pharmacother20015533333911478586
- van den BosEJWagnerAMahrholdtHImproved efficacy of stem cell labeling for magnetic resonance imaging studies by the use of cationic liposomesCell Transplant20031274375614653621
- WuXTanYMaoHZhangMToxic effects of iron oxide nanoparticles on human umbilical vein endothelial cellsInt J Nanomedicine2010538539920957160
- ArsiantiMLimMLouSNGoonIYMarquisCPAmalRBi- functional gold-coated magnetite composites with improved biocompatibilityJ Colloid Interface Sci201135453654521131002
- Ow SullivanMMGreenJJPrzybycienTMDevelopment of a novel gene delivery scaffold utilizing colloidal gold-polyethylenimine conjugates for DNA condensationGene Ther2003101882189014502217
- ConnorEEMwamukaJGoleAMurphyCJWyattMDGold nanoparticles are taken up by human cells but do not cause acute cytotoxicitySmall2005132532717193451
- PanYNeussSLeifertASize-dependent cytotoxicity of gold nanoparticlesSmall200731941194917963284
- GoodmanCMMcCuskerCDYilmazTRotelloVMToxicity of gold nanoparticles functionalized with cationic and anionic side chainsBioconjug Chem20041589790015264879
- AlkilanyAMNagariaPKHexelCRShawTJMurphyCJWyattMDCellular uptake and cytotoxicity of gold nanorods: molecular origin of cytotoxicity and surface effectsSmall2009570170819226599
- CuiYWangYHuiWZhangZXinXChenCThe synthesis of Gold- Mag nano-particles and their application for antibody immobilizationBiomed Microdevices2005715315615940431
- ZhouXXuWWangYFabrication of cluster/shell Fe3O4/Au nanoparticles and application in protein detection via a SERS methodJ Phys Chem C20101141960719613
- LevinCSHofmannCAliTAMagnetic-plasmonic core-shell nanoparticlesACS Nano200931379138819441794
- PalSMoralesMMukherjeePSrikanthHSynthesis and magnetic properties of gold coated iron oxide nanoparticlesJ Appl Phys2009707B504-07B504503
- WangFHKimDKYoshitakeTDiffusion and clearance of superparamagnetic iron oxide nanoparticles infused into the rat striatum studied by MRI and histochemical techniquesNanotechnology20112201510321135466
- XiTFBiological evaluation of biology based on medical devicesChina Medical Device Information19995914
- LiuWWWangTZhanDSHaemolysis test for evaluating the Biocompatibility of SiC implant materialsCRTER20081218731875
- ZhaoXWLinJHWangZRStudy on biocompatibility and security of homemade calcium phosphate cementJ Fujian Med University200236388392
- LiuJZhangJWangLLiYZhangDBiocompatibility study of Mn0.5Zn0.5Fe2O4 magnetic nanoparticlesKey Eng Mater2011483552558
- WangJChenYChenBPharmacokinetic parameters and tissue distribution of magnetic Fe(3)O(4) nanoparticles in miceInt J Nanomedicine2010586186621042548
- BassetCVadrotJDenisJPouponJZafraniESProlonged cholestasis and ductopenia following gold salt therapyLiver Int201023899312654130
- TofovicSPJacksonEKEffects of long-term caffeine consumption on renal function in spontaneously hypertensive heart failure prone ratsJ Cardiovasc Pharmacol19993336036610069669
- EstevanatoLCintraDBaldiniNPreliminary biocompatibility investigation of magnetic albumin nanosphere designed as a potential versatile drug delivery systemInt J Nanomedicine201161709171721980234