Abstract
Background
Although there are a number of reports regarding the toxicity evaluation of inorganic nanoparticles, knowledge on biodegradable nanomaterials, which have always been considered safe, is still limited. For example, the toxicity of chitosan nanoparticles, one of the most widely used drug/gene delivery vehicles, is largely unknown. In the present study, the zebrafish model was used for a safety evaluation of this nanocarrier.
Methods
Chitosan nanoparticles with two particle sizes were prepared by ionic cross-linking of chitosan with sodium tripolyphosphate. Chitosan nanoparticles of different concentrations were incubated with zebrafish embryos, and ZnO nanoparticles were used as the positive control.
Results
Embryo exposure to chitosan nanoparticles and ZnO nanoparticles resulted in a decreased hatching rate and increased mortality, which was concentration-dependent. Chitosan nanoparticles at a size of 200 nm caused malformations, including a bent spine, pericardial edema, and an opaque yolk in zebrafish embryos. Furthermore, embryos exposed to chitosan nanoparticles showed an increased rate of cell death, high expression of reactive oxygen species, as well as overexpression of heat shock protein 70, indicating that chitosan nanoparticles can cause physiological stress in zebrafish. The results also suggest that the toxicity of biodegradable nanocarriers such as chitosan nanoparticles must be addressed, especially considering the in vivo distribution of these nanoscaled particles.
Conclusion
Our results add new insights into the potential toxicity of nanoparticles produced by biodegradable materials, and may help us to understand better the nanotoxicity of drug delivery carriers.
Introduction
With the rapid development of nanotechnology, there is a growing interest in the application of nanomaterials in various fields, such as photonics, catalysis, magnetics, and biotechnology including cosmetics, pharmaceutics, and medicines.Citation1–Citation4 There are several reports regarding the toxicity evaluation of inorganic nanomaterials.Citation5,Citation6 However, little is known about the potential toxicity of nanoparticles used in targeted drug delivery and gene therapies in terms of human health. The risk these nanoparticles may pose to human health must be addressed, because it has been shown that nanoparticles can be administered to the human body by several routes, including inhalation, ingestion, dermal penetration, and injection, followed by distribution of these nanoparticles to various tissues through the systemic circulation.Citation7,Citation8
Until now, various site-specific drug targeting strategies using nanoparticle drug carrier systems have been developed, and nanoparticle-based drug targeting delivery systems have been introduced in the treatment of various diseases.Citation9,Citation10 Chitosan [poly (1,4-β-d-glucopyranosamine)], a natural polysaccharide prepared by N-deacetylation of chitin, has great potential as a biomaterial for the construction of nanosized drug carriers and gene transfer vectors. These carriers have been the focus of considerable attention due to their many advantages, including good stability and simple preparation.Citation9 In addition, chitosan nanoparticles can facilitate drug delivery across cellular barriers and transiently cause opening of the tight junctions between epithelial cells.Citation11 Therefore, the possibility exists that chitosan nanoparticles may translocate from the gastrointestinal tract, nasal cavity, or alveolar sacs into the systemic circulation, thereby causing toxicity to the human body. Several studies addressing the cytotoxicity of chitosan nanoparticles have been reported.Citation12–Citation15 It was shown that chitosan nanoparticles with a particle size of 40 nm elicited dose-dependent inhibitory effects on the proliferation of a tumor cell line, and it appeared that chitosan nanoparticles had much higher cytotoxicity than chitosan.Citation15 Other reports also indicated that chitosan nanoparticles (110–390 nm) had cytotoxicity profiles against A549 cells comparable with those of the corresponding dissolved chitosan materials, with cell viability generally not affected by sample concentrations lower than 740 μg/ mL.Citation12,Citation14 Further, researchers have recently used live human cells to examine the uptake and cytotoxicity of chitosan nanoparticles, and found that they could be internalized by the cells, reducing cell viability and proliferation and compromising cell membrane integrity.Citation13 However, the toxicity profile of chitosan nanoparticles in the body has seldom been investigated. Due to their special physicochemical properties, including a large surface area that can enhance their biological effect, nanoparticles may cause toxicity after entering the body.Citation16 Therefore, evaluation of the potential toxic effects of these nanoparticles in the body is required, given that specific mechanisms and pathways through which nanoparticles may exert their toxic effects remain largely unknown. Until now, there has been little information on the impact of chitosan nanoparticles on human health. Further, systems for evaluation of nanoparticle toxicity are not well established. ZnO nanoparticles were previously evaluated for toxicity using a zebrafish model,Citation5,Citation6 and it was found that these nanoparticles caused toxicity to zebrafish embryos and larvae, reducing the hatching rate and causing pericardial edema. In the present study, we used ZnO nanoparticles as a positive control to evaluate the toxicity of chitosan nanoparticles.
Recently, the zebrafish has become a prominent vertebrate model for assessing the toxicity of drugs and chemicals.Citation17–Citation20 The zebrafish offers a variety of advantages as a desirable model for in vivo high-throughput drug screening. It is low cost and transparent, and its small size allows it to be assayed in 96-well plates. The embryos can develop promptly into larvae within 5 days of fertilization. As a vertebrate, the genomes of zebrafish and humans are closely related.Citation19,Citation21 The zebrafish is also an ideal model for the screening of different nanomaterials and for obtaining nanotoxicity information at the whole animal level, which can then be extrapolated to humans and other vertebrates.Citation6,Citation22 As shown in , nanotoxicity could be evaluated in a zebrafish model, and these toxicities, including a lower hatching rate, mortality, and malformations could be observed.
Figure 1 Toxicity evaluation of nanoparticles using zebrafish model.Citation18
Reprinted from Adv Drug Deliv Rev, vol 61, issue 6, Fako and Furgeson, Zebrafish as a correlative and predictive model for assessing biomaterial nanotoxicity, p. 478–486, Copyright (2009), with permission from Elsevier.
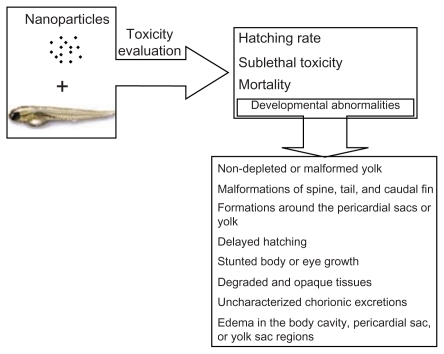
In this study, we prepared chitosan nanoparticles based on ionic gelation of chitosan with tripolyphosphate anions and used the zebrafish embryo as a rapid, medium throughput, cost-effective whole animal model to assess the toxicity of the biodegradable drug/gene carrier, ie, chitosan nanoparticles. Our results demonstrate that chitosan nanoparticles of different particle sizes could cause concentration-dependent toxicity with regard to hatching, growth, and development of zebrafish embryos. Furthermore, it was demonstrated that embryos exposed to chitosan nanoparticles exhibited an increased rate of cell death and enhanced expression of reactive oxygen species, as well as overexpression of heat shock protein 70 (HSP70), indicating that chitosan nanoparticles cause damage to zebrafish embryos. We hope that our discoveries will provide more insight into the potential toxicity of nanoparticles produced by biodegradable materials and will help in understanding better the nanotoxicity of drug delivery carriers.
Materials and methods
Experimental fish
Zebrafish (Danio rerio), 1 year of age and 2–3 cm in length, were kept in recirculating water at 26°C, and fed with commercial pellets at a daily ration of 0.7% of their body weight. All fish were held in the laboratory for at least two weeks prior to the experiments for acclimatization and evaluation of overall fish health. The authors certify that all experiments with live animals were performed in compliance with the relevant laws, and the institutional committees approved our experiments.
Materials
Chitosan (85% deacetylation, molecular weight 105 Da) was purchased from Yuhuan Oceanic Biochemistry (Taizhou, China). ZnO (>98% pure as determined by the manufacturer) with a particle size of 30 nm was purchased for Hangzhou Wan Jing Biotechnology Co Ltd (Hangzhou, China). Bovine serum albumin and a 2′,7′-dichlorodihydrofluorescein diacetate (H2DCF-DA) fluorescent probe was purchased from Sigma-Aldrich Inc (St Louis, MO). Nitrocellulose membrane (Hybond) was obtained from Amersham Pharmacia, Piscataway, NJ. Rabbit antirat HSP70 antibody and horseradish peroxidase-labeled antirabbit IgG were purchased from Wuhan Boshide Company (Wuhan, China). Acridine orange was purchased from Beyotime (Shanghai, China).
Preparation and characterization of nanoparticles
Chitosan solution 2.5 mg/mL was prepared by dissolving the polymer in 1% (v/v) acetic acid aqueous solution for 0.5 hours under magnetic stirring. The pH of the solution was adjusted to 5.0–6.0 using 1 mol/L NaOH. The chitosan solution was then stirred for 0.5 hours at room temperature. Finally, sodium tripolyphosphate (TPP), the counter ion, was dissolved in pure water to prepare a 1 mg/mL solution, and added to the chitosan solution under mild magnetic stirring to form chitosan nanoparticles. The nanoparticle solution was centrifuged at 18,000 rpm and 4°C for 30 minutes, after which the nanoparticles at the bottom were collected, extensively washed with water to remove the TPP and the acetic acid, and finally lyophilized. By adjusting the mass ratio of TPP and chitosan, chitosan nanoparticles of different sizes could be prepared.
ZnO nanoparticles 100 mg/L were prepared in zebrafish culture medium (consisting of 64.75 mg/L NaHCO3, 5.75 mg/L KCl, 123.25 mg/L, MgSO4 · 7H2O, and 294 mg/L CaCl2 · 2H2O), which was prepared according to the International Organization for Standardization, Geneva, Switzerland, standard 7346-3:1996Citation23 as the stock solution.
Morphological examination of the nanoparticles was performed by transmission electron microscopy. The nanoparticles were stained with 2% (w/v) phosphotungstic acid solution for 10 seconds, immobilized on copper grids with formvar, and dried overnight before microscopy. The particle sizes were determined by laser diffraction spectrometry (Malvern Zetasizer 3000HS; Malvern, Worcestershire, UK).
Exposure of zebrafish embryos to nanoparticles
Zebrafish embryos were collected 4–5 hours post-fertilization from the zebrafish aquarium at the Department of Pharmacy, Zhejiang University, and staged according to standard procedures. Citation23 For the toxicity studies, 20 healthy embryos were transferred to the wells of a 24-well plate along with 2 mL of zebrafish culture medium. Different concentrations of chitosan nanoparticles (10, 20, and 40 μg/mL), with particle sizes of 200 nm or 340 nm and dissolved using zebrafish culture medium were added to the wells and incubated for 72 hours at 28.5°C. ZnO nanoparticles 10 mg/L was used as a positive control. Tests were performed in triplicate. The hatching rate, mortality, and malformations of zebrafish embryos at 96 hours post-fertilization were observed under stereomicroscopes (Jiangnan, China) to evaluate the toxicity of the chitosan nanoparticles. The mortality rate was expressed as the number of dead embryos after 96 hours post-fertilization as compared with the control group. The hatching rate was expressed as the number of embryos that had hatched by 96 hours post-fertilization, as compared with the control group. Morphological anomalies, including chorion with attached debris, delayed development, lack of spontaneous movement at 24 hours post-fertilization, pericardial edema, yolk sac edema, bent trunk, tail malformation, and an uninflated swim bladder were observed under stereomicroscopes.
Acridine orange staining
To investigate the role of apoptosis in the toxicity of chitosan nanoparticles, acridine orange staining of nanoparticle- treated or -untreated embryos was performed.Citation24 After exposure to 200 nm chitosan nanoparticles for 96 hours post-fertilization, the embryos were taken out, washed using phosphate- buffered saline, transferred to Eppendorf tubes, and stained with 100 μL acridine orange 5 μg/mL for 20 minutes at room temperature. The embryos were then rinsed quickly in phosphate-buffered saline before being examined under an inverse microscope.
Analysis of intracellular reactive oxygen species in zebrafish embryos
To determine the mechanisms underlying chitosan nanoparticle- mediated toxicity, intracellular reactive oxygen species were examined in zebrafish embryos because these have been implicated in the oxidative stress associated with nanotoxicity.Citation25 Intracellular reactive oxygen species were measured by flow cytometry using a cell-permanent H2DCF-DA fluorescent probe as a reactive oxygen species indicator. The acetates of colorless H2DCF-DA are cleaved by intracellular esterase and converted to fluorescent (dichloro) fluorescein in the presence of reactive oxygen species, which can be detected by flow cytometry. For reactive oxygen species analyses, the larvae were collected at 96 hours post-fertilization and rinsed quickly using culture medium. Homogenation was conducted to prepare a single cell suspension. The cell suspension obtained was incubated with 1.25 mg/L H2DCF-DA for 20 minutes in the dark and at 28.5°C in a fish room. The cells were then washed and resuspended in phosphate-buffered saline. The cells were subsequently analyzed by flow cytometry using a FACScan flow cytometer (Becton Dickinson, San Jose, CA). At least 10,000 events were collected. The analysis was performed using Cell Quest Software (Becton Dickinson).
Western blot analysis
To investigate the developmental toxicity of chitosan nanoparticles and to compare the toxicity caused by ZnO nanoparticles, Western blot analysis was performed to evaluate the protein expression of HSP70 in whole zebrafish larvae. The larvae were collected and rinsed with phosphate-buffered saline, followed by extraction of protein. The same amount of protein extracts from zebrafish larvae were loaded on each lane, electrophoresed and subsequently blotted on a nitrocellulose membrane (Hybond). The membrane was then blocked in Tris-buffered saline containing 2% bovine serum albumin and 0.05% Tween-20 at 4°C overnight and then incubated with rabbit antirat HSP70 antibody (1:2000) at 37°C for 2 hours, followed by staining with horseradish peroxidase-labeled antirabbit IgG. After washing with Tris- buffered saline for three times, the membrane was incubated with a horseradish peroxidase-conjugated secondary antibody and visualized with an enhanced chemiluminescence detection system and X-ray film.
Statistical analysis
Statistical evaluation of differences between experimental group means was done by analyses of variance and Student’s t-tests using Excel software (v 2003; Microsoft, Inc, Redmond, WA), and the results are presented as the mean ± standard error of the mean. A value of P < 0.05 was considered to be statistically significant and P < 0.01 was considered to be very significant. Data points were obtained from at least three independent experiments.
Results
Characterization of nanoparticles
Chitosan nanoparticles with two kinds of particle size were successfully prepared by ionic cross-linking of chitosan with TPP. As shown in , the chitosan nanoparticles maintained a round shape with an almost homogeneous structure, being on average 200 ± 6 nm and 340 ± 10 nm in diameter.
Figure 2 Characterization of 200 nm and 340 nm chitosan nanoparticles. (A) Particle size of chitosan nanoparticles measured by laser diffraction spectrometry, (B) transmission electron micrograph of 200 nm chitosan nanoparticles, (C) particle size of chitosan nanoparticles measured by laser diffraction spectrometry, (D) transmission electron micrograph of 340 nm chitosan nanoparticles.
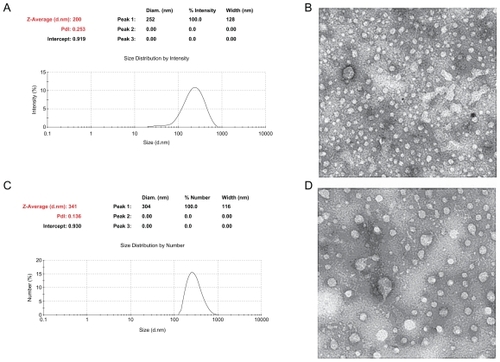
Toxicity of chitosan nanoparticles in zebrafish embryos
To assess the toxicity of the chitosan nanoparticles, the zebrafish embryo model was used to determine whether and how chitosan nanoparticles influence embryonic development. In this study, zebrafish embryos were exposed to chitosan nanoparticles for 96 hours, and dose-dependent inhibition of embryo hatching was induced by chitosan nanoparticles of different particle sizes, as shown in . Compared with the control group, a significant decrease (P < 0.05) in hatching rate was observed at 20 mg/L and 40 mg/L concentrations for the 340 nm chitosan nanoparticles (). For the 200 nm chitosan nanoparticles, as compared with the control group, a significant or very significant decrease in hatching rate was observed for 30 mg/L and 40 mg/L chitosan nanoparticles (200 nm), respectively (). Therefore, at high concentrations, the chitosan nanoparticles had toxic effects on the zebrafish embryos.
Figure 3 Toxicity of chitosan nanoparticles in zebrafish embryo. Hatching rate (%) of zebrafish embryos exposed to (A) 340 nm chitosan nanoparticles or (B) 200 nm chitosan nanoparticles over 96 hours. Mortality of zebrafish embryos at 96 hours post-fertilization following exposure to (C) 340 nm or (D) 200 nm chitosan nanoparticles over 96 hours. Examples of malformation in zebrafish larvae exposed to 5 mg/L 200 nm chitosan nanoparticles. (E) Larva with bent trunk at 96 hours post-fertilization, (F) larva with yolk sac edema at 96 post-fertilization, and (G) control larva at 96 post-fertilization.
Note: *P < 0.05 and **P < 0.01 vs water control.
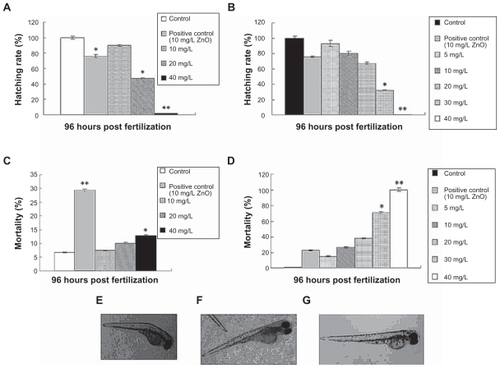
As shown in , a toxic effect of the chitosan nanoparticles was also detected by observation of mortality among zebrafish embryos. Mortality was both dose-dependent and time-dependent (data not shown). As compared with the control group, both 30 mg/L chitosan nanoparticles (200 nm) and 40 mg/L chitosan nanoparticles (340 nm) has significant effects on mortality in zebrafish embryos. All the embryos died after exposure to the 40 mg/L chitosan nanoparticles (200 nm) within 96 hours of exposure. Also, surviving embryos in the 5 mg/L group (200 nm) showed signs of malformation, including a bent spine and an opaque yolk () as compared with controls (). The rate of malformation was increased with increasing chitosan nanoparticle concentration. However, embryos treated with 340 nm chitosan nanoparticles showed no significant malformations when compared with the 200 nm chitosan nanoparticle-treated group. Therefore, 200 nm chitosan nanoparticles had greater toxic effects than 340 nm chitosan nanoparticles on zebrafish embryos.
Chitosan nanoparticle-induced cellular apoptosis
To determine whether in vivo chitosan nanoparticle exposure induced cellular apoptosis in the zebrafish embryo, acridine orange staining was performed to detect cellular death in live embryos. Acridine orange is a nucleic acid-selective metachromatic dye, which emits green fluorescence upon intercalation with DNA and RNA, and is widely used for detecting sites of apoptosis in zebrafish. Acridine orange can permeate apoptotic cells and binds to DNA, whereas normal cells are nonpermeable to acridine orange. Cellular apoptosis was determined using acridine orange staining of larvae exposed to chitosan nanoparticles at 96 hours post- fertilization. In the control group, low natural fluorescence was observed, while a bright green spot could be observed on the larvae exposed to both 20 mg/L and 30 mg/L chitosan nanoparticles (), indicating that these particles could cause cellular apoptosis.
Figure 4 Investigation of the mechanism of toxicity caused by chitosan nanoparticles. (A) Cellular apoptosis was determined using acridine orange staining of chitosan nanoparticle-exposed embryos at 96 post-fertilization. Treated larvae showing bright green spots on the skin indicate the presence of apoptotic cells. Upper panel, control larvae; lower panel, larvae exposed to chitosan nanoparticles 20 mg/L. (B) Flow cytometric analysis of intracellular reactive oxygen species in the control group (left panel) and 5 mg/L chitosan nanoparticle-treated group (right panel). (C) Western blot analysis of HSP70 in zebrafish embryos.
Notes: 1, control group; 2, treated with 10 mg/mL chitosan nanoparticles; 3, treated with 20 mg/mL chitosan nanoparticles; 4, treated with 40 mg/mL chitosan nanoparticles; 5, treated with 5 mg/L ZnO nanoparticles.
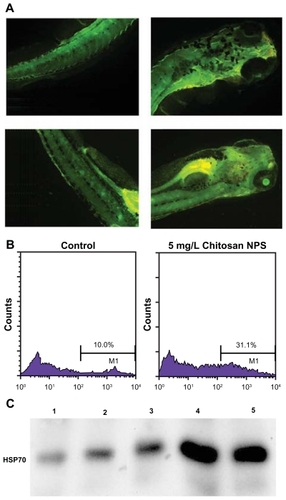
Analysis of intracellular reactive oxygen species in zebrafish embryos
To determine the mechanism underlying chitosan nanoparticle- mediated toxicity, intracellular reactive oxygen species were measured.Citation25 In the present study, we tried to measure and compare intracellular reactive oxygen species in an embryonic cell suspension treated with chitosan nanoparticles. It was shown that the percentages of positive cells in 5 mg/L treated groups (31.1% ± 1.89%) were all significantly higher (P < 0.01) than in the control groups (10.0% ± 0.87%, ). Therefore, an increase in reactive oxygen species was found in embryos exposed to 5 mg/L chitosan nanoparticles, indicating that a higher level of cellular oxidative stress may be caused by treatment with chitosan nanoparticles. This increase in reactive oxygen species may be associated with the observed developmental toxicity of chitosan nanoparticles.
Western blot analysis of HSP70 protein
In order to characterize HSP70 expression, we examined protein expression in zebrafish larvae using Western blot analysis. HSP70 protein in control embryos is detectable. Following 96 hours of exposure to 200 nm chitosan nanoparticles, HSP70 protein levels increased significantly in the larvae (). Elevated HSP70 expression was also evident in the ZnO group, indicating that both 30 nm ZnO nanoparticles and chitosan nanoparticles could be stressful stimuli for zebrafish embryos.
Discussion
Zebrafish embryos have several advantages as an animal model for the study of nanoparticle toxicity. Zebrafish have recently emerged as a model for toxicological study of various nanoparticles. For example, 30 nm ZnO nanoparticles were found to cause dose-dependent toxicity in zebrafish embryos. When the concentrations exceeded 2 mg/L, death of the zebrafish occurred and mortality increased with increasing particle concentration. Furthermore, 100% mortality was observed at a concentration of 30 mg/L,Citation5 and 80 nm copper nanoparticles were found to be acutely toxic to zebrafish, with a 48-hour LC50 concentration of 1.5 mg/L.Citation26 It was also demonstrated that exposure to single-walled carbon nanotubes induces significantly delayed hatching in zebrafish embryos 52–72 hours post-fertilization at concentrations greater than 120 mg/L.Citation27 Although many studies have reported nanoparticle toxicity using the zebrafish model, these embryo toxicity studies were mostly carried out in relation to an evaluation of the toxicity of environmental nanoparticles/pollutants.Citation22,Citation27–Citation29 Relatively few studies have used zebrafish to evaluate the toxicity of nanocarriers, such as biodegradable nanoparticles, which are widely used in the pharmaceutical area. Because these nanomaterials possess unique physical and surface properties, they have inspired plans for a wide spectrum of applications, such as target-specific vehicles for in vivo sensing, diagnosis, and therapy (eg, nanomedicine, drug delivery). However, after entering the body, they may show cytotoxicity and immune toxicity because of their distribution in the body, changes in cellular affinity, and increased cellular uptake.Citation30–Citation32 After systemic administration, these nanoparticles are small enough to penetrate very small capillaries throughout the body, and therefore could be a very effective approach to target certain tissuesCitation33 and may affect the physiology of many cells in the animal body.Citation34
Several reports have shown that nanoparticles can enter the brain and cause tissue injury.Citation4,Citation35 Therefore, evaluation of the toxicity of these nanocarriers is important for human health. Unfortunately, the evaluation of nanoparticle toxicity remains limited. In the present study, zebrafish embryos were explored as an effective model for toxicity evaluation of chitosan nanoparticles. No apparent toxic effects were observed for chitosan nanoparticles at low concentrations. However, death and malformation of zebrafish embryos occurred with increasing chitosan nanoparticle concentrations. Almost 100% mortality was observed at a concentration of 40 mg/L for the 200 nm chitosan nanoparticles. Therefore, the toxicity was dose-dependent and should be considered at high concentrations. Although there was no decrease in hatching rate or mortality at a concentration of 5 mg/L for both 200 nm and 300 nm chitosan nanoparticles, malformation was found at this concentration for 200 nm chitosan nanoparticles, while no malformation was detected for 300 nm chitosan nanoparticles. Therefore, the 200 nm nanoparticles showed higher toxicity than the 300 nm nanoparticles in the zebrafish embryo model. Meanwhile, 200 nm chitosan nanoparticles at a concentration of 5 mg/L were examined for production of reactive oxygen species in zebrafish embryos, and higher levels were detected, which confirms the toxic effect caused by the 5 mg/L nanoparticles.
From the description in a review paper,Citation9 chitosan nanoparticles are a promising system for drug delivery because of their biodegradability, biocompatibility, and good stability. They have been reported to have key applications in various areas including parenteral drug delivery, oral administration of drugs, vaccine delivery, ocular drug delivery, and brain targeting drug delivery.Citation9 The preparation of chitosan nanoparticles was mainly by the ionotropic gelation method. Using this method, the chitosan nanoparticles prepared were mostly around 200–400 nm diameter, which was normally used in the drug delivery system. For example, estradiol-loaded chitosan nanoparticles with an average size of 269.3 ± 31.6 nm were prepared by Wang et al.Citation36 Aktas et al prepared chitosan nanoparticles containing a caspase inhibitor with a particle diameter (Z average) ranging from approximately 313 nm to 412 nm.Citation37 Sadeghi et alCitation38 prepared chitosan-insulin nanoparticles with a diameter of 250 ± 20 nm. It was also reported that the particle size of unloaded chitosan nanoparticles and doxorubicin-loaded nanoparticles was 259 ± 15 nm and 292 ± 42 nm, respectively.Citation39 Recently, we prepared Ganoderma lucidum polysaccharide-loaded chitosan nanoparticles with a mean diameter of 217 ± 6 nm.Citation40 Following on from the aforementioned literature reports, we prepared blank chitosan nanoparticles of two different sizes, ie, 200 ± 6 nm and 340 ± 10 nm, for toxicity evaluation in zebrafish.
The mechanism underlying the toxicity of chitosan nanoparticles was also explored by evaluation of cellular apoptosis, expression of reactive oxygen species, and HSP70. As shown by acridine orange staining, apoptosis could be seen in the embryos after exposure to the nanoparticles. Increased production of intracellular reactive oxygen species was also detected, indicating chitosan nanoparticles caused oxidative stress, as a result of the observed toxicity in zebrafish embryos. We also observed higher expression of HSP70 protein in the nanoparticle-exposed group, indicating stress was caused by the chitosan nanoparticles. Heat shock proteins could be induced by a variety of stimuli, and are potentially damaging to the cell. They are associated with many cellular processes including protein synthesis, folding and translocation, and assembly of larger protein complexes, all of which can be impaired by stress.Citation41 HSP70, one of the major heat shock proteins, is usually expressed at low levels, but increases in response to environmental and physiological stressors.Citation42 HSP70 is conserved throughout evolution and has been shown to protect cells against induction of cell death by a variety of stresses and different modes of cell death.Citation42
Although more and more research is being done using the zebrafish model for drug discovery and drug screening,Citation20,Citation43 few studies have assessed the toxicity of nanocarriers using this model. Here, we first report concentration-dependent toxicity of biodegradable chitosan nanoparticles. However, in evaluation of nanoparticle toxicity, the surface area, surface charge, coating, material solubility, and exposure time are also expected to play important roles, and thus should be carefully considered in future work.
Disclosure
The authors report no conflicts of interest in this work.
Acknowledgments
We thank Cheng Chengzhi, from Zhejiang University, China, for his technical assistance. We thank Yang Zhou from Iowa State University for polishing this article. This work was supported in part by the National Basic Research Program of China (2009CB930300), the 46th China Postdoctoral Grant (20090461389), and the Medical Scientific Research Foundation of Zhejiang Province, China (2010 KYB035).
References
- DonaldsonKResolving the nanoparticles paradoxNanomedicine (Lond)20061222923417716112
- KaganVEBayirHShvedovaAANanomedicine and nanotoxicology: two sides of the same coinNanomedicine20051431331617292104
- LinkovISatterstromFKCoreyLMNanotoxicology and nanomedicine: making hard decisionsNanomedicine20084216717118329962
- MedinaCSantos-MartinezMJRadomskiACorriganOIRadomskiMWNanoparticles: pharmacological and toxicological significanceBr J Pharmacol2007150555255817245366
- XiongDFangTYuLSimaXZhuWEffects of nano-scale TiO2, ZnO and their bulk counterparts on zebrafish: acute toxicity, oxidative stress and oxidative damageSci Total Environ201140981444145221296382
- ZhuXWangJZhangXChangYChenYThe impact of ZnO nanoparticle aggregates on the embryonic development of zebrafish (Danio rerio)Nanotechnology2009201919510319420631
- BurchWMPassage of inhaled particles into the blood circulation in humansCirculation200210620e141e14212427664
- TakenakaSKargERothCPulmonary and systemic distribution of inhaled ultrafine silver particles in ratsEnviron Health Perspect2001109Suppl 454755111544161
- NagpalKSinghSKMishraDNChitosan nanoparticles: a promising system in novel drug deliveryChem Pharm Bull (Tokyo)201058111423143021048331
- AgnihotriSAMallikarjunaNNAminabhaviTMRecent advances on chitosan-based micro- and nanoparticles in drug deliveryJ Control Release2004100152815491807
- DodaneVAmin KhanMMerwinJREffect of chitosan on epithelial permeability and structureInt J Pharm19991821213210332071
- MaZLimLYUptake of chitosan and associated insulin in Caco-2 cell monolayers: a comparison between chitosan molecules and chitosan nanoparticlesPharm Res200320111812181914661926
- LohJWYeohGSaundersMLimLYUptake and cytotoxicity of chitosan nanoparticles in human liver cellsToxicol Appl Pharmacol2010249214815720831879
- HuangMKhorELimLYUptake and cytotoxicity of chitosan molecules and nanoparticles: effects of molecular weight and degree of deacetylationPharm Res200421234435315032318
- QiLXuZJiangXLiYWangMCytotoxic activities of chitosan nanoparticles and copper-loaded nanoparticlesBioorg Med Chem Lett20051551397139915713395
- GovernaMValentinoMVisonaIMonacoFAmatiMImportance of the specific surface area in the study of the biological effects of dusts of industrial useG Ital Med Lav1995171–6105109 Italian8991817
- WangSLiuKWangXHeQChenXToxic effects of celastrol on embryonic development of zebrafish (Danio rerio)Drug Chem Toxicol2011341616520954803
- FakoVEFurgesonDYZebrafish as a correlative and predictive model for assessing biomaterial nanotoxicityAdv Drug Deliv Rev200961647848619389433
- HillAJTeraokaHHeidemanWPetersonREZebrafish as a model vertebrate for investigating chemical toxicityToxicol Sci200586161915703261
- McGrathPLiCQZebrafish: a predictive model for assessing drug-induced toxicityDrug Discov Today2008139–1039440118468556
- YangLHoNYAlshutRZebrafish embryos as models for embryotoxic and teratological effects of chemicalsReprod Toxicol200928224525319406227
- BaiWTianWZhangZEffects of copper nanoparticles on the development of zebrafish embryosJ Nanosci Nanotechnol201010128670867621121381
- International Organization for StandardizationWater quality-determination of the acute lethal toxicity of substances to a freshwater fish [Brachydaniorerio Hamilton–Buchanan (Teleostei, Cyprinidae)]Part 3: Flow-through methodGeneva, SwitzerlandInternational Organization for Standardization1996
- AsharaniPVWuYLGongZYValiyaveettilSToxicity of silver nanoparticles in zebrafish modelsNanotechnology2008192525510221828644
- LiNXiaTNelAEThe role of oxidative stress in ambient particulate matter-induced lung diseases and its implications in the toxicity of engineered nanoparticlesFree Radic Biol Med20084491689169918313407
- GriffittRJWeilRHyndmanKAExposure to copper nanoparticles causes gill injury and acute lethality in zebrafish (Danio rerio)Environ Sci Technol200741238178818618186356
- ChengJPFlahautEChengSHEffect of carbon nanotubes on developing zebrafish (Danio rerio) embryosEnviron Toxicol Chem200726470871617447555
- FentKWeisbrodCJWirth-HellerAPielesUAssessment of uptake and toxicity of fluorescent silica nanoparticles in zebrafish (Danio rerio) early life stagesAquat Toxicol2010100221822820303188
- King-HeidenTCWiecinskiPNManghamANQuantum dot nanotoxicity assessment using the zebrafish embryoEnviron Sci Technol20094351605161119350942
- HuYLGaoJQPotential neurotoxicity of nanoparticlesInt J Pharm20103941–211512120433914
- Maurer-JonesMABantzKCLoveSAMarquisBJHaynesCLToxicity of therapeutic nanoparticlesNanomedicine (Lond)20094221924119193187
- XiaTKovochichMLiongMZinkJINelAECationic polystyrene nanosphere toxicity depends on cell-specific endocytic and mitochondrial injury pathwaysACS Nano200821859619206551
- Braydich-StolleLHussainSSchlagerJJHofmannMCIn vitro cytotoxicity of nanoparticles in mammalian germline stem cellsToxicol Sci200588241241916014736
- BrookingJDavisSSIllumLTransport of nanoparticles across the rat nasal mucosaJ Drug Target20019426727911697030
- SharmaHSNanoneuroscience: emerging concepts on nanoneurotoxicity and nanoneuroprotectionNanomedicine (Lond)20072675375818095842
- WangXChiNTangXPreparation of estradiol chitosan nanoparticles for improving nasal absorption and brain targetingEur J Pharm Biopharm200870373574018684400
- AktasYAndrieuxKAlonsoMJPreparation and in vitro evaluation of chitosan nanoparticles containing a caspase inhibitorInt J Pharm2005298237838315893439
- SadeghiAMDorkooshFAAvadiMRSaadatPRafiee-TehraniMJungingerHEPreparation, characterization and antibacterial activities of chitosan, N-trimethyl chitosan (TMC) and N-diethylmethyl chitosan (DEMC) nanoparticles loaded with insulin using both the ionotropic gelation and polyelectrolyte complexation methodsInt J Pharm20083551–229930618206322
- JanesKAFresneauMPMarazuelaAFabraAAlonsoMJChitosan nanoparticles as delivery systems for doxorubicinJ Control Release2001732–325526711516503
- LiNHuYLHeCXPreparation, characterisation and anti-tumour activity of Ganoderma lucidum polysaccharide nanoparticlesJ Pharm Pharmacol201062113914420723010
- LindquistSCraigEAThe heat-shock proteinsAnnu Rev Genet1988226316772853609
- FuldaSGormanAMHoriOSamaliACellular stress responses: cell survival and cell deathInt J Cell Biol2010201021407420182529
- KariGRodeckUDickerAPZebrafish: an emerging model system for human disease and drug discoveryClin Pharmacol Ther2007821708017495877