Abstract
Background
Mesoporous bioactive glasses (MBGs) are very attractive materials for use in bone tissue regeneration because of their extraordinarily high bone-forming bioactivity in vitro. That is, MBGs may induce the rapid formation of hydroxy apatite (HA) in simulated body fluid (SBF), which is a major inorganic component of bone extracellular matrix (ECM) and comes with both good osteoconductivity and high affinity to adsorb proteins. Meanwhile, the high bioactivity of MBGs may lead to an abrupt initial local pH variation during the initial Ca ion-leaching from MBGs at the initial transplant stage, which may induce unexpected negative effects on using them in in vivo application. In this study we suggest a new way of using MBGs in bone tissue regeneration that can improve the strength and make up for the weakness of MBGs. We applied the outstanding bone-forming bioactivity of MBG to coat the main ECM components HA and collagen on the MBG-polycarplolactone (PCL) composite scaffolds for improving their function as bone scaffolds in tissue regeneration. This precoating process can also expect to reduce initial local pH variation of MBGs.
Methods and materials
The MBG-PCL scaffolds were immersed in the mixed solution of the collagen and SBF at 37°C for 24 hours. The coating of ECM components on the MBG-PCL scaffolds and the effect of ECM coating on in vitro cell behaviors were confirmed.
Results
The ECM components were fully coated on MBG-PCL scaffolds after immersing in SBF containing dilute collagen-I solution only for 24 hours due to the high bone-forming bioactivity of MBG. Both cell affinity and osteoconductivity of MBG-PCL scaffolds were dramatically enhanced by this precoating process.
Conclusion
The precoating process of ECM components on MBG-PCL scaffold using a high bioactivity of MBG was not only effective in enhancing the functionality of scaffolds but also effective in eliminating the unexpected side effect. The MBG-PCL scaffold-coated ECM components ideally satisfied the required conditions of scaffold in tissue engineering, including 3D well-interconnected pore structures with high porosity, good bioactivity, enhanced cell affinity, biocompatibility, osteoconductivity, and sufficient mechanical properties, and promise excellent potential application in the field of biomaterials.
Introduction
Mesoporous materials synthesized via a sol-gel process and the supramolecular polymer templating methods are expected to have a range of applications in biomaterial science on account of their unique structural properties, including an ordered open pore structure ranging in size from 2 nm to 50 nm as well as a large specific surface area and pore volumes.Citation1–Citation4 Bone tissue regeneration is the latest bioapplication using mesoporous materials.Citation5–Citation21 The large specific surface area and pore volume of mesoporous materials dramatically enhance their bone-forming bioactive behavior and allow them to be loaded with osteogenic agents, thus promoting new bone formation, or with antibiotics for treatment purposes.Citation5,Citation6 The development of mesoporous bioactive glasses (MBGs) has increased the use of mesoporous materials in tissue regeneration based on their superior hydroxy apatite (HA) deposition performance, ie, greater bone-forming bioactivity in vitro, when compared with normal sol-gel-derived BGs.Citation6 However, the meso-sized pores are too small to promote bone tissue regeneration. That is, MBGs are difficult to use alone as bone scaffolds for tissue regeneration at this stage.Citation10 The bone scaffold serves as a template for cell interactions and the formation of the bone extracellular matrix (ECM) for providing structural support to newly formed tissue.Citation22–Citation25 An ideal scaffold, therefore, should have bone-mimicking features such as high porosity with a pore size between 10 μm and 1000 μm with a 3D interconnected pore structure, bioactivity, biodegradability, and biocompatibility. Sufficient mechanical strength and ease of processability are also required to match the intended site of implantation and handling. To compensate for the weak structural properties of MBGs and make them applicable for tissue regeneration, our group proposed and successfully prepared hierarchically 3D porous BG ceramic scaffolds using a combination of sol-gel, polymer templating, and rapid prototyping (RP) techniques, which can generate a physical model directly from computer-aided design data.Citation10 Even though these newly proposed BG scaffolds addressed the structural limitations of MBGs, their mechanical stability was lacking. For this, the development of a 3D porous MBG-polymer composite scaffold was constructed using the biodegradable polymer poly ɛ-caprolactone (PCL).Citation11 The MBG-PCL composite scaffolds possessed largely improved mechanical properties as well as molding capability, and they displayed effective in vitro bone-forming bioactivity. However, there still remain the problems to be solved not only relating to the high bioactivity of MBG accompanied with abrupt initial local pH variations, which may have unexpected negative effects such as cell damage when the scaffold is implanted in vivo, but also relating to the strong hydrophobicity of PCL, which may cause loss of cell recognition signaling.Citation26,Citation27 To address these problems and improve the functionality of MBG as a bone scaffold, we applied the high bioactivity of MBG to coat biomimetic components on the surface of MBG-PCL scaffolds. That is, MBG may induce extraordinarily fast formation of HA on scaffolds in simulated body fluid (SBF), and we used this phenomenon to coat the main components of bone-ECM, HA, and collagen.
HA is a major inorganic component of bone ECM and has good osteoconductivity, high affinity to living cells, and the ability to adsorb proteins.Citation27–Citation29 Meanwhile, the major organic component of bone ECM is collagen, which promotes early cell adhesion and interacts with osteoblasts.Citation28,Citation30 The surface of the scaffolds plays an important role in interacting with host cells of tissue after implantation in situ.Citation31 Surface coating of MBG-PCL scaffolds combined with HA and collagen may provide for better interactions between scaffolds and cells compared with bare MBG-PCL scaffolds, resulting in improved tissue regeneration. Furthermore, the precoating process of ECM components on MBG-PCL scaffolds not only may improve the hydrophilicity of MBG-PCL scaffolds but also may eliminate initial variation in pH.
Materials and methods
Synthesis of MBG and preparation of gel paste for rapid prototyping
MBGs with 3D cubic pore structure were prepared according to our previously reported method.Citation32 In a typical synthesis, 2.88 g of F127 is dissolved in 18.1 mL of EtOH. Stock solutions, which were prepared by mixing 1.36 g of calcium nitrate tetrahydrate, 0.26 mL of triethyl phosphate, 6 mL of tetraethyl orthosilicate, 0.95 mL of HCl (1 M), 7.62 mL of ethyl alcohol, and 2.86 mL of H2O, were added to this solution after stirring them for 1 hour separately and were vigorously stirred together for another 4 hours at 40°C. The reactant solution was transferred to a polystyrene vessel without a cap and aged at 40°C 40% RH for 48 hours without stirring. Gel films were obtained on evaporating the solvent. The obtained gel films were calcined at 600°C for 6 hours in air to remove the template. The MBGs were then ground and sieved. Granules with size below 25 μm were selected. PCL was dissolved in chloroform at 40°C, and the determined amounts of MBG powders (60 wt% of MBG to PCL) were then mixed with this to produce a homogeneous MBG-PCL paste. All chemicals were purchased from Sigma-Aldrich (St Louis, MO) and were used without further purification.
Fabrication of 3D scaffolds
The scaffolds were fabricated by directly extruding the gel paste onto a chilled substrate using a robotic deposition device.Citation11 A gantry robotic deposition apparatus was used with specially altered systems such as an actuator to control the position of the deposition nozzle for the fabrication of the scaffold and a heat-controlled blowing system to maintain the 3D scaffold morphology, followed by the rapid evaporation of the solvent. Three axes of motion control (x-, y-, and z-axis) were provided by the gantry system, and a material delivery assembly composed of a syringe as a reservoir was affixed on the z-axis motion stage. The z-axis motion stage assembly was mounted on a moving gantry to enable the controlled motion of the mounted syringe in three dimensions. The gel paste housed in the syringe was deposited through a cylindrical nozzle (24 G [≈500 μm] is generally used). A linear actuator served to depress the plunger of the syringe at a fixed speed such that the volumetric flow rate could be precisely controlled. The extruding strength and speed were adjusted to 50 μL/minute and 10 mm/second, respectively. The shapes and sizes of the scaffold can be designed at discretion and can be controlled by a computer system.
Coating of ECM components
Collagen (type I [Col I]; Sigma-Aldrich, St Louis, MO) was dissolved in 0.1 M acetic acid (2 mg/mL). Two milliliters of this collagen solution was added to 200 mL of SBF. The SBF contained 142.0 mM Na+, 5 mM K+, 1.5 mM Mg2+, 2.5 mM Ca2+, 147.8 mM Cl−, 4.2 mM HCO3−, 1.0 mM HPO42−, and 0.5 mM SO42−.Citation33 Its chemical composition was similar to that of human plasma. The solution had a pH of 7.4 and was kept at 37°C before use. The MBG-PCL scaffolds were immersed in the mixed solution of the collagen-SBF at 37°C for 24 hours. After soaking, each scaffold was collected from the SBF, rinsed, and air dried.
Characterization
Characterization of structure and cell morphology was carried out by field emission scanning electron microscopy (FE-SEM) (Hitachi-S5500; Hitachi, Tokyo, Japan and JEOL-5800, Tokyo, Japan). Cell morphology was also studied using a fluorescence microscope (BX51; Olympus, Tokyo, Japan) with Meta Morph software (Olympus) after incubating for 6 hours at a density of 3 × 104 cells/well. The compressive modulus was determined using a micro load system (R&B Inc, Daejeon, Korea) at a head speed of 0.5 mm/minute. The porosity was measured by the Hg intrusion method (AutoPore IV 9510; Micromeritics, Norcross, GA). Wettability was observed using the contact angle measurement system (Phoenix-300, Surface Electro Optics, Gyunggido, Korea) after dropping 10 μL of water onto each scaffold. X-ray photoelectron spectroscopy (XPS) (ESCALAB 250; Thermo Scientific, Waltham, MA) was used to study the scaffold surface with ALKα electron source. Protein concentration was measured using a protein assay kit (Coomassie Plus 23236; Thermo Scientific). The absorbance at 595 nm was read using a spectrophotometer (Asys UVM 340; Biochrom, Holliston, MA).
Cell culture
Cell culture experiments were performed using a mouse calvaria-derived preosteoblast MC3T3-E1 subclone 4 cell line obtained from American Type Cell Culture Collection (ATCC, Manassas, VA). Cells were maintained in α-minimum essential medium (Gibco BRL Life Technologies, Grand Island, NY) containing 10% fetal bovine serum (Gibco BRL Life Technologies), 100 U/mL penicillin (Keunhwa Pharmaceutical, Seoul, Korea), and 100 U/mL streptomycin (Donga Pharmaceutical, Seoul, Korea). The cells were cultured under 100% humidity and 5% CO2 at 37°C. The medium was changed every other day and, prior to confluence, cells were passaged using 0.05% trypsin/0.02% EDTA.
Initial cell attachment and cell proliferation
Cell attachment and proliferation were evaluated by a colorimetric assay based on the conversion of a 3-(4,5-dimethylthiazol-2-yl)-2,5-diphenyltetrazolium bromide (MTT, Sigma-Aldrich). MC3T3-E1 was seeded onto a square-shaped scaffold at a density of 1 × 105 cells/mL and cultured for 3 hours, 1 day, 3 days, 5 days, and 7 days. Each scaffold was prewetted in culture medium for at least 3 hours and then placed in a well plate.
Alkaline phosphate activity
Total cellular alkaline phosphate (ALP) activity of the cell lysates was measured in 2-amino-2-methyl-1-propanol buffer, pH 10.3, at 37°C using p-nitrophenyl phosphate as the substrate. The absorbance change at 405 nm was measured using a microplate reader. ALP activity was expressed as nanomoles of p-nitrophenol liberated per microgram of total cellular protein.
Quantitative real-time polymerase chain reaction
Quantitative real-time polymerase chain reaction (PCR) was carried out using the Thermal Cycler Dice TP850 (Takarabio Inc, Shiga, Japan) according to the manufacturer’s protocol. Briefly, 2 μL of cDNA (100 ng), sense and antisense primer solution (1 μL, 0.4 μM), SYBR Premix Ex Taq (12.5 μL, Takarabio Inc), and dH2O (9.5 μL) were mixed together to obtain a final reaction mixture (25 μL) in each reaction tube. Quantitative real-time PCR was carried out with the following primers: RUNX2 (s 5′-TAA GAA GAG CCA GGC AGG TG-3′; as 5′-TGG CAG GTA CGT GTG GTA GT-3′), Col 1 (s 5′-ATC CAA CGA GAT CGA GCT CA-3′; as 5′-GGC CAA TGT CTA GTC CGA AT-3′), ALP (s 5′-CTT GAC TGT GGT TAC TGC TG-3′; as 5′-GAG CGT AAT CTA CCA TGG AG-3′), OP (s 5′-TCA AGT CAG CTG GAT GAA CC-3′; as 5′-CTT GTC CTT GTG GCT GTG AA-3′), and OC (s 5′-TGC TTG TGA CGA GCT ATC AG-3′; as 5′-GTG ACA TCC ATA CTT GCA GG-3′). β-actin (s 5′-tag-3′; as 5′-TGG CAG GTA CGT GTG GTA GT-3′) was used to verify equal amounts of cDNA. The amplification conditions were 10 seconds at 95°C, 40 cycles of 5 seconds at 95°C and 30 seconds at 60°C, 15 seconds at 95°C, 30 seconds at 60°C, and 15 seconds at 95°C. Relative quantification of mRNA expression was performed using the TP850 software.
Statistical analysis
The results were expressed as mean ± standard deviation. Statistical analyses were performed using GraphPad Prism (GraphPad Software Institute, San Diego, CA). Treatment effects were analyzed using one-way analysis of variance. Significance was set at P < 0.05.
Results and discussion
Coating of ECM components on MBG-PCL scaffolds
The synthetic strategy is illustrated in . We preliminarily prepared 3D MBG-PCL scaffolds according to our previously reported method.Citation11 The pore size and the porosity of MBG-PCL scaffolds was about 500 μm and 70%, respectively, with well-interconnected pores in three dimensions. The scaffolds were immersed in SBF containing dilute Col I solution at 37°C for 24 hours to form HA/collagen composite coatings on the scaffolds using high bioactivity of MBG (designated by ECM-coated scaffold). The abrupt initial local pH variations occurred during this stage because of initial release of Ca ions from the MBG. MBGs facilitate the rapid and massive release of Ca ions and subsequently induce high bioactivity.Citation6 Both Ca and P ions play an important role in promoting the formation of an extracellular mineralized matrix.Citation34 However, this property may also induce serious negative effects such as an inflammatory response, due to the sudden change of the pH value.Citation18,Citation29,Citation35,Citation36 The abrupt initial pH variations were largely reduced during the biomimetic coating process and may reduce negative effects from the abrupt pH variation in vivo. The pH value of the solution for ECM-coated scaffolds after 1 day of immersion was around 8, whereas the pH value for MBG-PCL scaffolds was around 9.8 ().
Figure 1 Comparison of pH variation of mesoporous bioactive glass (MBG)-polycarplolactone (PCL) and extracellular matrix (ECM)-coated scaffolds in distilled water (A) and X-ray photoelectron spectra before and after ECM coating (B).
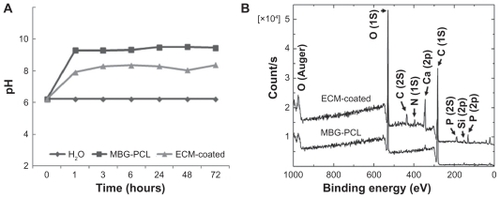
The surface morphology of the MBG-PCL scaffolds started to change after treatment with SBF/collagen solution for 4 hours (see ). The MBG-PCL scaffolds immersed in SBF/collagen solution for 24 hours exhibited a rough surface morphology with fully covered HA-like particles and collagen fibers ( and , and ), whereas uncoated MBG-PCL scaffolds had a smooth surface morphology ( and ). The formation of HA and collagen composites on the surface of the scaffolds was also confirmed by XPS, as shown in . Only weak Ca (345 eV) and Si (152 eV) peaks were detected from MBG-PCL scaffolds, proving the nonexistence of HA before the coating process. The weak peaks suggest that many parts of MBG were masked by PCL during the RP extrusion process, although the scaffolds still exhibited high bioactivity from MBG. Several sharp Ca and P peaks in the XPS spectra of the ECM-coated scaffolds were newly detected, suggesting the formation of HA on the surface of the scaffolds.Citation28 In addition, the detection of an N peak around 397 eV provided evidence of the presence of Col I on the surface of the ECM-coated scaffolds. Interestingly, a larger amount of collagen (5.5 ± 0.7 μg cm−3) adsorbed to the MBG-PCL scaffold by the coprecipitation process of collagen and HA in SBF, compared with the scaffold coated only with single collagen in aqueous condition (3.2 ± 0.2 μg cm−3) under the same conditions such as concentration of collagen and immersing time, possibly due to high interactions between HA and collagen from good protein affinity of HA ().Citation37
Figure 2 Field emission scanning electron microscopy images of mesoporous bioactive glass and polycarplolactone scaffolds before (A) and (B) and after (C) and (D) extracellular matrix component coating. (B) and (D) are the high magnification images of (A) and (B), respectively.
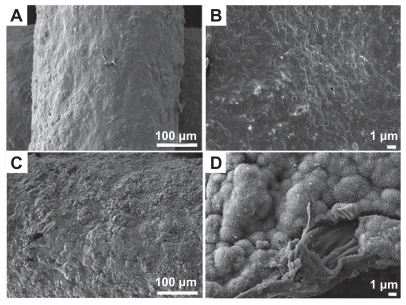
Figure 3 Comparison of the protein adsorption amount.
Note:***P < 0.001.
Abbreviation: ECM, extracellular matrix.

The wettability of the material surface is one of the key parameters affecting the adhesion and spreading of osteoblastic cells and determines subsequent processes such as cell morphology, proliferation, and differentiation.Citation38 Osteogenic cells tend to prefer hydrophilic surfaces rather than hydrophobic surfaces. To test the effects of ECM component coating on the wettability of scaffolds, we prepared scaffolds without pores by extruding paste in succession using RP systems (). The water contact angle of the MBG-PCL scaffolds after applying water droplets for 60 seconds was 74.3°, whereas the water contact angle of PCL was 81.3°. The hydrophilicity of PCL improved by mixing with MBG but remained low due to limited exposure of MBG on the scaffold surface. Meanwhile, the water contact angle of the ECM-coated scaffolds was dramatically changed to 20.1°, suggesting that the hydrophilicity of the scaffolds significantly improved by coating with the ECM components (). The hydrophilic surface of the scaffolds may have been responsible for the increase in cell attachment and osteoconduction. The mechanical properties of the MBG-PCL scaffolds did not decrease during the biomimetic coating process. The compressive moduli of the MBG-PCL and the ECM-coated scaffolds were 17.2 ± 2.4 MPa and 17.1 ± 1.9 MPa, respectively.
Figure 4 Wettability of polycarplolactone (PCL) (A) and mesoporous bioactive glass (MBG)-PCL scaffolds without (B) and with (C) extracellular matrix (ECM) component coating. (D–F) are field emission scanning electron microscopy images of initial cell attachment on PCL (D), MBG-PCL (E), and ECM-coated (F) scaffolds at 0.5 hours after seeding, and (G) and (H) are top view fluorescence microscope images of MBG-PCL (G) and ECM-coated (H) scaffolds after 6 hours of seeding.
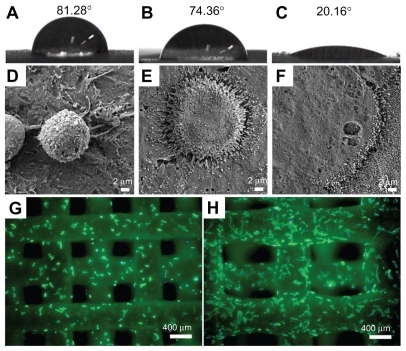
Effect of ECM component coating on the preosteoblast cell behavior
HA generally plays a key role in bone bonding with BG, and HA/collagen promotes the early stage of proliferation, differentiation, and bone mineralization.Citation27,Citation29,Citation39,Citation40 The effects of coating with ECM components on cellular behavior were evaluated in vitro by studying the behavior of mouse calvaria- derived preosteoblast MC3T3-E1 cells. The spreading of cells at the initial stage is an important step for essential biological processes such as proliferation.Citation29 The improved hydrophilicity of the scaffolds greatly affected the enhancement of initial cell affinity. shows the adhesion and spreading morphology of MC3T3-E1 cells on the PCL-, MBG-PCL-, and ECM-coated scaffolds after 30 minutes of incubation. Cells on the PCL scaffolds were spherical in shape without spreading, whereas the appearance of cells on MBG-PCL scaffolds were more spread out with numerous filopodia and lamellipodia. Faster attachment, higher degree of cell extension, and flattened morphology with numerous filopodia and lamellipodia were observed in the cells on ECM-coated scaffolds, proving the high efficiency of ECM coating on initial cell attachment. Similar phenomena can be confirmed by fluorescent microscopic images. shows top view fluorescent microscopic images of cell growth on the MBG-PCL- and ECM-coated scaffolds after 6 hours of incubation, respectively. A higher degree of cell extension and growth was observed in the cells on ECM-coated scaffolds than MBG-PCL, proving the positive efficiency of ECM component coating on cell attachment and growth.
The initial cell attachment efficiency after 3 hours of incubation, evaluating MTT study, proved the favorable effect of ECM coating (). The highest rate of cellular attachment was observed in the ECM-coated scaffolds, whereas the PCL scaffolds showed the lowest rate. There was also a clear difference in the cell proliferation behaviors of scaffolds depending on their surface properties for all testing periods from 1 to 7 days of culture (). The ECM-coated scaffolds displayed the highest increase in the number of cells for all proliferation periods, indicating meaningful effect of the ECM components on the cell proliferation behavior.
Figure 5 Initial MEC3T3-E1 cell attachment at 3 hours of culture (A), proliferation at 1, 3, 5, and 7 days of culture (B), and alkaline phosphate activity at 3, 7, and 14 days of culture (C) on three types of scaffolds expressed as a percentage of cell number.
Notes: Data are presented as the mean ± standard deviation (n = 5 samples per group). Differentiation from ECM-coated scaffolds (*P < 0.05, **P < 0.01, and ***P < 0.001).
Abbreviations: ECM, extracellular matrix; MBG, mesoporous bioactive glass; PCL, polycarplolactone.

The most notable effect of ECM coating was observed on the osteoblast differentiation behavior. The differentiation of cells cultured on PCL-, MBG-PCL-, and ECM-coated scaffolds was assessed in terms of the ALP activity of MC3T3-E1 cells at 3, 7, and 14 days as shown in . It is known that the ALP activity of MC3T3-E1 cells increases after differentiation and then decreases at the beginning of cell mineralization.Citation41 The ALP activity of the ECM-coated sample significantly increased at Day 7 and then decreased, indicating the upregulated osteoblastic differentiation of the cells at Day 7 and the start of cell mineralization at Day 14, whereas the ALP activities of both PCL and MBG-PCL scaffolds slowly increased throughout the entire tested periods. To check the effect of each ECM component on differentiated osteoblast functions, the ALP activity of MC3T3-E1 cells cultured on the MBG-PCL scaffolds coated with HA and collagen was also assessed separately for the same culturing periods (). ALP level in HA-coated scaffolds was largely increased at Day 7, whereas the ALP level in collagen-coated scaffolds was increased at Day 14. Both coating of HA and collagen on MBG-PCL scaffolds is effective in enhancing the differentiation of cells, but HA initiates an earlier stage of differentiation than collagen. Meanwhile, the coating of both HA and collagen at the same time by our coprecipitation method using high bioactivity of MBG could promote the synergistic effect of both components and lead to a much better effect on inducing differentiation of osteoblast cells than the coating of individual components. The differences of ALP activity of MBG-PCL scaffolds with a different ECM component coating condition are mainly caused by the different effect of each component on the cell functions, because all scaffolds were treated using the precoating process under the same conditions, which may reduce abrupt initial pH variation.
Figure 6 ALP activity of MT3T3-E1 cells on scaffolds with different surface conditions at 3, 7, and 14 days of culture.
Note: Data are presented as the mean ± standard deviation (n = 5 samples per group).
Abbreviations: ALP, alkaline phosphate; ECM, extracellular matrix; HA, hydroxy apatite; MBG, mesoporous bioactive glass; PCL, polycarplolactone.
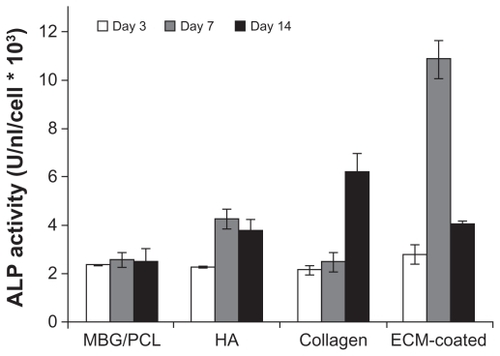
Similar results were obtained from real-time PCR analysis at 4 and 7 days, as shown in . Key osteoblast genes, such as RUNX2, Col I, ALP, osteopontin (OP), and osteocalcine (OC), were selected to evaluate the defect in the differentiation process at mRNA level.Citation42–Citation44 RUNX2 is the master gene in osteogenesis and is the key transcription factor regulating osteoblast differentiation.Citation45 The expression of RUNX2 in all of the samples steadily increased over time. In particular, ECM-coated scaffolds expressed the highest level of RUNX2 from the early stage at Day 4. Col 1 is regularly used as an early marker of osteoblast differentiation. The level of Col 1 in the ECM-coated scaffolds was the highest at Day 4 but downregulated at Day 7 when differentiation started. ALP is another early-stage marker of osteoblast differentiation and function in making phosphate available for calcification. ALP was expressed at the same level in both the MBG-PCL- and ECM-coated scaffolds, whereas it was expressed much lower in the PCL scaffold at Day 4. ALP of the ECM-coated scaffolds was expressed at the highest level after 7 days. OP is a mineral-binding protein found in bone ECM. It associates with biomineralization of ECM into bone and is often used as a middle-stage marker of osteogenic differentiation. The OP level of ECM-coated scaffold was expressed the highest throughout the study and significantly increased at Day 7. OC is secreted by osteoblasts and plays a role in mineralization and calcium ion homeostasis. Consequently, it is often used as a terminal marker of osteoblast differentiation. The highest level of OC was observed in ECM-coated scaffolds at Day 7. The early increased expression of OP and OC in cells grown on ECM-coated scaffolds may be due to the fact that mineralization occurred much sooner. That is, the presence of ECM components may have been the key factor promoting stimulation of the osteogenic differentiation of osteoblast cells. These results also show that our suggested coating process on MBG/PCL scaffolds was effective in enhancing the applicability of MBG/PCL scaffolds to bone tissue regeneration.
Figure 7 Quantitative real-time polymerase chain reaction analysis of RUNX2, collagen-I, ALP, OP, and OC mRNA expression in cells grown on each scaffold at 4 (A) and 7 (B) days of culture.
Note: Data are presented as the mean ± standard deviation (at least n = 3 samples per group). Differentiation from ECM-coated scaffolds (*P < 0.05, **P < 0.01, and ***P < 0.001).
Abbreviations: ALP, alkaline phosphate; ECM, extracellular matrix; MBG, mesoporous bioactive glass; OC, osteocalcine; OP, osteopontin; PCL, polycarplolactone.

The advantage of using an RP technique to produce 3D scaffolds is the construction of a highly porous and well-interconnected 3D structure.Citation46,Citation47 These structural properties allow efficient cell migration, vascularization, and tissue in-growth. The 3D cell movement is essential for 3D tissue in-growth through 3D scaffolds. Meanwhile, the surface properties of 3D scaffolds are also important for promoting sufficient tissue regeneration. The ECM-coated scaffolds display good cell affinity and consequently promote good cell migration as well as penetration within the interior of the 3D scaffolds. shows top view FE-SEM images of cells on the ECM-coated scaffolds after 5 days of incubation. The morphology and distribution of the cells on each strut were similar from the first layer () to the fourth layer (), indicating good cell penetration into ECM-coated scaffolds. shows FE-SEM images of the PCL-, MBG-PCL-, and ECM-coated scaffolds before and after 21 days of cell culture. Although all three scaffolds had the same structure, their cell growth progressed differently. Less tissue sheet formation was observed on the PCL scaffold, especially within the interface of each strut (). The MBG-PCL scaffold showed the formation of denser cell sheets compared with the PCL, and therefore better function as a bone scaffold (). In contrast, there was greater dense cell and tissue sheet formation on the ECM-coated scaffolds, revealing a significant role for ECM components in tissue distribution (). These results suggest that the biomimetic ECM component coating process on MBG/PCL scaffold using a high bioactivity of MBG is effective for enhancing proliferation, osteogenic differentiation, penetration, and growth of cells, and provides high potential possibilities of MBG/PCL scaffolds in the field of bone tissue regeneration.
Figure 8 Field emission scanning electron microscopy images of extracellular matrixcoated scaffolds after 5 days of culture of MC3T3-E1 cell: first (A), second (B), third (C), and fourth layer (D).
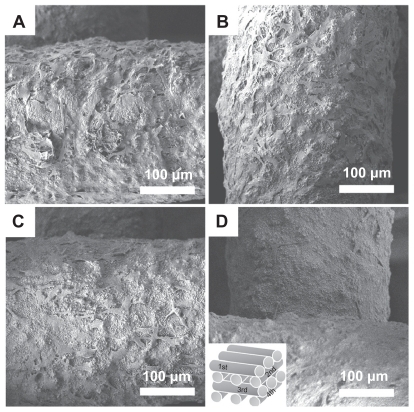
Conclusion
A scaffold must meet several specific requirements to achieve the goal of bone reconstruction. Ideal scaffolds should have several features, including high porosity with 3D interconnected pore structures, bioactivity, biodegradability, biocompatibility, osteoconductivity, and sufficient mechanical properties. Our proposed scaffolds ideally satisfied these conditions. That is, the RP technique provided an easily controllable and highly interconnected 3D pore structure with 70% porosity. MBG supplied high bioactivity, and both MBG and PCL were found to be biodegradable and biocompatible. The combination of MBG and PCL may have provided enhanced mechanical properties compared with the case of using each material alone. ECM component coating using high bioactivity of MBG on the MBG-PCL scaffolds promoted high osteoconductivity. This biomimetic coating process was also helpful in preventing negative effects of local pH variations during the initial ion-leaching. The mesoporosity of MBG should also make possible the controlled release of drugs into scaffolds. We hope that this simple and reproducible process can be adapted for the preparation of various scaffolds for use in tissue regeneration. More detailed studies in vivo are currently in progress.
Supplementary figures
Figure S1 Field emission scanning electron microscopy images of mesoporous bioactive glass and polycarplolactone scaffold surface after immersing in simulated body fluid collagen solution for 4 hours (left) and 24 hours (right).
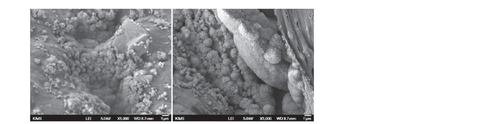
Acknowledgment
This work was supported by the Mid-career Researcher Program through a National Research Foundation of Korea grant funded by the Korean Ministry of Education, Science and Technology (No. 2011-0017572).
Disclosure
The authors report no conflicts of interest in this work.
References
- WanYZhaoDOn the controllable soft-templating approach to mesoporous silicateChem Rev20071072821286017580976
- HartmannHOrdered mesoporous materials for bioadsorption and biocatalysisChem Mater20051745774593
- ColillaMManzanoMVallet-RegíMRecent advances in ceramic implants as drug delivery systems for biomedical applicationInt J Nanomedicine2008340341419337409
- LeeCHLinTSMouCYMesoporous materials for encapsulating enzymesNano Today20094165179
- Vallet-RegíMRuiz-GonzálezLIzquierdo-BarbaIGonzález-CalbetJMRevisiting silica based ordered mesoporous materials: medical applicationsJ Mater Chem2006162631
- YanXYuCZhouXTangJZhaoDHighly ordered mesoporous bioactive glasses with superior in vitro bone-forming bioactivitiesAngew Chem Int Ed Engl2004435980598415547911
- López-NiriegaAArcosDIzquiedo-BarbaISakamotoYTerasakiOVallet-RegiMOrdered mesoporous bioactive glasses for bone tissue regenerationChem Mater20061831373144
- ShiQHWangJFZhangJPFanJStuckyGDRapid-setting mesoporous bioactive glass cements that induce accelerated in vitro apatite formationAdv Mater20061810381042
- OstomelTAShiQTsungCKLiangHStuckyGDSpherical bioactive glass with enhanced rates of hydroxyapatite deposition and hemostatic activitySmall2006111261126517192971
- YunHSKimSEHyeonYTDesign and preparation of bioactive glasses with hierarchical pore networksChem Commun (Camb)20072139214117520115
- YunHSKimSEHyunYTHeoSJShinJWThree-dimensional mesoporous-giantporous inorganic/organic composite scaffolds for tissue engineeringChem Mater20071963636366
- LiXWangXChenHJiangPDongXShiJHierarchically porous bioactive glass scaffolds synthesized with a PUF and P123 cotemplated approachChem Mater20071943224326
- ZhuYWuCRamaswamyYPreparation, characterization and in vitro bioactivity of mesoporous bioactive glasses (MBGs) scaffolds for bone tissue engineeringMicropor Mesopor Mater2008112494503
- ZhuYKaskelSComparision of the in vitro bioactivity and drug release property of mesoporous bioactive glasses (MBGs) and bioactive glasses (BGs) scaffoldsMicropor Mesopor Mater2009118176182
- YunHSKimSEHyunYTPreparation of bioactive glass ceramic beads with hierarchical pore structure using polymer self-assembly techniqueMater Chem Phys2009115670676
- PenaJRománJCabanasMVVallet-RegíMAn alternative technique to shape scaffolds with hierarchical porosity at physiological temperatureActa Biomater201061288129619887122
- WuCZhangYZhuYFriisTXiaoYStructure-property relationships of silk-modified mesoporous bioglass scaffoldsBiomaterials2010313429343820122721
- AlcaideMPortolésPLópez-NoriegaAArcosDVallet-RegíMPortolésMTInteraction of an ordered mesoporous bioactive glass with osteoblasts, fibroblasts and lymphocytes, demonstrating its biocompatibility as a potential bone graft materialActa Biomater2010689289919766743
- YunHSKimSHLeeSSongIHSynthesis of high surface area mesoporous bioactive glass nanospheresMater Lett20106418501853
- YunHSKimSEParkEKBioactive glass-poly (ɛ-caprolactone) composite scaffolds with 3 dimensionally hierarchical pore networksMater Sci Eng C201131198205
- WuCLuoYCunibertiGXiaoYGelinskyMThree-dimensional printing of hierarchical and tough mesoporous bioactive glass scaffolds with controllable pore architecture, excellent mechanical strength and mineralization abilityActa Biomater201172644265021402182
- HutmacherDWScaffolds in tissue engineering bone and cartilageBiomaterials2000212529254311071603
- KarageorgiouVKaplanDPorosity of 3D biomaterial scaffolds and osteogenesisBiomaterials2005265474549115860204
- StevensMMBiomaterials for bone tissue engineeringMater Today2008111825
- HollisterSJScaffold design and manufacturing: from concept to clinicAdv Mater2009213330334220882500
- YunHSParkJWKimSHKimYJJangJHEffect of the pore structure of bioactive glass balls on biocompatibility in vitro and in vivoActa Biomater201172651266021320647
- ChenYMakAFWangMLiJSWongMSIn vitro behavior of osteoblast-like cells on PLLA films with a biomimetic apatite or apatite/collagen composite coatingJ Mater Sci Mater Med2008192261226818058196
- ArafatMTLamCXFEkaputraAKWongSYLiXGibsonIBiomimetic composite coating on rapid prototyped scaffolds for bone tissue engineeringActa Biomater2011780982020849985
- OlmoNMartínAISalinasAJTurnayJVallet-RegíMLizarbeMABioactive sol-gel glasses with and without a hydroxycarbonate apatite layer as substrates for osteoblast cell adhesion and proliferationBiomaterials2003243383339312809766
- LynchMPSteinJLSteinGSLianJBThe influence of type I collagen on the development and maintenance of the osteoblast phenotype in primary and passaged rat calvarial osteoblasts: modification of expression of genes supporting cell growth, adhesion, and extracellular matrix mineralizationExp Cell Res199521635457813631
- BoyanBDHummertTWDeanDDSchwartzZRole of material surfaces in regulating bone and cartilage cell responseBiomaterials1996171371468624390
- YunHSKimSEHyunYTPreparation of highly cubic ordered mesoporous bioactive glassesSolid State Sci20081010831092
- KokuboTTakadamaHHow useful is SBF in predicting in vivo bone bioactivityBiomaterials2007272907291516448693
- GreenleeJrBeckhamTKCreboARMalmorgJCGlass ceramic bone implant. A light microscopic studyJ Biomed Mater Res197162352445027323
- Vitale-BrovaroneCVernéERobiglioLDevelopment of glass-ceramic scaffolds for bone tissue engineering: characterization, proliferation of human osteoblasts and nodule formationActa Biomater2007319920817085090
- LotyCSautierJMOboeufMBioactive glass simulates in vitro osteoblast differentiation and creates a favorable template for bone tissue formationJ Bone Miner Res20011623123911204423
- HenchLLPolakJMThird-generation biomedical materialsScience20022951014101711834817
- Padial-MolinaMGalindo-MorenoPFernández-BarberoJERole of wettability and nanoroughness on interactions between osteoblast and modified silicon surfacesActa Biomater2011777177820807595
- WahlDACzernuszkaJTCollagen-hydroxyapatite composites for hard tissue repairEur Cell Mater200611435616568401
- LiJChenYMakAFTuanRSLiLLiYA one-step method to fabricate PLLA scaffolds with deposition of bioactive hydroxyapatite and collagen using ice-based macroporogensActa Biomater201062013201920004261
- KubokiYKudoAMizunoMKawamuraMTime-dependent changes of collagen cross-links and their precursors in the culture of osteogenic cellsCalcif Tissue Int1992504734801596783
- JellGStevensMMGene activation by bioactive glassesJ Mater Sci Mater Med200617997100217122910
- SetzerBBächleMMetzgerMCKohalRJThe gene-expression and phenotypic response of hFOB 1.19 osteoblasts to surface-modified titanium and zirconiaBiomaterials20093097999019027946
- StangenbergLSchaeferDJBuettnerODifferentiation of osteoblasts in three-dimensional culture in processed cancellous bone matrix: quantitative analysis of gene expression based on real-time reverse transcription-polymerase chain reactionTissue Eng20051185586415998225
- KomoriTRegulation of bone development and extracellular matrix genes by RUNX2Cell Tissue Res201033918919519649655
- HollisterSJPorous scaffold design for tissue engineeringNat Mater2005451852416003400
- LandersRHübnerUSchmelzeisenRMülhauptRRapid prototyping of scaffolds derived from thermoreversible hydrogels and tailored for applications in tissue engineeringBiomaterials2003234437444712322962