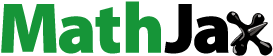
Abstract
Insulin was complexed with sodium deoxycholate to form an insulin-sodium deoxycholate complex (Ins-SD-Comp) using an hydrophobic ion pairing method in aqueous phase to enhance the liposolubility of insulin. In order to obtain the maximal complexation efficiency, the molar ratio of sodium deoxycholate to insulin was found. The zeta potential method was used to confirm the optimal ratio for formation of Ins-SD-Comp. The structural characteristics of Ins-SD-Comp were assessed using the Fourier transform infrared method. The apparent partition coefficient of insulin increased upon the formation of Ins-SD-Comp. Based on the preliminary study, Ins-SD-Comp was encapsulated into poly(lactide-co-glycolide) (PLGA) nanoparticles using an emulsion solvent diffusion method. The maximal encapsulation efficiency of Ins-SD-Comp into PLGA nanoparticles was 93.6% ± 2.81%, drug loading was about 4.8% ± 0.32%, and the mean diameter of the nanoparticles was 278 ± 13 nm. Biological activity and in vivo results revealed that the bioactivity of insulin was not destroyed during the preparation process. Ins-SD-Comp-loaded PLGA nanoparticles have the potential to reduce serum glucose levels and increase the oral bioavailability of insulin.
Introduction
Insulin is a 51-amino acid peptide that was discovered in 1921 by Branting and Best, together with MacLeod and Collip. It is excreted by pancreatic β cells, and has the ability to control glucose levels in the blood by facilitating glucose uptake in the cells of the organism. The most common therapy for diabetes mellitus is injection of insulin, but this is painful and inconvenient, resulting in poor patient compliance.Citation1 In recent decades, oral delivery of insulin has been widely investigated because it can improve patient compliance and transport insulin directly to the liver, which can prevent peripheral hyperinsulinemia.Citation2 In addition, orally delivered insulin undergoes hepatic bypass before entering the circulation, so has the potential to mimic the effects of insulin secreted by the pancreas in terms of inhibition of hepatic gluconeogenesis and hepatic glucose output.Citation3–Citation6
However, there are various obstacles concerning oral administration of insulin, including, but not limited to, poor bioavailability owing to its inherently poor absorption properties, poor absorption from the intestinal lumen due to the tight junctions in the epithelial lining,Citation7 and susceptibility to chemical and physiological degradation.Citation8,Citation9
In order to overcome the obstacles mentioned above and enhance the oral bioavailability of insulin, biodegradable polymeric nanoparticles, such as poly(lactic acid), poly(glycolic acid), and their copolymers, poly(lactide-co-glycolide) (PLGA) nanoparticles, have been widely studied by our group.Citation10,Citation11 During encapsulation of hydrophilic insulin into the hydrophobic copolymer, the hydrophobic ion pairing technique was applied to enhance the hydrophobicity of insulin. It was reported that the hydrophobic ion pairing method can be used to enhance the hydrophobicity of protein/peptides.Citation12–Citation15 This technique is simply performed by the interaction between ionic protein and the opposite ionic head group of fatty acid, surface-active agents or other amphiphilic molecules at suitable pH. In this study, sodium deoxycholate was chosen as the counterpart of insulin. As part of the enterohepatic circulation, sodium deoxycholate is produced in the liver and recirculated 10–20 times per day. It can be reabsorbed from the site of action in the intestinal lumen and returned to the liver via the portal vascular system after being released into the duodenum.Citation16 The selective reabsorption of sodium deoxycholate can potentially be used to improve the bioavailability of insulin. Moreover, it was reported that bile salts can disrupt tight junctions in the epithelial lining, allowing for paracellular and transcellular transport pathways.Citation17,Citation18
In this paper, a more liposoluble insulin-sodium deoxycholate complex (Ins-SD-Comp) was prepared using the hydrophobic ion pairing method. The lyophilized complex was then loaded into PLGA nanoparticles by the emulsion solvent diffusion method. Afterwards, the physicochemical characteristics of the PLGA nanoparticles, bioactivity of insulin in different forms, and hypoglycemic effect of orally administered Ins-SD-Comp PLGA nanoparticles were investigated.
Materials and methods
Materials
Porcine insulin (28.8 IU/mg) and streptozotocin were purchased from Sigma-Aldrich (St Louis, MO). Polyvinyl alcohol (PVA-403) was kindly supplied by Kuraray Co Ltd (Osaka, Japan). PLGA (75/25, molecular weight 20 kDa) and sodium deoxycholate were purchased from Wako Pure Chemical Industries Ltd (Osaka, Japan). Glucose GOD-PAD kits were purchased from Beijing BHKT Clinical Reagent Co Ltd (Beijing, China). All other reagents were of chemical grade.
Animals
Male Wistar rats (weighing 200 ± 20 g and aged 12–13 weeks) were provided by the pharmacological laboratory of Shenyang Pharmaceutical University. The study protocol was reviewed and approved by the Institutional Animal Care and Use Committee, Shenyang Pharmaceutical University, People’s Republic of China.
Induction of diabetes
The rats were induced into diabetes by a tail vein injection of streptozotocin 65 mg/kg in a 10 mM citrate buffer at pH 4.5 as previously described.Citation19 Rats were considered diabetic when glycemia was higher than 16.7 mmol/dL 7 days after streptozotocin treatment.
Preparation of Ins-SD-Comp
Ins-SD-Comp was prepared using the hydrophobic ion pairing method. Briefly, various amounts of sodium deoxycholate 1 mg/mL in purified water were added into 4.5 mg of insulin solution (pH 4.0, 4.0 mL) under magnetic stirring in dropwise manner. The solution immediately became cloudy and the precipitate was treated as the Ins-SD-Comp. The precipitative complex was easily collected by centrifugation at 14,000 rpm for 15 minutes while the native insulin still remained in the supernatant. The resulting complex was washed with distilled water three times and lyophilized into powder. The insulin complexation efficiency (CE [%]) was calculated by measuring the concentration of insulin in the supernatant using a high-pressure liquid chromatography (HPLC) method, and the bound insulin fraction was calculated based on the initial amount of insulin added. The insulin CE (%) was then calculated from the following equation:
where Mi and Mf represent the initial amount of insulin added and the measured amount of free insulin in the supernatant, respectively. The HPLC mobile phase for insulin in this paper was composed of 0.1 mol/L NaH2PO4 aqueous solution, 0.05 mol/L Na2SO4 aqueous solution, and acetonitrile at a ratio of 35:35:30, and the final pH was adjusted to 3.0 with H3PO4 solution. The detector wavelength, the column temperature, and the flow rate of the mobile phase were set at 214 nm, 35°C, and 1 mL/minute, respectively. The injection volume of the test samples was 20 μL. The zeta potentials of Ins-SD-Comp in distilled water were evaluated in order to confirm further the optimal molar ratio of sodium deoxycholate to insulin for the charge-charge interaction, using a Zetasizer Nano-ZS90 (Malvern Instruments, Worcestershire, UK) at 25°C.
Partitioning coefficient of Ins-SD-Comp
Two milliliters of sodium deoxycholate 1 mg/mL solution were added dropwise into 4.5 mg (4.0 mL) of insulin solution. The mixture was centrifuged at 14,000 rpm for 15 minutes to get the complex. Then 2.5 mL of 1-octanol and 2.5 mL of acidified water was added to the complex under magnetic stirring. After being allowed to equilibrate for 3 hours, the mixture was centrifuged for 15 minutes at 14,000 rpm. The concentrations of insulin in the aqueous phase and oil phase were measured by the HPLC method.
FTIR analysis of Ins-SD-Comp
Fourier transform infrared spectrophotometry (FTIR spectrometer, Bruker IFS-55, Switzerland) was used to study the interaction between sodium deoxycholate and insulin. The infrared spectra of insulin, sodium deoxycholate, the Ins- SD-Comp, and the Ins-SD physical mixture were obtained by the KBr method.
Preparation and characterization of Ins-SD-Comp-PLGA nanoparticles
Ins-SD-Comp-PLGA nanoparticles were prepared using the emulsion solvent diffusion method. Based on a preliminary study, 100 mg of PLGA and 5 mg of insulin-equivalent Ins- SD-Comp were dissolved in the organic phase of acetone-ethanol (4:1) mixture with a small quantity of 0.01 N HCl (speeding up solution). The oil phase was then added into 50 mL of aqueous solution containing 1% of PVA at 2 mL/minute using a peristaltic pump while being continuously stirred at 400 rpm with a propeller mixer. The solidified Ins-SD-Comp-PLGA nanoparticles were recovered by ultracentrifugation at 20,000 rpm for 10 minutes at 25°C and then washed with distilled water three times, followed by lyophilization. For the control group, Ins-SD-Comp was replaced by 5 mg of insulin while the other conditions remained unchanged. The concentration of insulin in the supernatant was analyzed by the HPLC method, and the entrapped insulin fraction was calculated based on the initial amount of insulin added. The particle size analysis and zeta potential measurements were performed using photon correlation spectroscopy with a Zetasizer Nano-ZS90 at 25°C.
The morphology of the formulated PLGA nanoparticles was observed by scanning electron microscopy, Jeol JSM 5600 LV (Tokyo, Japan), which required an ion coating with platinum by a sputter coater (JFC-1300, Jeol) for 40 seconds in a vacuum at a current intensity of 40 mA after preparing the sample on metallic studs with double-sided conductive tape.
Cell growth assay
NIH 3T3 cells (1 × 10,000/mL) were suspended in Dulbecco’s Modified Eagle Medium supplemented with 10% fetal bovine serum and 1% penicillin-streptomycin solution and seeded into a 24-well tissue culture plate (Becton Dickinson, Franklin Lakes, NJ). Determined amounts of free insulin, insulin extracted from Ins-SD-Comp, or Ins- SD-Comp-PLGA nanoparticles were added to the cells, and then each culture was incubated for 24 hours in a humidified atmosphere of 5% CO2 in air at 37°C. Cell growth was then assessed by the [3H] thymidine ([methyl-1′-2′-3′H thymidine (1.40 TBq/mmol)], Amersham, UK) incorporation assay. Radioactivity was counted with a scintillation counter (MicroBeta TRILUX, Pharmacia, Sweden).
In vivo bioactivity evaluation
Prior to oral administration, the hypoglycemic effect of insulin in different forms was evaluated in normal rats by the subcutaneous method. Test solutions were prepared as follows:
For the control group, free insulin was dissolved in phosphate-buffered saline (pH 7.4).
For Ins-SD-Comp, the complex was first dissolved in dimethylsulfoxide (DMSO, 10 mg complex versus 0.5 mL DMSO) and then introduced into phosphate-buffered saline (pH 7.4). The theoretical amount of insulin in the complex (TAIC) was calculated by the following formula:
where TAI and TAS represent the total amount of initial insulin and total amount of insulin in supernatant during preparation of the complex, respectively.
For the Ins-SD-Comp-PLGA nanoparticles, the theoretical amount of insulin in the supernatant was measured by the following method. One hundred milligrams of freeze-dried nanoparticles were dissolved in 10 mL of acetonitrile to dissolve the polymer and precipitate the insulin. The samples were centrifuged at 20,000 rpm for 15 minutes and the pellet was dissolved in DMSO (10 mg pellet versus 0.5 mL DMSO), and then diluted with phosphate-buffered saline (pH 7.4). The amount of insulin in the pellet was measured by the HPLC method as mentioned earlier. The theoretical amount of insulin in the supernatant (TAIS, pH 7.4) was calculated by the following formula, where TAP represents the total amount of insulin in 100 mg of PLGA nanoparticles.
All of the three solutions were diluted to an insulin-equivalent concentration of 1 IU/mL (theoretically) with phosphate-buffered saline (pH 7.4) before administration.
The three kinds of solutions were administered subcutaneously to three groups of normal rats (six rats in each group) which had been fasted for 12 hours. Blood samples were collected from the retro-orbital plexus of the rats at baseline and one hour after insulin was administered subcutaneously at 1 IU/kg. Plasma was separated by centrifugation at 4000 rpm for 5 minutes and the glucose level was measured using the glucose oxidase method. The hypoglycemic response to insulin was characterized as follows: the initial plasma glucose (at baseline) was taken as 100% level and the plasma glucose at one hour was calculated as the percentage of the initial value.
Investigation of hypoglycemic effect and relative bioavailability
Twenty-four male rats with streptozotocin-induced diabetes were fasted for 12 hours before administration of the study treatment. During the experiment, the animals had free access to water. The rats were randomized into four groups (six rats in each group). Aqueous free insulin 1 IU/kg was administered subcutaneously in the first group. Ins-SD-Comp-PLGA nanoparticles 20 IU/kg, free insulin 20 IU/kg, and saline were administered orally to the second group, the third group, and the fourth group, respectively. The methods for plasma separation and glucose level determination were the same as in the previous section. The hypoglycemic response to insulin was characterized as follows: the initial plasma glucose was taken as the 100% level and all the following concentration-time data were given accordingly as the percentage of the initial value. The areas above the plasma glucose level-time curves (AAC) were calculated using the trapezoidal rule. The specific value of AAC for the different groups to the AAC of subcutaneous injection group was used to calculate the relative pharmacological bioavailability (RB%) using the following equation:
Results and discussion
Formation of Ins-SD-Comp
It is well known that insulin cannot be directly dissolved in hydrophobic organic solvents due to its hydrophilic nature. However, it is possible to alter its liposolubility using the hydrophobic ion pairing method. Sodium deoxycholate was used because of its good biocompatibility, availability, and well known physiological activity. Further, sodium deoxycholate can enhance the apparent liposolubility of insulin in the form of Ins-SD-Comp. shows that as sodium deoxycholate was slowly added to the insulin solution, the CE (%) increased gradually until reaching a stoichiometric amount of 6:1. This can be explained as follows. Since the isoelectric point is about 5.35 to 5.45, it has a net positive charge of 6 (due to two histidine, one lysine, one arginine, and two N-terminal amino acid residues) at pH 4.0. Therefore, insulin could be complexed completely with sodium deoxycholate (negative charge of 1) under this condition at a molar ratio of 1:6. The CE (%) reached up to 99.65% ± 0.46%. In order to confirm the optimal molar ratio of sodium deoxycholate to insulin, the zeta potential of the complexes was measured. From , it was found that the zeta potential of Ins-SD-Comp changed from +23.64 ± 6.11 through zero to a level of −9.23 ± 3.94 mV as the molar ratio of sodium deoxycholate to insulin changed from 1.85 to 9.23. As expected, the net surface charge on the Ins-SD-Comp was positive because of the surplus positive insulin when the molar ratio of sodium deoxycholate to insulin was below 6:1. As the molar ratio of sodium deoxycholate to insulin got to 6:1, the net surface charge on the Ins-SD-Comp became nearly zero due to the complete complexation of sodium deoxycholate and insulin. When the molar ratio of sodium deoxycholate to insulin was higher than 6:1, the zeta potential became negative, owing to the excess of sodium deoxycholate.
Partitioning coefficient of Ins-SD-Comp
The partitioning coefficient in the 1-octanol/water system was measured in order to confirm the increase in lipophilicity of Ins-SD-Comp. We hypothesized that the insulin and sodium deoxycholate molecules formed a more lipophilic complex, and the aqueous solubility of the complex decreased due to enhanced lipophilicity. The concentrations of insulin and Ins-SD-Comp in the aqueous system were determined to be 0.8922 mg/mL and 0.4638 mg/mL, respectively. Concentrations of insulin and Ins-SD-Comp in 1-octanol were 0.0036 mg/mL and 0.4163 mg/mL, respectively. Partitioning of the complex into 1-octanol increased by two orders of magnitude compared with free insulin. The enhanced lipophilicity of the insulin was definitely due to the hydrophobic counterpart in the complex.Citation20
FTIR analysis of Ins-SD-Comp
FTIR was conducted in order to confirm the complexation behavior between insulin and sodium deoxycholate. We hypothesized that the anionic carboxyl group (COO−) of sodium deoxycholate may interact with the cationic amino group (NH3+) of insulin and thereby form an ionic complex between the two compounds. As a result, some changes would be evident on the absorption bands of carboxyl groups and amide bonds in the infrared spectra.
shows the FTIR spectra of free insulin, sodium deoxycholate, Ins-SD-Comp, and the Ins-SD physical mixture. In the insulin spectrum shown in , four strong bands were observed, ie, 3398.9 (amide A), 1658.3 (amide I), 1516.4 (amide II), and 1240.6 (amide III) cm−1 bands.Citation21 shows three distinctive features at 2938.1, 2864.3, and 1561.2 cm−1, which are infrared absorption bands corresponding to the CH stretching vibration and the COO− stretching vibration modes of sodium deoxycholate.Citation22,Citation23
Figure 3 Infrared spectra of (A) insulin, (B) sodium deoxycholate, (C) the insulin-sodium deoxycholate complex, and (D) the insulin-sodium deoxycholate physical mixture.
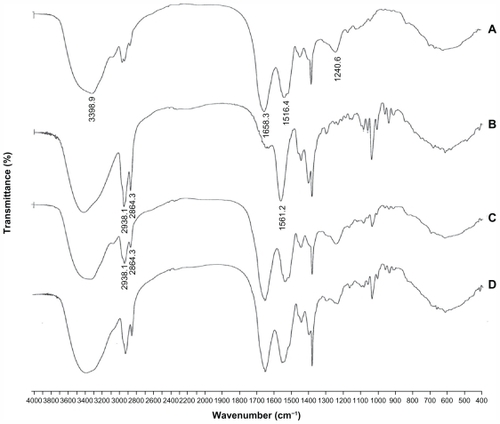
It was found that there was a significant difference between the infrared spectra of the insulin-sodium deoxycholate physical mixture and Ins-SD-Comp. The infrared spectra of the physical mixture () were the simple superposition of the spectra of the two components. However, in the spectrum of Ins-SD-Comp, the stretching band of the carboxylic group at 1564.0 cm−1 disappeared, while infrared absorption bands corresponding to CH stretching vibration at 2938.1 and 2864.3 cm−1 could still be detected. This indicated that the carboxyl group of the anionic sodium deoxycholate interacted with the cationic amino group of insulin and formed an ionic complex between the two compounds.
Preparation and characterization of Ins-SD-Comp-PLGA nanoparticles
As shown in , it was found that the entrapment efficiency and the drug loading of insulin increased in the Ins-SD-Comp form compared with the free insulin form, which can be explained as follows. Due to formation of Ins- SD-Comp, the apparent solubility of insulin in the organic phase increased, so it was much easier to encapsulate the complex into nanoparticles using the emulsion solvent diffusion method. The zeta potential of the Ins-SD-Comp- PLGA nanoparticles was a little higher than that of the insulin-loaded PLGA nanoparticles because of introduction of negative sodium deoxycholate. It is interesting that the particle size of Ins-SD-Comp-PLGA nanoparticles was a little larger than that of the insulin-loaded PLGA nanoparticles because of increasing entrapment of insulin. Further, the nanoparticles prepared by this method were nearly monodispersed and spherical, as shown in .
Table 1 Characteristics of Ins-SD-Comp-PLGA nanoparticles and free insulin PLGA nanoparticles
Biological activity of insulin
It was important to determine whether insulin still maintained its biological activity or not during the preparation process. Insulin acts as a growth factor (IGF), and can control glucose levels. NIH 3T3 mouse fibroblasts have few endogenous insulin receptors and are insensitive to insulin, but express IGF receptors and are responsive to IGF-1.Citation24 Both insulin and IGF regulate certain cellular functions via both overlapping receptor and postreceptor signaling pathways.Citation25 Therefore, we used these cells to test the biological activity of insulin extracted from Ins-SD-Comp and Ins-SD-Comp-PLGA nanoparticles compared with that of normal insulin. In control cells, [3H] thymidine uptake was 9368.7 ± 1231.9 disintegrations per minute (). The radioactivity of cells treated with extracted insulin had similar potency to that of normal insulin. This shows that insulin retained its bioactivity during the preparation process.
Figure 5 Biological activity of normal insulin, insulin extracted from the complex, and insulin extracted from poly(lactide-co-glycolide) nanoparticles.
Note: Data are the mean ± standard deviation, n = 6.
Abbreviations: Ins, insulin; SD, sodium deoxycholate; PLGA, poly(lactide-co-glycolide); NP, nanoparticles.
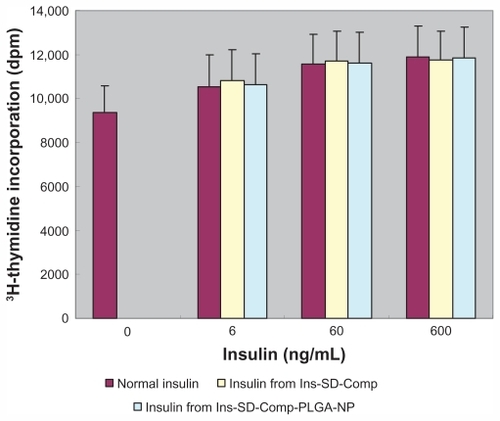
In vivo bioactivity evaluation
In order to confirm further whether insulin retained its bioactivity during the complexation and encapsulation process, the hypoglycemic effect of free insulin, insulin in the complex, and in the PLGA nanoparticles was evaluated by the subcutaneous method. It was reported that the noticeable insulin response to blood sugar appears 45–90 minutes after subcutaneous administration of insulin,Citation26 so plasma glucose levels in the rats were determined to confirm the bioactivity of insulin in different forms at 60 minutes after subcutaneous administration of the various samples.
As shown in , for free insulin, insulin in Ins- SD-Comp, and Ins-SD-Comp-PLGA-NP, subcutaneous administration in rats reduced plasma glucose levels to almost the same percentage for about 58%, 55% and 57%, respectively. However, there was no statistically significant difference between these values. This result clearly indicated that the preparation process did not decrease the bioactivity in vivo.
Table 2 Bioactivity of various insulin samples
Hypoglycemic effect and relative bioavailability studies
The serum glucose versus time curves of Ins-SD-Comp-PLGA nanoparticles are shown in . It can be observed that oral administration of saline and free insulin did not appreciably lower the blood glucose levels of the animals, demonstrating no insulin absorption. Conversely, oral administration of Ins-SD-Comp-PLGA nanoparticles produced significant plasma glucose reduction (percentage relative to the initial value). A t-test with a significance level of P < 0.01 showed that there was a significant difference between the nanoparticles and the control group. This phenomenon illustrates that the Ins-SD-Comp-PLGA nanoparticles had an effective hypoglycemic effect. The relative bioavailability was about 11.7%, while the relative bioavailability of free insulin was less than 0.5%. The increased absorption of insulin could be due to several factors. Firstly, sodium deoxycholate is a biological compound that consists of an amphiphilic steroid nucleus with a hydrophobic α-side and a hydrophilic β-side. It is synthesized in the liver and carried via the bile duct to the small intestine, and returned to the liver by absorption through the bile acid transporter.Citation27 Therefore, cycling of insulin in the enterohepatic circulation due to the presence of sodium deoxycholate could provide sustained release into the bloodstream. Secondly, due to the formation of Ins- SD-Comp, the apparent partitioning coefficient of insulin increased, which led to increased absorption of insulin. Furthermore, when encapsulated into PLGA nanoparticles, insulin can be partly protected from degradation by acid and enzymes in the gastrointestinal tract. In addition, the particle size of the complex was about 270 nm. Thus the complex may be taken up via Peyer’s patches located in the mucosa of the small intestine before insulin is degraded.Citation28 The absorption mechanism will be studied further in our future work.
Figure 6 Changes (%) of plasma glucose level with time (hours) after administration of various samples.
Notes: Data represents the mean ± standard deviation, n = 6 per group (■) subcutaneous injection of 1 IU/kg insulin solution; oral administration of (♦) insulin-sodium deoxycholate complex poly(lactide-co-glycolide) nanoparticles (20 IU/kg), (▴) free insulin (20 IU/kg) and (▵) saline.
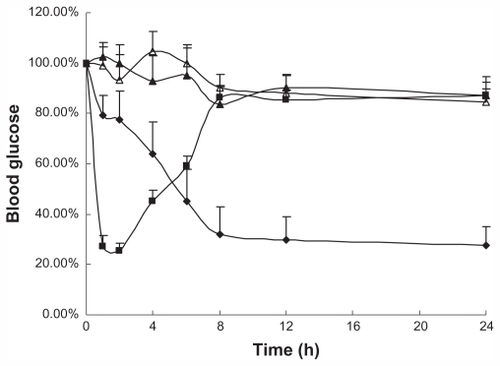
During our studies, fasting plasma glucose levels in the Ins-SD-Comp-PLGA nanoparticle group did not return to the initial level within 24 hours, probably as a result of reduced responses of counterregulatory hormones, such as glucagon, growth hormone, and cortisol.Citation29 This problem has also been encountered in other studies.Citation30,Citation31
Conclusion
In this paper, hydrophilic insulin was hydrophobically modified with sodium deoxycholate and further encapsulated into PLGA nanoparticles, with high entrapment efficiency and high drug loading. The potential of Ins-SD-Comp-PLGA nanoparticles to improve the oral bioavailability of insulin was demonstrated, indicating that it is a promising candidate for oral insulin delivery.
Disclosure
The authors report no conflicts of interest in this work.
References
- PillaiOPanchagnulaRInsulin therapies – past, present and futureDrug Discov Today20016201056106111590034
- TrehanAAliARecent approaches in insulin deliveryDrug Dev Ind Pharm19982475895979876502
- LewisGFZinmanBGroenewoudYVranicMGiaccaAHepatic glucose production is regulated both by direct hepatic and extrahepatic effects of insulin in humansDiabetes19964544544628603767
- EatonRPAllenRCSchadeDSStandeferJC“Normal” insulin secretion: the goal of artificial insulin delivery systems?Diabetes Care1980322702736993139
- DamgéCMaincentPUbrichNOral delivery of insulin associated to polymeric nanoparticles in diabetic ratsJ Control Release2007117216317017141909
- ClementSStillJGKosuticGMcAllisterRGOral insulin product hexyl-insulin monoconjugate 2 (HIM2) in type 1 diabetes mellitus: the glucose stabilization effects of HIM2Diabetes Technol Ther20024445946612396740
- CarinoGPMathiowitzEOral insulin deliveryAdv Drug Deliv Rev1999352–324925710837700
- OwensDRZinmanBBolliGAlternative routes of insulin deliveryDiabet Med2003201188689814632713
- JintapattanakitAJunyaprasertVBMaoSSitterbergJBakowskyUKisselTPeroral delivery of insulin using chitosan derivatives: A comparative study of polyelectrolyte nanocomplexes and nanoparticlesInt J Pharm20073421–224024917597316
- SunSLiangNPiaoHYamamotoHKawashimaYCuiFInsulin-S.O (sodium oleate) complex-loaded PLGA nanoparticles: Formulation, characterization and in vivo evaluationJ Microencapsul201027647147820113168
- CuiFDTaoAJCunDMZhangLQShiKPreparation of insulin loaded PLGA-Hp55 nanoparticles for oral deliveryJ Pharm Sci200796242142717051590
- AdjeiARaoSGarrenJMenonGVadnereMEffect of ion-pairing on 1-octanol-water partitioning of peptide drugs. I: The nonapeptide leuprolide acetateInt J Pharm1993902141149
- MeyerJDManningMCHydrophobic ion pairing: altering the solubility properties of biomoleculesPharm Res19981521881939523302
- SunSCuiFKawashimaYA novel insulin-sodium oleate complex for oral administration: preparation, characterization and in vivo evaluationJ Drug Deliv Sci Technol2008184239243
- MatsuuraJPowersMEManningMCShefterEStructure and stability of insulin dissolved in 1-octanolJ Am Chem Soc1993115412611264
- BaharRJStolzABile acid transportGastroenterol Clin North Am1999281275810198777
- LindmarkTKimuraYArturssonPAbsorption enhancement through intracellular regulation of tight junction permeability by medium chain fatty acids in Caco-2 cellsJ Pharmacol Exp Ther199828413623699435199
- SakaiMImaiTOhtakeHAzumaHOtagiriMEffects of absorption enhancers on the transport of model compounds in Caco-2 cell monolayers: Assessment by confocal laser scanning microscopyJ Pharm Sci19978677797859232516
- DamgéCMichelCAprahamianMCouvreurPNew approach for oral administration of insulin with polyalkylcyanoacrylate nanocapsules as drug carrierDiabetes19883722462513292320
- DaiW-GDongLCCharacterization of physiochemical and biological properties of an insulin/lauryl sulfate complex formed by hydrophobic ion pairingInt J Pharm20073361586617174492
- DorkooshFACoos VerhoefJAmbagtsMHCRafiee-TehraniMBorchardGJungingerHEPeroral delivery systems based on superporous hydrogel polymers: release characteristics for the peptide drugs buserelin, octreotide and insulinEur J Pharm Sci200215543343912036720
- YangPWMantschHHThe critical micellization temperature and its dependence on the position and geometry of the double bond in a series of sodium octadecenoatesJ Colloid Interface Sci19861131218224
- YangLXuYSuYFT-IR spectroscopic study on the variations of molecular structures of some carboxyl acids induced by free electron laserSpectrochim Acta A2005624–512091215
- TakenagaMYamaguchiYKitagawaAOptimum formulation for sustained-release insulinInt J Pharm20042711–2859415129976
- HofmannCGoldfineIDWhittakerJThe metabolic and mitogenic effects of both insulin and insulin-like growth factor are enhanced by transfection of insulin receptors into NIH3T3 fibroblastsJ Biol Chem198926415860686112656686
- EnerothGAhlundKBiological assay of insulin by blood sugar determination in miceActa Pharm Suec1968565915945732300
- MaternSGerokWPathophysiology of the enterohepatic circulation of bile acidsRev Physiol Biochem Pharmacol197985125204373057
- DesaiMPLabhasetwarVAmidonGLLevyRJGastrointestinal uptake of biodegradable microparticles: effect of particle sizePharm Res19961312183818458987081
- PaquotNScheenAJFranchimontPLefebvrePJThe intra-nasal administration of insulin induces significant hypoglycaemia and classical counterregulatory hormonal responses in normal manDiabetes Metab19881413136
- SuzukiAMorishitaMKajitaMEnhanced colonic and rectal absorption of insulin using a multiple emulsion containing eicosapentaenoic acid and docosahexaenoic acidJ Pharm Sci19988710119612029758676
- PanyamJDaliMMSahooSKPolymer degradation and in vitro release of a model protein from poly(D,L-lactide-co-glycolide) nano- and microparticlesJ Control Release2003921–217318714499195