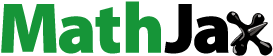
Abstract
Background
Cationic copolymers consisting of polycations linked to nonionic amphiphilic block polymers have been evaluated as nonviral gene delivery systems, and a large number of different polymers and copolymers of linear, branched, and dendrimeric architectures have been tested in terms of their suitability and efficacy for in vitro and in vivo transfection. However, the discovery of new potent materials still largely relies on empiric approaches rather than a rational design. The authors investigated the relationship between the polymers’ structures and their biological performance, including DNA compaction, toxicity, transfection efficiency, and the effect of cellular uptake.
Methods
This article reports the synthesis and characterization of a series of cationic copolymers obtained by grafting polyethyleneimine with nonionic amphiphilic surfactant polyether-Pluronic® consisting of hydrophilic ethylene oxide and hydrophobic propylene oxide blocks. Transgene expression, cytotoxicity, localization of plasmids, and cellular uptake of these copolymers were evaluated following in vitro transfection of HeLa cell lines with various individual components of the copolymers.
Results
Pluronics can exhibit biological activity including effects on enhancing DNA cellular uptake, nuclear translocation, and gene expression. The Pluronics with a higher hydrophilic-lipophilic balance value lead to homogeneous distribution in the cytoplasm; those with a lower hydrophilic-lipophilic balance value prefer to localize in the nucleus.
Conclusion
This Pluronic-polyethyleneimine system may be worth exploring as components in the cationic copolymers as the DNA or small interfering RNA/microRNA delivery system in the near future.
Introduction
Gene therapy has become a promising strategy for the treatment of many inheritable or acquired diseases that are currently considered incurable. Because of their structural diversity, easy production, nonimmunogenicity, and safety, delivery of nucleic acids into cells using cationic polymers has recently attracted remarkable interest in the field of nonviral gene therapy.Citation1,Citation2 Polycation polyethylenimine (PEI) is one of the most effective and widely studied synthetic nonviral gene delivery vectors, and it has been employed for designing DNA delivery vehicles.Citation3,Citation4 Through the addition of cationic polymers to DNA, the condensed complexes are spontaneously formed through electrostatic interactions between the positively charged groups of the polycation and the negatively charged phosphate groups of the DNA, resulting in efficient transport of intact DNA into the nucleus.Citation5–Citation7 The whole gene delivery using PEI involves condensation of DNA into compact particles, uptake into cells, release from the endosomal compartment into the cytoplasm, and uptake of the DNA into the nucleus. The high transfection efficiency of PEI/DNA complexes has been ascribed to the capacity of PEI to buffer endosomes, which protects DNA from nuclease degradation and facilitates endosomal escape of PEI/DNA complexes (the “proton sponge” effect).Citation8,Citation9 However, polyplexes of PEI/DNA have shown low colloidal stability and considerable toxicity in vivo.Citation10 Meanwhile, as both the transfection efficiency and cytotoxicity seem to depend on such physicochemical properties as molecular weight and branching ratio, it becomes evident that polymer structure significantly influences the efficacy of PEI-based vectors.Citation9,Citation11 Therefore, the discovery of new potent materials still largely relies on empiric approaches rather than a rational design.
Pluronic® block copolymers, which consist of hydrophilic ethylene oxide (EO) and hydrophobic propylene oxide (PO) blocks that are arranged in a basic A-B-A structure (EOx-POy-EOx), are amphiphilic molecules that can be used as structural elements of the polycation-based gene delivery system (polyplexes).Citation4,Citation6 The number of hydrophilic EO (x) and hydrophobic PO (y) units can be altered, and the retention of the block copolymer in the organs increases as the length of the hydrophobic PO block increases, or as the hydrophilic-lipophilic balance (HLB) value decreases. Kabanov and other researchersCitation12–Citation14 reported that Pluronic could enhance cell interactions, DNA transport, and transgene expression, and that Pluronics possess the unique ability to incorporate themselves into cell membranes in the presence of the hydrophobic poly(propylene oxide) chain. In contrast, the hydrophilic EO chain does not interact with lipid membranes but it could be used to prevent the binding of other polymers with the membranes.Citation4 The system is stabilized in dispersion by the EO corona in a manner similar to regular Pluronic micelles. To design an effective gene delivery vector, many efforts have been made to improve the transfection efficiency and to reduce the cytotoxicity. Optimal efficacy has been observed with Pluronic copolymers, which have intermediate lengths of PO chains and relatively short EO segments.Citation15 The micelles of these copolymers are sufficiently potent in the ability of binding cellular membranes and a relatively high concentration of micelles could be reached in solution.Citation16
Based on previous studies, the authors prepared a series of cationic copolymers by grafting low-molecular-weight PEI with Pluronics consisting of different ratios of EO and PO. These copolymers were then evaluated as delivery systems for the plasmid DNA in vitro. The authors further investigated the structure–cellular uptake relationship of low-molecular-weight PEI modified by Pluronic with different EO/PO ratios and an HLB that could reduce the cytotoxicity of PEI and accelerate endocytosis of the polyplexes. This study was conducted to formulate a nonviral system for gene delivery and to clarify the relationship between the structure and the function of intracellular trafficking.
Materials and methods
Materials
Branched PEI (molecular weight, 2000) was obtained from Sigma-Aldrich (St Louis, MO). The BASF Corporation (Mount Olive, NJ) kindly provided the Pluronics (F68, P123, P105, L61). Bis-(trichloromethyl) carbonate, N-hydroxysuccinimide, triethylamine, acryloyl chloride, anhydrous dichloromethane, and dithiothreitol (DTT) were purchased from Sinopharm Chemical Reagent Co, Ltd (Shanghai, China) and were used without further purification. A luciferase assay system for physicochemical characterization study and in vitro transfection assay and pGL-3 control vector with SC-40 promoter and enhancer encoding firefly (Photinus pyralis) luciferase were obtained from Promega (Madison, WI). The Institute of Life Science and Technology of Tongji University in Shanghai, China, kindly provided the quantum dots (QDs).
Synthesis of activated Pluronic
The Pluronic (P123, P105, F68, L61, 0.6 mmol) was dried by co-evaporation with anhydrous toluene under vacuum at 50°C, dissolved in a toluene/dichloromethane mixture (3:1, 40 mL), and treated with bis-(trichloromethyl)-carbonate (1.2 mmol) overnight. The solution was evaporated to dryness under vacuum. The residue was redissolved in a toluene/ dichloromethane mixture (2:1, 30 mL) and was treated with solid N-hydroxysuccinimide (2.0 mmol) followed by anhydrous triethylamine (0.28 mL, 2.0 mmol). After 4 hours of stirring, the solution was filtered and evaporated to dryness. The product was purified on a silica gel column using stepwise elution with trichloromethane methanol (10%–15% v/v) mixtures; the yield was about 80%–85%.
Synthesis of polymer particle copolymers
The PEI (0.10 mmol) was dissolved in 10 mL of anhydrous dichloromethane as solution A, and the predetermined amount of activated Pluronic (0.01 mmol) was dissolved in 10 mL of anhydrous ethanol as solution B. Solutions A and B were then slowly added to a base solution of anhydrous dichloromethane (10 mL) with constant stirring. The reaction mixture was left overnight at room temperature. After completion of reaction, the conjugate was dialyzed against distilled water at 4°C for 2 days using a Spectra/Por® membrane (Carl Roth GmbH & Co. KG Karlsruhe, Germany) (molecular weight cut-off, 7000) and was lyophilized.
Characterizations of polymer particles (nuclear magnetic resonance/gel permeation chromatography)
The ratio of Pluronic and PEI in the copolymer samples was determined from proton nuclear magnetic resonance (1H-NMR) spectra (Varian, Palo Alto, CA 300 MHz) using integral values obtained for the –CH2CH2O– protons of Pluronic and the –CH2NH– protons of PEI. The 1H-NMR analysis was carried out with a 10 mg sample of polymer particles (PPs) dissolved in 0.6 mL of deuterium oxide at room temperature.
Molecular weight and molecular weight distribution of the polymers were determined by gel permeation chromatography with multi-angle laser light scattering (Shimadzu LC-20 AD; Shimadzu, Kyoto, Japan) (laser wavelength, 690 nm), using a TSK-GEL G5000PWXL column (Tosoh, Japan) (temperature, 40°C) operated at a flow rate of 0.4 mL/minute. Ammonium acetate (0.2 mol/L) was used as a mobile phase.
Degradation of PPs
Degradation of the branched PPs was estimated through the measurement of molecular weight. Briefly, 0.5 g of each polymer was dissolved in 10 mL of phosphate-buffered saline (PBS) (Gibco, Invitrogen, Carlsbad, CA) (0.1 mol/L, pH 7.4) and then incubated at 37°C with shaking at 100 rpm for specific time points. After incubation, solutions of polymers were lyophilized and the molecular weight of the lyophilized samples was measured by gel permeation chromatography with multi-angle laser light scattering (laser wavelength, 690 nm).
Preparation of PP/DNA complexes
The charge ratio of the PP/DNA complexes was expressed as the ratio of PP weight to DNA weight (w/w). Complexes were induced to self-assemble by mixing plasmid DNA with appropriate polymer solution (PBS 0.1 mol/L, pH 7.4) at the desired charge ratio. The complexes were allowed to stand at room temperature for 30 minutes.
Agarose gel retardation assay
Various amounts of Pluronic-g-PEI dissolved in deionized water were added into the aqueous solution with a fixed amount of plasmid DNA (50 ng/well) and incubated for 30 minutes at room temperature. The mixture was electrophoresed on a 0.7% (weight/volume) agarose gel for about 30 minutes at 100 V in the Tris-acetate-ethylenediaminetetraacetic acid buffer (Gibco, Invitrogen, Carlsbad, CA). The gel was stained with ethidium bromide (0.5 g/mL) and was illuminated on an ultraviolet illuminator (302 nm) to show the location of the DNA and various levels of polyplex formation.
Measurement of particle size and zeta potential
The size and zeta potential of cationic polymer/DNA complexes in PBS buffer at room temperature were measured using an electrophoretic light-scattering spectrophotometer (Zetasizer Nano ZS90, Malvern Instruments, Worcestershire, United Kingdom), with scattering angles of 90°. The polyplexes were prepared by adding an appropriate amount of cationic polymer solution based upon desired w/w ratios to DNA (20 μg) in 250 μL of PBS while vortexing. The complexes were incubated at room temperature for 30 minutes before measurement of size and zeta potential was carried out, and all the experiments were performed in triplicate.
DNA protection and release assay
Each complex solution with PPs to DNA at a weight ratio of 20:1 was divided into equal triplicates. One served as the control and the other two were incubated with DNase I at 37°C for 2 hours at a final DNase I concentration of 50 U/g of plasmid DNA. Ethylenediaminetetraacetic acid (3 μL, 0.5 mol/L) was added to one of these two solutions to immediately stop DNA degradation, and then 3 μL of sodium dodecyl sulfate (10%, w/v) was added to displace DNA. Naked DNA, with and without DNase I treatment, served as the control. All samples were then placed in an ice bath. Finally, 0.7% agarose gel electrophoresis was performed to evaluate the integrity of DNA in the complexes.
Then 10 μL of PBS (as control) or of PBS containing DTT was added to give a final concentration of DTT 10 mmol/L in the resultant solution, and the dispersions were incubated for 60 minutes. The samples were then analyzed by gel electrophoresis, as described.
Cytotoxicity assay
Cell toxicity of PP/DNA complexes was investigated by cell proliferation assay. HeLa cells were seeded in 96-well plates at an initial density of 1 × 104 cells/well and were incubated for 18–20 hours to reach 80% confluency at treatment. The culture medium was then replaced with fresh serum-free media containing serial dilutions of PP/DNA or PEI complexes at various concentrations (4, 6, 8, 16, 24, and 32 mg/mL). After 24 hours of incubation, 20 μL of CellTiter 96® AQueous One Solution Reagent (Promega) was added to each well and the cells were further incubated for 1–4 hours. The absorbance was then measured at 490 nm using an enzyme-linked immunosorbent assay plate reader (Model 318MC, Sanco, Shanghai, China) to obtain the metabolic activity of the cells. The spectrophotometer was calibrated to 0 absorbance using culture medium without cells. The cell viability was calculated according to the following equation:
where Atest is the absorbance of the PPs or PEI-treated cells and Acontrol is the absorbance of the untreated cells.
Luciferase assay in vitro
HeLa cells were seeded in a 24-well plate at a density of 1 × 105 cells per well and were incubated for 18–24 hours to 60%–70% confluency. Then 3 μg of pGL3 was formulated with the different polymers at various w/w ratios and incubated for 30 minutes at room temperature. Serum-free Dulbecco’s modified Eagle’s medium (DMEM) (Gibco, Invitrogen, Carlsbad, CA) was added to the complex-dispersed medium. The polymer/ DNA complexes dispersed in serum-free DMEM were added to the 24-well plate and incubated for 5 hours at 37°C under a 5% CO2 atmosphere. The medium was then replaced with growth medium containing serum and was incubated for 48 hours. The luciferase assay was carried out according to the manufacturer’s instructions (Promega). The relative light units per second determined in 20 μL of cell extract were converted into the amount of luciferase (pg) using a luciferase standard curve. The standard curve was obtained by diluting known amounts of recombinant luciferase (E1701; Promega) in lysis buffer used to extract cells. The amount of cell protein in 50 μL of cell extract was determined using a Micro-BCA protein assay kit (Pierce, Rockford, IL). The transfection efficiency was expressed as relative light units per milligram of cell protein. All transfection experiments were performed in triplicate.
Cellular uptake study
Green QDs were used as molecular probes to label F68-, P123-, P105-, and L61-PEI 2KD. Cells were seeded on glass coverslips and were then transfected with the PP/DNA complexes (10:1, w/w), which had been incubated for 1, 3, 5, 10, 20, and 30 minutes in serum-free DMEM at 37°C before addition into the cell culture. At the end of each time point, cells were washed, fixed (using 4% paraformaldehyde), and examined under a confocal microscope (FluoView® FV1000; Olympus, Tokyo, Japan).
Statistical analysis
The data are presented as the mean plus or minus the standard deviation. Statistically significant differences were determined using two-sample Student’s t-tests and analysis of variance, with P < 0.05 as the level of statistical significance.
Results and discussion
Synthesis and characterization of PP copolymers
Cationic copolymers were synthesized by grafting polyether chains, Pluronic, to the amino groups of PEI. The authors chose four different types of Pluronic, and the HLB order was F68 > P105 > P123 > L61 ().Citation17 In all cases, free hydroxyl groups of the Pluronic polyether chains were activated by succinimidyl carbonate in advance and were then linked to the amino groups of PEI, as shown in .Citation18 The copolymers were separated from unconjugated polymers by dialysis, and then the preparation of PP copolymers was determined from 1H-NMR spectra using integral values obtained for the –CH2CH2O– protons (Pluronic F68) and the –CH2CH2NH– protons (PEI). shows the 1H-NMR spectra of Pluronic-g-PEI in deuterium oxide, where –CH2CH2O– proton peaks appear at δ3.8 ppm and –CH2CH2NH– proton peaks appear at δ2.7–3.5 ppm.
Table 1 Characteristics of Pluronic® block copolymers
Analysis and characterization of polyplex formation
Gel retardation assays were performed to investigate the ability of PPs to condense DNA via electrostatic interactions between the PEI and DNA at various w/w ratios ().
Figure 3 Agarose gel electrophoresis of complexes at various weight-to-weight (polymer particle/DNA) ratios: (A) F68-polyethylenimine (F68-PEI) 2KD, (B) P105-PEI 2KD, (C) P123-PEI 2KD, (D) L61-PEI 2KD, and (E) PEI 2KD.
Note: The used plasmid DNA was 0.1 μg, and copolymer/DNA complexes were prepared in phosphate-buffered saline.
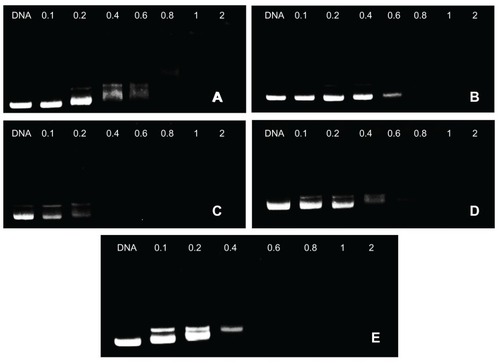
In this experiment, movement of the plasmid DNA in the gel was retarded as the amount of the cationic copolymer increased, demonstrating the copolymer bound to the DNA, neutralizing its charge.Citation4 As the w/w ratios exceeded the neutralization composition, the complexes migrated slightly toward the anode, suggesting they have a small positive charge. The assay confirmed the polymer/DNA ratio required for complete condensation and retardation of DNA. In addition, for unconjugated PEI, DNA was retarded at a w/w ratio of 0.2 (data not shown). For F68-PEI 2KD, P105-PEI 2KD, P123-PEI 2KD, L61-PEI 2KD, and PEI 2KD, DNA was retarded at a w/w ratio of 0.8, 0.8, 0.4, 0.6, and 0.6, respectively. Thus, it could shield the positive charge of the complex surface and easily condense the DNA as the amount of Pluronic increased.
shows that in the absence of DTT, PPs could completely retard plasmid DNA migration at the w/w ratio of 0.8:1. However, DNA release from PP polyplexes could be observed by gel electrophoresis in the presence of dithiothreitol 5.0 mmol/L, mimicking the intracellular reducing environment containing glutathione 0.1–10 mmol/L. For the nondegradable control polymer PEI 25KD, there was no DNA released from PEI/DNA complexes in the presence of DTT.Citation19 The rapid DNA release in the reduction condition suggests that the significant polyplex dissociation was mainly owing to the cleavage of the amide bond with DTT, leading to increased DNA release and increased gene expression.Citation20
Degradation study and resistance to nuclease degradation
Degradation of gene delivery polymers in vivo is important for safe and efficient gene delivery, because the appropriate degradation of the polymer could reduce cytotoxicity and facilitate elimination through the excretion pathway in vivo.Citation21,Citation22 Nondegradable PEI may accumulate in vivo because of a lack of degradation or excretion pathways, causing potential cytotoxicity. Therefore, it is expected that ester bonds in these polymers are susceptible to hydrolysis in physiological conditions, forming poloxamer oligomers and low-molecular-weight polyethylene.Citation23 shows degradation of the hyperbranched PPs over time at pH 7.4 and 37°C. The results indicate that the polymers degraded very slowly and the degradation rate of PPs was highly dependent on the hydrophilicity of the polymer.
Figure 5 Degradation of polymer particles – copolymers were dissolved in phosphate-buffered saline (0.1 mol/L, pH 7.4), and were incubated at 37°C with shaking at 100 rpm.
Notes: The same experiment was repeated three times for statistical analysis. Determination of molecular weight was measured by gel permeation chromatography with multi-angle laser light scattering (n = 3).
Abbreviation: PEI, polyethylenimine.
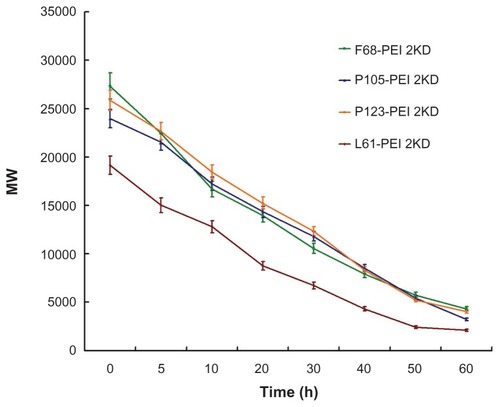
The primary responsibility of any gene delivery system is to protect DNA from degradation by nucleases.Citation24 Naked DNA was digested when treated with DNase I (, Lane 1). The other two complexes could encapsulate DNA completely and protect it from digestion by DNase I (, Lane 3). Plasmid DNA that recovered from the complexes remained nearly intact after further treatment with sodium dodecyl sulfate (an intensely electronegative substance that can displace DNA in the complexes) after DNase I digestion (, Lane 2).
Figure 6 The ability of anti-DNase of P123-polyethylenimine (P123-PEI) could protect the DNA out of DNase. Lane 1: naked plasmid DNA treated with DNase; Lane 2: polymer particle/DNA complexes treated with DNase and then with sodium dodecyl sulfate (SDS); Lane 3: polymer particle/DNA complexes as control.
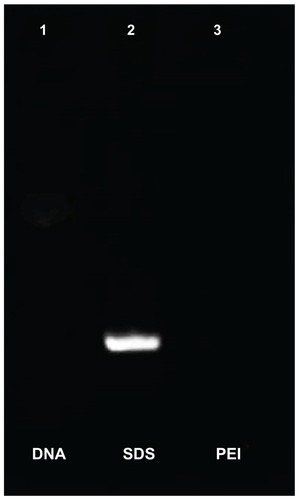
Particle size and zeta potential measurement
To investigate the effect of the cationic copolymer structure on the size of the complexes, each cationic copolymer was allowed to form complexes at different w/w ratios. shows that the mean particle size decreased significantly as the w/w ratio of PP/DNA increased, most probably because their high surface charge could condense DNA more effectively. The size of the particles formed in these systems varies from 80 to 220 nm depending on the composition of the complex, which is beneficial to efficient endocytosis and gene transfer.Citation25
Figure 7 Particle sizes of polymer particle/DNA complexes, with polyethylenimine (PEI)/DNA as control.
Notes: The same experiment was repeated three times for statistical analysis. Data expressed as mean values (plus or minus standard deviation, n = 3).
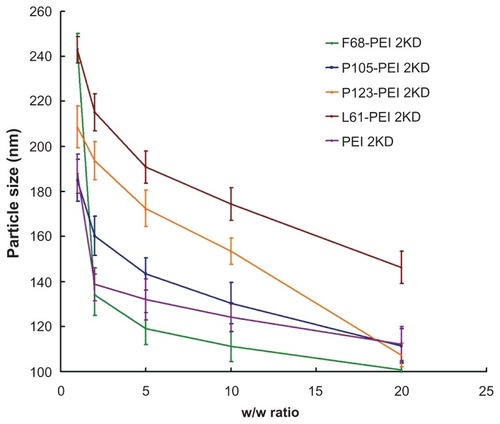
No precipitation was observed at any w/w ratio in the range of concentrations studied. Compared with unmodified PEI/DNA complexes, addition of Pluronic to PEI-based polyplexes had little effect on polyplex particle size but it led to a good stability of polyplexes.Citation10
A positive surface charge of untargeted polyplexes is necessary for binding to anionic cell surfaces, which consequently facilitates cell uptake.Citation26 However, strong cationic charges of the complexes are often cytotoxic.Citation24 Changes in the charge of the complexes upon addition of the cationic copolymers were further characterized by measuring the zeta potential of various w/w ratios. shows zeta potentials of the complexes according to the w/w ratio. The zeta potential of Pluronic-g-PEI was significantly lower than that observed for unmodified PEI at various w/w ratios. As the w/w ratio increased, so the zeta potential of the complexes also increased. Thus, the zeta potential measurements were consistent with the results of the agarose gel electrophoresis experiments.
Cytotoxicity assay
Knowing that the high-molecular-weight PEI is limited by its relatively high cytotoxicity when it is used as a transfection reagent, the authors focused on reducing the cytotoxicity and increasing the transfection efficiency. The cell proliferation assay was used to compare the cytotoxicity of Pluronic-g-PEI and PEI 25KD polymer. shows the cytotoxicity of the polymers at various concentrations with HeLa cell lines. It was found that Pluronic-g-PEI had significantly higher cell viability than PEI 25KD at any concentration (P < 0.01). It is worth noting that the modified Pluronic-g-PEI showed significantly higher cell viability at various concentrations, especially the high concentration, suggesting that transfection efficiency could be improved by administration of more PPs if necessary, knowing that the cytotoxicity is low.
Figure 9 Cytotoxicity of polymer particles at various concentrations in HeLa cell lines by Cell Counting Kit-8 assay (CCK-8, Dojindo, Japan).
Notes: The same experiment was repeated six times for statistical analysis. Each data point represents the mean plus or minus the standard deviation (n = 6, **P < 0.01).
Abbreviation: PEI, polyethylenimine.
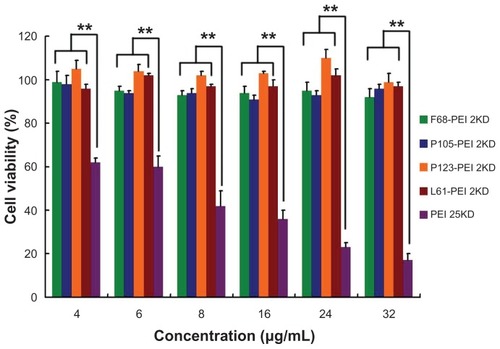
The enhanced cell viability is undoubtedly the result of the formation of low-toxic building blocks.Citation21 The cytotoxicity of cationic polymers is thought to be a result of the multiple attachment of PEI to the cell surface and the membrane-damaging effects.Citation27 The results of this study show that PEI macromolecules can be conjugated with Pluronic and that PPs are degradable under physiological conditions. The degradation products of the polymer are low-molecular-weight PEI and Pluronics, which are practically nontoxic and are rapidly excluded out of the cell nucleus and the cytoplasm or eliminated from the body. Thus, the safety of Pluronic formulations may be an important advantage for their utilization in nonviral gene delivery. Mean-while, the number of PEI attachments to cell surfaces may also be reduced, resulting in a lower cytotoxicity to cells.
In vitro transfection efficiency
Complexes formed between plasmid DNA and cationic copolymers were assessed for their in vitro transfection activity utilizing a transient expression of luciferase reporter in HeLa cell lines. For all cationic polymers, the efficacy of transfection was dependent on the electrostatic interactions and the “proton sponge effect” of PEI.Citation3 As shown in , transfection efficiency of PPs rapidly increased with the increase in ratio of PEI 2KD/Pluronic, which can be explained by the high degree of modification of PEI 2KD leading to an increase in positive surface and structural compactness of the complexes. The highest luciferase expression level was obtained at P105-PEI 2KD, and the Pluronic-g-PEI showed higher gene transfer ability than PEI 2KD. The enhanced transfection efficiency of Pluronic-g-PEI over PEI 2KD is because of the introduction of the hydrophilic EO chain and the hydrophobic PO chain of Pluronics. The hydrophilic EO chains extend into water and sterically prevent the complexes from approaching each other,Citation28 and they can shield the positive surface charge of complexes.Citation27 Therefore, the EO chain can reduce toxicity and improve the colloidal stability of the polymer/DNA complexes as well.Citation26 Also, the PO chain is believed to enhance lipophilic complexes, which possibly possess the ability of interacting with biological membranes and enhancing transport of the complexes into cells.Citation4 Therefore, the results of this study show that reducing cytotoxicity and increasing hydrophobicity and stability enhanced the efficacy of the newly synthesized Pluronic-g-PEI in transfection compared with unmodified PEI.
Figure 10 Transfection efficiency of different polymer particle/DNA complexes at the HeLa cell line.
Notes: The same experiment was repeated six times for statistical analysis. Each data point represents the mean plus or minus the standard deviation (n = 6, **P < 0.01).
Abbreviations: PEI, polyethylenimine; RLU, relative light unit.
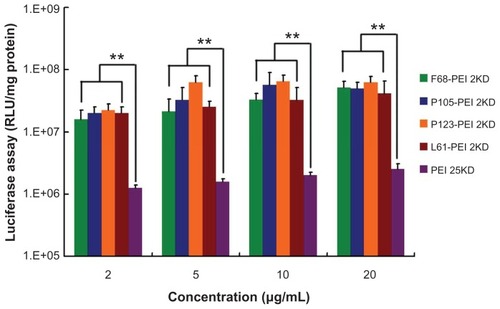
Cellular uptake study
Efficient entry of synthetic polymers inside cells is a central issue in polymeric drug delivery.Citation16 There is ample evidence that polycationic compounds can interact with negatively charged cellular membranes. However, amphiphilic block copolymers, such as Pluronic, depend on their aggregation state to cellular membranes and are usually taken into cells by endocytosis.Citation14 Previous research has suggested that the addition of Pluronic could intensify nonspecific endocytosis in several cells and could dramatically increase cellular uptake, nuclear transport, and transfection efficacy of polyplexes. Citation12 Furthermore, the effect of Pluronic on cell uptake was usually observed as early as 60 minutes after exposure.Citation10 The magnitude of the effect varied for each Pluronic type, mainly depending on the HLB value. A high HLB value for Pluronic could significantly improve serum stability of the complex. However, complexes with a lower HLB value should theoretically show marked improvement in cellular uptake compared with those with a higher HLB value.
The authors used confocal microscopy to study the intracellular trafficking of representative QDs labeled PPs. shows the cellular trafficking of PPs at the specific time. F68-PEI 2KD presented a slower association with outer membranes of HeLa cells (about 5–10 minutes) and homogeneous distribution in the cytoplasm (). This is possibly because the reduction of serum-mediated aggregation of gene transfer was prevented by the addition of high-HLB-value Pluronic F68. Serum stability of nonviral vectors is known to be a crucial factor for successful in vivo gene delivery, and therefore the authors think that these nonviral vectors incorporated with a higher HLB value for Pluronic could be used as potential vehicles for in vivo delivery of DNA because of marked improvement of their serum stability.
Figure 11 Cellular trafficking of (A) F68-polyethylenimine (F68-PEI) 2K, (B) P105-PEI 2K, (C) P123-PEI 2K, and (D) L61-PEI 2K analyzed by confocal microscopy in HeLa cells.
Notes: The first line of each group shows the respective transmitted light image, the second line shows the fluorescence micrographs of the same field, and the images in the third line are the merged pictures. Micrographs obtained at magnification 400×.
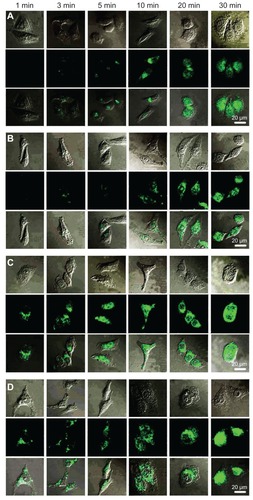
Similar pictures were obtained for P123-PEI 2KD and P105-PEI 2KD. After 30 minutes of incubation, the two PPs were mainly associated with the outer membrane, and significant quantities of drugs were already observed in cytosol (). This result reveals that P123/ P105-PEI 2KD complexes preferred intracellular accumulation sites in the perinuclear region after 30 minutes of incubation. The adjunction of Pluronic P123/P105, which had intermediate lengths of PO chains, relatively short EO segments, and appropriate HLB value, could not only form a stable dispersion but also facilitate cellular uptake of the synthetic polymers.Citation29 Therefore, P123/P105-PEI 2KD could be considered as a potential nonviral vector of systemic gene delivery, with a wide application in DNA transfection.
L61-PEI 2KD showed a rapid association with outer membranes of HeLa cells (1 minute of incubation) () because of the larger component of hydrophobic PO chain. After 30 minutes of incubation, transfection with L61-PEI 2KD–based polyplexes resulted in a more localized appearance in the nucleus and a less diffuse appearance throughout the cytoplasm than with the other Pluronics. Although Pluronic with the lowest HLB value could not show much improvement in transfection activity under serum-containing or serum-free conditions, it could lead to a substantial increase in drug accumulation and a change in cellular distribution. Citation30 These polyplexes could be used as the vector of genes that are essential to express at the nucleus of cells.
All evidence indicates that the supramolecular architecture of Pluronics may play a role in facilitating the entry of polyplexes through cell membranes and in regulating cellular distribution of the polyplexes.
Conclusion
This work reports the significant evaluation of the design and formulation of a DNA-polycation polyplex based on a Pluronic-PEI conjugate and the characterization of the cationic graft copolymer system, with the goal of developing a better understanding of this multicomponent delivery system. Complete understanding of this system is particularly important in light of the fact that Pluronics can exhibit biological activity, including effects on enhancing DNA cellular uptake, nuclear translocation, and gene expression. The authors’ work indicates that the addition of Pluronics can significantly facilitate intracellular trafficking and can change cellular distribution. The Pluronics with a higher HLB value lead to homogeneous distribution in the cytoplasm; those with a lower HLB value prefer to localize in the nucleus. As is well known, plasmid DNA should enter the nucleus before effective gene expression, whereas small interfering RNA and microRNA always play their regulatory roles in cytosol. Therefore, it is expected that these molecules are worth exploring as components in the cationic copolymers as the DNA or small interfering RNA/microRNA delivery system, respectively, in the near future.
Acknowledgments
The authors acknowledge the financial support of National Natural Science Foundation, China (30873178, 30973459, 81072100, 81172514, and 81101658). The authors thank Dr WangYue from the Department of Medical Genetics, Second Military Medical University, Shanghai, for his valuable suggestions and helpful discussions.
Disclosure
The authors report no conflicts of interest in this work.
References
- De SmedtSCDemeesterJHenninkWECationic polymer based gene delivery systemsPharm Res200017211312610751024
- WuXDingBGaoJSecond-generation aptamer-conjugated PSMA-targeted delivery system for prostate cancer therapyInt J Nanomedicine201161747175621980237
- ChoKCChoiSHParkTGLow molecular weight PEI conjugated pluronic copolymer: useful additive for enhancing gene transfection efficiencyMacromol Res2006143348353
- LeeKBaeKHLeeYLeeSHAhnCHParkTGPluronic/polyethylenimine shell crosslinked nanocapsules with embedded magnetite nanocrystals for magnetically triggered delivery of siRNAMacromol Biosci201010323924519924685
- GebhartCLKabanovAVEvaluation of polyplexes as gene transfer agentsJ Control Release2001732–340141611516515
- NguyenHKLemieuxPVinogradovSVEvaluation of polyether-polyethyleneimine graft copolymers as gene transfer agentsGene Ther20007212613810673718
- LungwitzUBreunigMBlunkTGöpferichAPolyethylenimine-based non-viral gene delivery systemsEur J Pharm Biopharm200560224726615939236
- LiuTYuXKanBEnhanced gene delivery using biodegradable poly(ester amine)s (PEAs) based on low-molecular-weight polyethylenimine and poly(epsilon-caprolactone)-pluronic-poly(epsilon-caprolactone)J Biomed Nanotechnol20106435135921323108
- NeuMFischerDKisselTRecent advances in rational gene transfer vector design based on poly(ethylene imine) and its derivativesJ Gene Med200578992100915920783
- YangZSahayGSriadibhatlaSKabanovAVAmphiphilic block copolymers enhance cellular uptake and nuclear entry of polyplex-delivered DNABioconjug Chem200819101987199418729495
- MintzerMASimanekEENonviral vectors for gene deliveryChem Rev2009109225930219053809
- GuoQShiSWangXSynthesis of a novel biodegradable poly(ester amine) (PEAs) copolymer based on low-molecular-weight polyethyleneimine for gene deliveryInt J Pharm20093791828919539737
- KoYTKaleAHartnerWCPapahadjopoulos-SternbergBTorchilinVPSelf-assembling micelle-like nanoparticles based on phospholipid-polyethyleneimine conjugates for systemic gene deliveryJ Control Release2009133213213818929605
- KabanovAVBatrakovaEVSriadibhatlaSYangZKellyDLAlakovVYPolymer genomics: shifting the gene and drug delivery paradigmsJ Control Release20051011–325927115588910
- OhKTBronichTKKabanovAVMicellar formulations for drug delivery based on mixtures of hydrophobic and hydrophilic Pluronic block copolymersJ Control Release2004942–341142214744491
- SahayGBatrakovaEVKabanovAVDifferent internalization pathways of polymeric micelles and unimers and their effects on vesicular transportBioconjug Chem200819102023202918729494
- KabanovAVBatrakovaEVAlakhovVYPluronic block copolymers as novel polymer therapeutics for drug and gene deliveryJ Control Release2002822–318921212175737
- YinDDingXGaoJChuCZouHGaoSNonionic amphiphilic surfactant conjuncted polyethyleneimine as a new and highly efficient non-viral gene carrierMacromol Res20091711925
- PiestMLinCMateos-TimonedaMANovel poly(amido amine)s with bioreducible disulfide linkages in their diamino-units: structure effects and in vitro gene transfer propertiesJ Control Release20081301384518585814
- TangGPGuoHYAlexisFLow molecular weight polyethylenimines linked by beta-cyclodextrin for gene transfer into the nervous systemJ Gene Med20068673674416550629
- KimTHCookSEAroteRBA degradable hyperbranched poly(ester amine) based on poloxamer diacrylate and polyethylenimine as a gene carrierMacromol Biosci20077561161917457939
- AroteRKimTHKimYKA biodegradable poly(ester amine) based on polycaprolactone and polyethylenimine as a gene carrierBiomaterials200728473574417034844
- XuPQuickGKYeoYGene delivery through the use of a hyaluronate- associated intracellularly degradable crosslinked polyethyleneimineBiomaterials200930295834584319631979
- LiangWGongHYinDLuSFuQHigh-molecular-weight polyethyleneimine conjuncted pluronic for gene transfer agentsChem Pharm Bull (Tokyo)20115991094110121881251
- XuZShenGXiaXComparisons of three polyethyleneimine-derived nanoparticles as a gene therapy delivery system for renal cell carcinomaJ Transl Med2011914621513541
- BertholdPRShiraishiTNielsenPECellular delivery and antisense effects of peptide nucleic acid conjugated to polyethyleneimine via disulfide linkersBioconjug Chem201021101933193820873710
- RoquesCFattalEFromesYComparison of toxicity and transfection efficiency of amphiphilic block copolymers and polycationic polymers in striated musclesJ Gene Med200911324024919189284
- HuXJiJCovalent layer-by-layer assembly of hyperbranched polyether and polyethyleneimine: multilayer films providing possibilities for surface functionalization and local drug deliveryBiomacromolecules2011121242644271 Epub November 10, 201122050053
- VinogradovSVKohliEZemanADComparison of nanogel drug carriers and their formulations with nucleoside 5′-triphosphatesPharm Res200623592093016715382
- XiaTKovochichMLiongMPolyethyleneimine coating enhances the cellular uptake of mesoporous silica nanoparticles and allows safe delivery of siRNA and DNA constructsACS Nano20093103273328619739605