Abstract
Diseases such as tuberculosis, hepatitis, and HIV/AIDS are caused by intracellular pathogens and are a major burden to the global medical community. Conventional treatments for these diseases typically consist of long-term therapy with a combination of drugs, which may lead to side effects and contribute to low patient compliance. The pathogens reside within intracellular compartments of the cell, which provide additional barriers to effective treatment. Therefore, there is a need for improved and more effective therapies for such intracellular diseases. This review will summarize, for the first time, the intracellular compartments in which pathogens can reside and discuss how nanomedicine has the potential to improve intracellular disease therapy by offering properties such as targeting, sustained drug release, and drug delivery to the pathogen’s intracellular location. The characteristics of nanomedicine may prove advantageous in developing improved or alternative therapies for intracellular diseases.
Introduction
Worldwide, a number of diseases (eg, HIV/AIDS [human immunodeficiency virus/ acquired immune deficiency syndrome], hepatitis, and tuberculosis [TB]) are caused by intracellular pathogens. Such diseases can be due to viral,Citation1–Citation8 bacterial,Citation9–Citation16 fungal,Citation17,Citation18 or other parasiticCitation19–Citation21 infection, as summarized in . The prevalence of each disease may differ geographically, but the intracellular nature of these pathogens, which may protect them from a variety of antibiotic therapies and host immune responses, presents a treatment challenge for the global medical community. Some antibiotic drugs like aminoglycosides and β-lactams have limited cellular penetration, whereas others such as fluoroquinolones or macrolides have the ability to penetrate host cells but are poorly retained and therefore inefficient.Citation22 Therapeutic drugs targeting the intracellular pathogens should overcome the cell membrane barriers and release and retain the drug intracellularly at the therapeutic level for a desired time period. Moreover, multidrug resistance is increasingCitation23–Citation29 and is making intracellular disease treatment more challenging. Therefore, there is a need for the development of advanced treatment methods to better control intracellular infections. This review will summarize the status of intracellular disease treatments and the current therapeutic strategies against common intracellular diseases, and present how nanomedicine is emerging as an attractive platform for advanced intracellular drug therapy. Note that therapeutic treatments that tune the cell-mediated immune responses against intracellular pathogens are important but will not be discussed here.
Table 1 Summary of disease-causing intracellular pathogens
Intracellular pathogens and current treatment approaches
A wide variety of pathogens are capable of causing intracellular diseases such as HIV/AIDS, hepatitis, and TB (see ). In this section, a brief description of selected viral and bacterial diseases is given along with a discussion of the current therapeutic strategies against the causative pathogens.
Tuberculosis
TB is a disease caused by Mycobacterium tuberculosis (M. tuberculosis). It is estimated that up to 2.2 billion people carry latent TB infections worldwide.Citation30 Exposure to TB does not necessarily lead to an active infection, as normal human immune systems are able to effectively control bacteria, and most people remain in a symptom-free latent stage of infection. Citation30 However, susceptible individuals with poor immune response or complicating factors such as HIV infection may develop an active TB infection.Citation31 People with active infections typically experience pain in the chest and a cough with blood or sputum (phlegm) lasting more than 3 weeks.Citation32 These symptoms could also be accompanied by fatigue, weight loss, fever, chills, or night sweats.Citation33 M. tuberculosis is a gram-positive bacterium with a very thick cell wall, which is characteristic of the Mycobacterium species. The thick cell wall provides an excellent permeability barrier, making Mycobacteria resistant to a wide variety of antimicrobial agents.Citation23 M. tuberculosis bacteria may reside and duplicate within macrophages of the lung.Citation34 A full description of M. tuberculosis pathogenesis is beyond the scope of this discussion and is reviewed elsewhere.Citation35,Citation36 However, an important factor is the bacteria’s ability to avoid the cell-mediated immune response through granuloma formation,Citation10 such that treatment of TB remains a challenge.
At present, the treatment of choice for an active TB infection is long-term antibiotic therapy, with an initial “intensive phase” consisting of the four first-line anti-TB drugs (isoniazid, rifampicin, ethambutol, and pyrazinamide) followed by a typical 4 month course of rifampicin and isoniazid alone.Citation37 This has been the most effective treatment to date, although, due to the length of antibiotic therapy, side effects frequently developCitation38 and the cost is high.Citation39 These factors may lead to low patient compliance and contribute to the development of drug-resistant bacteria.Citation40
HIV
Infection with HIV is a significant ongoing problem worldwide. As HIV infection progresses, infected individuals develop AIDS. According to the latest statistics from the World Health Organization, there are 33.3 million people living with HIV/AIDS.Citation41 Many of those infected live in sub-Saharan Africa, where access to treatment is extremely costly or unavailable. There have been major developments in the treatment of HIV/AIDS since the approval of zidovudine (Retrovir®; GlaxoSmithKline, Durham, NC) in 1987.Citation8 Current therapeutic efforts consist of a combination of several drugs,Citation42 typically from different classes of antiviral drugs.Citation43,Citation44 This regimen is referred to as highly active antiretroviral therapy (HAART) and has become the standard of care for those infected with HIV. There are five classes of drugs available for HIV/AIDS treatment, including nucleoside reverse transcriptase inhibitors, nucleotide reverse transcriptase inhibitors, non-nuceloside reverse transcriptase inhibitors, protease inhibitors, and viral fusion and integrase inhibitors. Typical regimens are combinations of three or four drugs, with subsequent modifications made based on the patient’s response to therapy.Citation45 Changes made to a patient’s regimen are often based on drug resistance testing, and take into consideration toxicity and tolerability of the new treatment strategy.Citation45 Although HAART has increased the median survival time of HIV/AIDS patients from less than a year to about 10 years,Citation29 patients often develop multidrug-resistant strains of the virusCitation41 over the course of therapy, leading to poor treatment outcomes.Citation46
Hepatitis C virus
It is estimated that at least 3 percent of the world’s population is infected with the hepatitis C virus (HCV).Citation3,Citation47 The virus affects only humans and is considered a “silent” disease, as infected individuals are usually symptom free until later stages of infection when liver inflammationCitation48 occurs. HCV can cause liver scarring and cirrhosis, which ultimately leads to hepatocarcinoma (liver cancer) and death.Citation3,Citation48 Unfortunately, there is no effective vaccineCitation49 against HCV, and the “gold standard” of HCV treatment is combination antiviral therapy with ribavirin and interferon-α;Citation4 however, both of these compounds are highly toxic and may cause severe side effects. Several months of treatment are usually required to eradicate a chronic infection, and the cost of therapy is high.Citation49 These factors contribute to low patient compliance, making therapy less effective and potentially contributing to the development of viral resistance.Citation29
Typhoid fever
Typhoid fever is an acute illness caused by the bacterium Salmonella enterica serovar Typhi (S. Typhi) or Paratyphi, which causes about 20 million cases of illness per year.Citation50 These bacteria are usually ingested by consuming contaminated food or water.Citation51 Once ingested, the bacteria translocate across intestinal epithelial cells and establish an intracellular growth environment within macrophages.Citation52 The bacteria survive within Salmonella-containing vacuoles in infected macrophages and later spread to organs such as the liver and spleen.Citation11,Citation12,Citation53 Infected individuals often experience sustained high fevers (up to 103°F), stomach pains, headache, weakness, and appetite loss.Citation51 Several vaccines against S. Typhi have been developed,Citation54 although they provide only short-term protection against the disease due to failure of the immune system to build a lasting response.Citation50,Citation54 Typhoid fever is endemic in many developing countries,Citation55 where access to vaccines and antibiotic drugs is limited and expensive even if available. Standard treatment for S. Typhi infection is the antibiotic chloramphenicol, although resistance has been reported.Citation50 Newer antibiotics like the fluoroquinolones have also proven effective in treating typhoid fever, but the widespread use of these drugs for a range of febrile illnesses in developing countries is contributing to the development of more drug-resistant strains of the bacterium.Citation55
Major challenges in treating intracellular pathogens
summarize the common intracellular viruses and bacteria and their related current therapeutic approaches. One can see that intracellular treatment approaches generally involve long-term therapy with a combination of drugs. Side effects can develop due to the drug’s inherent toxicity or the length of drug exposure. One of the critical challenges in treating these types of infections is to get enough drugs to reach the pathogens within their intracellular compartments. After reviewing the literature, we found evidence that intracellular pathogens reside in phagosomes,Citation15,Citation34,Citation56 vacuoles,Citation52,Citation53 cytosol,Citation16,Citation57,Citation58 and nucleus,Citation1,Citation59,Citation60 and may interact with the Golgi apparatusCitation61 and endoplasmic reticulumCitation62–Citation66 of host cells (). The host cell membranes make it a challenge for many drugs to reach the invading pathogens. Moreover, some antiviral and antibiotic medications have short half-lives, requiring frequent and large doses to obtain a therapeutic effect, which may lead to high cost, low patient compliance, and severe side effects. In addition, drug resistance may be developed when patients do not fully comply with their treatment regimensCitation28 or when pathogens are exposed to drugs at suboptimal concentrationsCitation67 for an extended time period. An alerting new phenomenon is that some pathogens that have traditionally been considered extracellular are emerging as intracellular pathogens and may lead to new types of intracellular diseases. As an example, Staphylococcus aureus (S. aureus) has long been considered an extracellular pathogen, although recent evidence in the literature demonstrates that this bacterium is capable of being internalized and of surviving within host cells (eg, osteoblastsCitation68–Citation72), and may contribute to recurrent infections.Citation73 In order to reduce side effects, improve patient compliance, and reduce the development of drug resistance, more effective therapeutic approaches need to be developed.
Table 2A Current therapeutic strategies against selected viral pathogens
Figure 1 Potential locations of intracellular pathogens. In a typical eukaryotic cell, pathogens may be internalized via endocytic mechanisms before establishing their intracellular life cycle. Pathogens may reside in various locations, including the cytosol, phagosome, lysosome, or vacuole compartments and the nucleus, and some may associate with the Golgi apparatus or endoplasmic reticulum. (1) Cytosol (Francisella tularensis,Citation57 Listeria monocytogenes,Citation58 ShigellaCitation64). (2) Phagosome/lysosome or vacuole (Mycobacterium tuberculosis,Citation43,Citation45 Brucella species,Citation15 Salmonella,Citation52,Citation53 LegionellaCitation56). (3) Nucleus (herpes simplex virus,Citation1,Citation60 HIVCitation59). (4) Golgi apparatus (ChlamydiaCitation61). (5) Endoplasmic reticulum (hepatitis C virus,Citation65 Brucella,Citation63 Toxoplasma gondii,Citation66 Legionella pneumophiliaCitation62,Citation64).
Note: Reproduced with permission from the Scripps Institution of Oceanography, UCSD.
Abbreviations: HIV, human immunodeficiency virus; UCSD, University of California, San Diego.
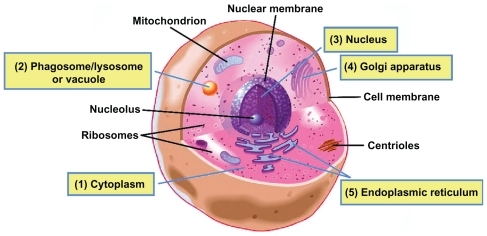
An ideal drug treatment method to eradicate intracellular pathogens is one that has the following characteristics: the ability to penetrate host cells and reach the pathogens, high efficacy and low toxicity, and sustained and site-specific drug release.Citation22 Recent evidence from the literature shows that nanomedicine is emerging as a promising potential treatment for intracellular pathogens, as nanomedicine has the capacity to address these specific challenges.
Nanomedicine as an emerging therapeutic approach
Conventional therapies for the treatment of intracellular diseases have existed for many years. These treatments may be further improved as we enter a new era of therapies based on nanomedicine. Nanomedicine can be most succinctly defined as “the application of nanotechnology to medicine.”Citation74 There are many potential advantages of using nanomedicine over the conventional therapies previously described. Combining new knowledge of nanomaterials with our current understanding of cellular and molecular functions may allow for the development of novel and advanced nanomedicines. As discussed here, nanomedicine has the capacity to incorporate, encapsulate, or conjugate a variety of drugs in order to target specific cell populations and to offer tunable and site-specific drug release. Nanomedicine could be advantageous in treating intracellular diseases.
Biocompatibility and nanotoxicity
Biocompatibility is an important feature of any drug delivery system, and the goal is to minimize nonspecific cytotoxic effects to healthy tissues while maximizing drug effects at the target tissue or against invasive pathogens.Citation75 Nanoparticles have been fabricated using a variety of materials, including poly(lactide-co-glycolide) (PLGA);Citation76–Citation81 poly-lactic acid (PLA);Citation77,Citation82,Citation83 polymethacrylic acid (PMA);Citation84,Citation85 polyethylene glycol (PEG);Citation77,Citation86,Citation87 “natural” polymers such as chitosan,Citation88,Citation89 gelatin,Citation90,Citation91 or alginate;Citation92,Citation93 and other materials such as lipids,Citation94–Citation96 gold,Citation75,Citation97 and silica.Citation98–Citation101
PLGA has been approved by the Food and Drug Administration for several biomedical applications, including surgical sutures, implants, and prosthetic devices.Citation78 PLGA micro- or nanoparticles have also been used for a variety of drug delivery applications.Citation79 PLGA displays good biocompatibility, biodegradability, suitable degradation kinetics, and mechanical properties and is easy to process. For this reason, PLGA is an attractive candidate for nanoparticle-based drug delivery systems, and there is a large body of ongoing research in this area. Other polymers such as PLA, PMA, PEG, chitosan, gelatin, and alginate also show promise as drug delivery vehicles due to their biocompatible properties. PEG may be used as a “coating” to prevent the rapid removal of nanoparticles from the bloodstream by the mononuclear phagocytic system, which may increase nanoparticle circulation time and theoretically improve the therapeutic capacity of the nanoparticle.Citation83,Citation86 Chitosan and alginate are polymers derived from natural sources and may offer more “friendly” conditions for the encapsulation or incorporation of DNA or peptides, because the use of organic solvents can be avoided.Citation93
Lipids are also being explored as potential nanodelivery systems, either as liposomes or lipid nanocapsules (LNCs). Liposomes are composed of lipid bilayers surrounding a hydrophilic “core” and can be designed to incorporate hydrophobic drugs within the lipid bilayer or hydrophilic drugs within the aqueous core.Citation94 Liposome-encapsulated aminoglycosides (such as gentamicin) have shown higher therapeutic efficacy than conventional aminoglycoside preparations in the liver and spleen using a murine S. typhi infection model.Citation94 Amphotericin B (an antifungal) has also been encapsulated in lipids and administered to mice with an Aspergillus fumigatus infection.Citation95 Liposome-treated mice have survived longer than mice treated with other amphotericin B preparations, with reduced renal toxicity and a prolonged drug circulation time.Citation95 These liposome formulations could be a better treatment for diseases affecting the liver or spleen (eg, typhoid fever, hepatitis). LNCs are another type of lipid-mediated delivery system under exploration. LNCs are considered a “hybrid” between a polymer nanoparticle and a liposome, with an oily core surrounded by a membrane composed of PEGylated surfactants.Citation96 LNCs have shown promise in in vitro and some animal models encapsulating anticancer drugs such as paclitaxel, doxorubicin, and etoposide.Citation96 The results of these studies have indicated higher intracellular drug delivery and reduced tumor size in vivo when LNC formulations were administered.
In contrast to these “soft” polymer-based nanoparticle systems, metals such as gold have also been explored as potential drug delivery vectors. Gold is an attractive drug delivery vector due to the ease with which biomolecules such as protein or DNA can be attached to the gold surface using thiol chemistry.Citation75 This process can also allow attachment of multiple targeting or functional groups to the nanoparticle surface to produce a multifunctional nanoparticle. Although gold nanoparticles can be easily functionalized, these nanoparticles may accumulate in tissues over time because they are not biodegradable. The effects of long-term nanoparticle accumulation are unknown, so in many cases it may be better to use a material that is fully biodegradable. Silica-based compounds are another option, as the biodegradation of silica avoids tissue accumulation concerns,Citation98 and it has been demonstrated that a variety of agents have been successfully incorporated into silica-based nanoparticlesCitation99–Citation101 for drug delivery applications.
Although each of these materials offers its own set of characteristics and biocompatible properties, some materials may be more suited to certain applications than others. It is important to determine the desired properties of the nanomedicine for defined applications.
Cellular penetration and intracellular delivery
One critical challenge in treating intracellular pathogens is to get enough drugs to reach the pathogen within an intracellular compartment. Nanoparticles can be internalized by endocytic mechanisms, which include receptor-mediated or clathrin-coated pit endocytosis.Citation102 Nanoparticles may reside within acidic endolysosome compartments following endocytosis,Citation79 and premature drug release within this acidic compartment may cause drug degradation and render treatment ineffective. It is therefore important for the nanoparticle to escape this compartment and gain access to the cytosol where either the drug cargo may be directly released or the nanoparticle goes on to further target a specific organelle. For instance, PLGA nanoparticles carrying doxorubicin are reportedly capable of escaping the endolysosomal compartment by a reversal of their surface charge. This allows the particles to interact with the membrane and escape into the cytosol where the doxorubicin is released.Citation79
There are a number of sources that report time- and concentration-dependent uptake of nanoparticles by a variety of cell types such as smooth muscle cells,Citation79 endothelial cells,Citation103,Citation104 macrophages,Citation40,Citation105–Citation107 and tumor cells.Citation108–Citation113 The uptake of PLGA nanoparticles containing bovine serum albumin as a model drug was found to be concentration dependent in human vascular endothelial cells, approaching first-order kinetics.Citation103 An in vitro uptake and cellular trafficking study using mesoporous hybrid silica nanoparticles demonstrated that the particles were internalized by receptor-mediated endocytosis, were localized in the endocytic compartment, and then released their cargo within the cytosol.Citation100 Another in vitro study examined the uptake of drug-loaded thiolated PMA hydrogel capsules.Citation113 The tracking experiments revealed that nanocapsules were taken up by endocytic mechanisms in a time-dependent manner and the drug was released throughout the cell. Collectively, these studies demonstrate that nanoparticles are capable of cellular penetration and intracellular drug release. This is an important characteristic of a nanomedicine that targets intracellular pathogens and promotes direct killing, as the drug must be able to effectively reach the invading pathogens within the intracellular compartment.
Targeting to specific cell types
The goal of targeting nanomedicines to specific cell populations is to increase the therapeutic efficacy of the drug while minimizing damage to healthy cells and tissues, thereby reducing the incidence and severity of side effects. Currently, the majority of experiments demonstrating the targeting of nanoparticles are related to cancer/tumor targeting, although these same concepts can be applied to pathogen-infected cells and the intracellular compartmentsCitation98 where the pathogens reside. Nanoparticles can be targeted toward specific cell populations by conjugating targeting ligands to the surface of the nanoparticles. These targeting ligands can be attached directly to nanoparticle surfaces or via a spacer (eg, PEG), which acts to enhance the flexibility of the targeting ligand and increase the likelihood that it will bind the appropriate receptor on the target cell.Citation110,Citation114 There are a variety of methods available to attach ligands to nanoparticle surfaces.Citation115,Citation116 Targeting ligands can include antibodies (whole or fragment), receptors or receptor ligands, peptides, aptamers, or other small molecules.Citation114 A few examples are presented here.
When incorporating antibodies on nanoparticle surfaces, it is important to consider whether attachment will affect the binding site or structure of the antibody. Hybrid lipid nanoparticles composed of PLGA, phospholipids, and an outer PEG layer have shown targeting capacity to pancreatic cancer cells when coupled with an anticarcinoembryonic antigen (CEA) half-antibody.Citation112 Nanoparticles incubated with CEA-presenting pancreatic cancer cells showed selective uptake of targeted nanoparticles over nontargeted control nanoparticles.
Receptor ligands can also act as targeting moieties. It has recently been reported that nanosized poly(ethylene glycol)-block-poly(ɛ-caprolactone) (PEG-b-PCL) micelles bearing a surface epidermal growth factor (EGF) have been targeted to breast cancer cells overexpressing the epidermal growth factor receptor (EGFR) in vitro and in vivo.Citation108 The uptake of the nanomicelles was two-fold higher using EGFR-overexpressing cells compared with cells that express low levels of EGFR. These results were confirmed using a xenograft mouse model, with mice bearing EGFR-overexpressing tumors demonstrating increased tumor uptake of the particles compared with mice bearing low EGFR-expressing tumors.
Polyester nanoparticles carrying paclitaxel can be targeted to irradiated tumor cells with a short peptide, Gly-Ile-Arg-Leu-Arg-Gly (GIRLRG), which binds specifically to GRP78 receptors expressed by glioma and breast tumor cells in response to radiation therapy.Citation109 In vitro and in vivo studies were used to compare tumor volumes following treatment with control and targeted nanoparticles. Mice treated with nanoparticles bearing the targeting component had a significant decrease in tumor volume compared with control.
Conjugation of an A10 aptamer to PLGA/PEG nanoparticles has also been shown to be an effective targeting strategy.Citation116 The A10 aptamer binds to the prostate-specific membrane antigen (PSMA) on the surface of prostate cancer cells, and the A10 aptamer-conjugated nanoparticles showed higher uptake by PSMA-positive cells than by PSMA-negative cells.
Site-specific and tunable drug release
In order to effectively eradicate intracellular pathogens, drugs that are intended to kill the pathogens directly should reach the intracellular locations of infected host cells. As we have summarized in , locations may include phagosomes,Citation15,Citation34,Citation56 vacuoles,Citation52,Citation53 cytosol,Citation16,Citation57,Citation58 and nucleusCitation1,Citation59,Citation60 and may interact with the Golgi apparatusCitation61 or endoplasmic reticulum.Citation62–Citation66 With proper engineering and design, nanoparticles can be tailored to carry their drug payloads into the infected cells and then release the drug within specific intracellular compartments. One way to accomplish site-specific drug release is to use pH-responsive polymers. In one study, short peptides were conjugated to pH-responsive polymers designed specifically to disrupt the endosomal membrane at pH 5.5 and subsequently release the peptide into the cytosol.Citation117 The polymers had no membrane-disruptive activity at pH 7.4 due to a “masking” PEG group, which is later cleaved to expose the membrane-disruption domain at pH 5.5. Peptide-polymer conjugates demonstrated a diffuse cytosolic distribution after 1 hour, the time normally required for macrophage endosomes to mature into lysosomes. Unconjugated peptide was located primarily in the lysosome after the same time period, indicating that the peptide itself was unable to escape into the cytosol. This polymer technology may allow for local drug delivery to the cytosol, although it is more critical to reach the specific intracellular location of the pathogen, such as a vacuole or the nucleus.
It has been demonstrated that nanoparticles can be specifically targeted to mitochondriaCitation118 or nucleusCitation119 and may be capable of entering vacuolesCitation120 where pathogens such as Salmonella may reside during an infection process. Although intracellular pathogens do not typically live within mitochondria, pathogens such as Listeria monocytogenes can secrete toxins that interfere with normal mitochondrial function.Citation121 So the ability to target mitochondria may provide a means to treat these types of infection and attenuate the effects of secreted toxins. One study successfully localized fluorescent nanodiamonds conjugated with mitochondrial protein antibodies to mitochondria in live cells.Citation118 The inherent fluorescence of these nanodiamonds allowed for tracking the localization of the nanoparticles, and the microscopy experiments revealed that these nanodiamonds were capable of specifically binding the mitochondria compared with control nanodiamonds without conjugated antibody.
Nuclear targeting has been demonstrated in a similar manner by conjugating nuclear localization signal (NLS) peptides on the surface of PLGA nanoparticles for the nuclear delivery of doxorubicin to cancer cells.Citation119 The NLS-targeted nanoparticles demonstrated a six-fold increase in uptake compared with free doxorubicin in solution, and also a 2.5-fold increase in uptake over nontargeted nanoparticles. Cells treated with NLS-targeted nanoparticles also showed a higher toxicity than control nanoparticles, which was expected due to the increased delivery of drug to its nuclear target site. Nuclear targeting would be especially useful in viral infections such as HIV and HSV, as these viruses must enter the nucleus to begin their replicative life cycles.
There is also evidence that demonstrates that nanoparticles loaded with ampicillin are capable of entering cells and delivering the drug within Salmonella-containing vacuoles (SCVs).Citation120 Ampicillin was tritium-labeled and found to localize within both the cytosol and SCVs using ultrastructural autoradiography. The colocalization of the drug with the bacteria led to enhanced bacterial killing and elimination compared with control cells treated with ampicillin in solution. The colocalization of the drug within the SCVs is an important step in effectively targeting intracellular pathogen compartments.
Another issue plaguing intracellular pathogen treatments is the lower concentration of drug at the target site and the short duration of efficacy of the drug administered. Large and frequent doses of the drugs are often required to obtain a therapeutic effect. Nanoparticles have the potential to overcome this issue by offering sustained release of drugs, which would lower the required dose and decrease the frequency of administration.Citation122 As an example, polymer-based nanoparticles composed of PLGA are able to sustain release of a variety of drugs, such as rolipram for 7 days,Citation123 gentamicin for 25 days,Citation124,Citation125 and dexamethasone for 15 days.Citation126 Hyperbranched poly(amine-ester)-PLGA nanoparticles have also demonstrated sustained release of isoniazid, an important anti-TB drug, up to 14 days.Citation81 Another study targeted gelatin nanoparticles loaded with rifampicin, another anti-TB drug, to macrophages.Citation91 Test results indicated that the rifampicin-loaded gelatin nanoparticles were capable of localizing in the lungs and reducing bacterial loads in a mouse model of TB. In addition, nanoparticle treatment was as effective as traditional daily oral rifampicin at a reduced dosing frequency (every 3 days), due to the sustained release of rifampicin from the gelatin matrix.Citation91
Perspectives for the future
We have reviewed the current therapeutic strategies against commonly encountered intracellular pathogens like viruses and bacteria, and have evaluated the potential of nanomedicine to improve upon the current treatments. When treating intracellular diseases, it is important to avoid or prevent further development of pathogen resistance, which is becoming a major problem for the management of TB.Citation23 Generally speaking, the success of an antibiotic drug relies on its ability to penetrate the bacterial cell wall and membranes and to bind to its target site (eg, protein, enzyme).Citation127 Bacteria may develop resistance by mutating various drug target sites,Citation27 and viruses may also develop drug resistance through genetic mutation and recombination eventsCitation29 that can render our current treatments ineffective. For this reason, there is a need for new drugs with novel mechanisms of action that may slow or stop the development of pathogen resistance.
As an example, recent literature has emerged touting the potential of cationic antimicrobial peptides (CAMPs) to serve as an alternative to conventional antibiotic therapy.Citation128–Citation132 Antimicrobial peptides are short, positively charged peptides that are produced endogenously by human epithelial tissues where they function to prevent invasion of pathogens, demonstrating broad-spectrum killing activity against bacteria, yeast, and fungi.Citation129,Citation133 The complete mechanism of CAMP action is yet to be fully understood, but one proposed mechanism suggests that the CAMPs interact electrostatically with the negatively charged molecules on the outer surface of bacteria, where they can insert themselves into the membrane and form a pore.Citation129 Such a disruption in the bacterial cell wall and membranes will affect the osmolytic balance of the bacterium and ultimately cause cell death. Because CAMPs do not have a specific molecular target per se, pathogens may have more difficulty in developing resistance to these peptides. There is also some evidence indicating that CAMPs can help modulate exogenous antibiotic action against several strains of S. aureus.Citation134 Bacterial cultures treated with both CAMPs and antibiotics had lower antibiotic minimum inhibitory concentration values than for cultures treated with antibiotics alone, indicating complementary action between the CAMPs and antibiotics.
As a result, these CAMPs are attractive targets for the development of novel nanomedicines to treat intracellular diseases. It may be possible to design nanoparticles capable of carrying CAMPs into the cell, or to develop self-assembled CAMP nanoparticles. The development of such nanoparticles may greatly improve intracellular drug therapy by offering high efficacy against a variety of pathogens, and also offer very high biocompatibility, as endogenous CAMPs can be used. We anticipate that CAMP nanoparticles will be a potential advanced nanomedicine for intracellular disease treatments.
Another potential strategy to improve intracellular disease treatments is to take advantage of the preferential accumulation of nanoparticles by the reticuloendothelial system (RES). The RES can also be referred to as the “mononuclear phagocytic system” and is composed of macrophages residing in the liver, spleen, and lungs. Normally, this phenomenon is avoided in nanomedicine, as the RES removes the nanoparticles from circulation and may prevent drugs from reaching target tissues. However, this treatment modality may be particularly useful in treating intracellular diseases such as TB (affecting the lungs) or typhoid fever (affecting the liver and spleen), as these pathogens primarily live and duplicate within macrophages of the affected organs. Using nanoparticles to deliver drugs of interest to fight these diseases may be aided by the natural tendency of these cells to internalize nanoparticles, which would help reduce unnecessary tissue exposure and likely decrease the amount of drug required, because it is being delivered to the appropriate cell.
To create the “perfect” intracellular drug delivery system for fighting infections, certain characteristics of nanoparticles or nanomedicine must be successfully incorporated such that the medicine exhibits acceptable biocompatibility, possesses targeting capacity, and offers efficient and sustained drug release at the target site. illustrates an “ideal” nanoparticle drug delivery system with such characteristics suited to treat intracellular diseases. The nanomaterial used will vary with each application, but there are a variety of biocompatible materials available, as summarized in . The drug may be used to form the nanoparticle, encapsulated within a polymer matrix, or attached to the surface of a solid “carrier” nanoparticle. The incorporation of surface targeting components to help localize to the affected tissue may improve therapeutic efficacy, along with the presence of molecules to enhance cellular penetration such as cell-penetrating peptides (CPPs). CPPs are short, cationic peptides, typically derived from the HIV TAT proteins,Citation135 which have been shown to readily translocate through cell membranes. The addition of CPPs, such as TAT, to the surface of a nanoparticle can increase the efficiency with which the nanoparticles are delivered intracellularly,Citation136,Citation137 although the mechanism by which these CPPs are able to enter cells is currently under debate. It is suggested that either the CPPs promote direct translocation through the membrane via electrostatic interactions or the CPPs bind to specific receptors on the membrane and induce rapid receptor-mediated endocytosis.Citation137 In either case, promoting efficient cellular penetration is critical for the treatment of intracellular diseases.
Table 2B Current therapeutic strategies against common intracellular bacterial pathogens
Table 3 Examples of biocompatible nanoparticles discussed in this review
Figure 2 Components of an “ideal” nanoparticle for intracellular drug delivery. The important components of a nanoparticle used for intracellular drug delivery include choice of nanomaterials (eg, polymer, gold), targeting molecules, cellpenetrating peptides (to promote internalization), and the incorporated drug molecules of interest.
Abbreviation: PEG, polyethylene glycol.
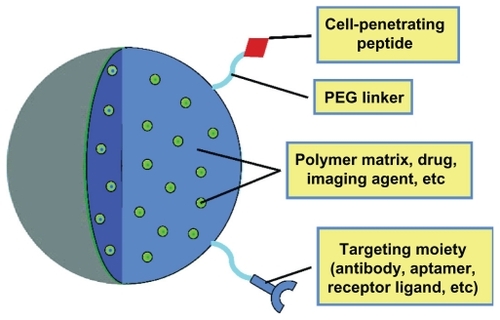
Nanomedicine meets the requirements for an “ideal” drug delivery system to improve intracellular disease therapy; however, we are still exploring this relatively new field. It is early to assess how quickly nanomedicine will be adopted and integrated into mainstream health care. Research on nanomedicine is relatively well funded,Citation138 so it is likely that many new treatment methods will be approved and available in the future. However, this investment cost for drug development is still high from an economic standpoint,Citation139 and the price will probably be passed down to patients via high prescription copays and may not be available for years in developing areas where these improved therapies are needed most. Initially, this may be a deterrent to promoting nanomedicine, but over the long term with new cost-effective technologies, higher drug efficacy, and better treatment outcomes, the price will become acceptable.
Conclusion
The burden of treating intracellular diseases is continually increasing due to the sheer number of people living with diseases such as HIV/AIDS, hepatitis, and TB worldwide, along with the increasing incidence of drug resistance. We have summarized the locations where these pathogens reside, such as phagosomes,Citation15,Citation33,Citation55 vacuoles,Citation51,Citation52 cytosol,Citation16,Citation56,Citation57 nucleus,Citation1,Citation58,Citation59 Golgi apparatus,Citation60 and endoplasmic reticulum.Citation61–Citation65 The major challenges in treating these invasive pathogens include getting enough drugs to penetrate the host cell and reach the pathogen, having high drug efficacy and low toxicity, and maintaining sustained, site-specific drug release throughout the duration of treatment. In the future, it may be possible to improve disease treatment by utilizing the uptake of nanoparticles by the RES, especially in the case of TB and hepatitis, which affect macrophages. Additionally, looking to endogenous sources such as CAMPs or other immunomodulatory compounds such as interleukins (not covered) may further improve upon current therapies.
The literature presented here shows the potential for nanomedicine to address these challenges and improve upon the current therapeutic strategies. The incorporation of nanomedicine into mainstream health care is a lofty, but achievable, goal. Researchers from multiple disciplines must work together, push the boundaries of science at the nanoscale, and incorporate concepts from biology, engineering, and drug design in order to make this goal a reality.
Acknowledgments
The authors thank Suzanne Smith and Katherine Hickey for their thoughtful and critical commentary during the manuscript revision process.
Disclosure
The authors acknowledge financial support from the National Science Foundation (No 1003907), the National Institutes of Health (No HL091456), and WVNano.
References
- AkhtarJShuklaDViral entry mechanisms: cellular and viral mediators of herpes simplex virus entryFEBS J200927677287736
- WilsonSSFakioglyEHeroldBCNovel approaches in fighting herpes simplex virus infectionsExpert Rev Anti Infect Ther2009755956819485796
- AlterMJEpidemiology of hepatitis c virus infectionWorld J Gastroenterol2007132436244117552026
- SharmaSDHepatitis c virus: molecular biology and current therapeutic optionsIndian J Med Res2010131173420167971
- EmpeyKMStokes PeeblesJRKollsJKPharmacological advances in the treatment and prevention of respiratory syncytial virusClin Infect Dis2010501258126720235830
- WelliverRCPharmacotherapy of respiratory syncytial virusCurr Opin Pharmacol20101028929320493773
- ChatterjeeKHost genetic factors in susceptibility to HIV and progression to AIDSJ Genet20108910911620505255
- EsteJACihlarTCurrent status and challenges of antiretroviral research and therapyAntiviral Res201085253320018390
- LoBuePSEnarsonDAThoenTCTuberculosis in humans and its epidemiology, diagnosis and treatment in the United StatesInt J Tuberc Lung Dis20101411261232
- FlynnJLChanJImmunology of tuberculosisAnnu Rev Immunol2001199312911244032
- LahiriALahiriAIyerNDasPChakravorttyDVisiting the cell biology of salmonella infectionMicrobes Infect20101280981820538070
- WatsonKGHoldenDWDynamics of growth and dissemination of Salmonella in vivoCell Microbiol2010121389139720731667
- FrancoMPMulderMGilmanRHSmitsHLHuman brucellosisLancet Infect Dis2007777578618045560
- GamazoCLecarozMCPriorSVitasAICampaneroMAIracheJMChemical and biological factors in the control of Brucella and brucellosisCurr Drug Deliv2006335936517076637
- RoopRMBellairBHValderasMWCardelliJAAdaptation of the brucellae to their intracellular nicheMol Microbiol20045262163015101970
- TheirotJAThe cell biology of infection by intracellular bacterial pathogensAnnu Rev cell Dev Biol1995112132398689557
- Lopez-MartinezRCandidosis, a new challengeClin Dermatol20102817818420347660
- BalloyVChignardMThe innate immune response to Aspergillus fumigatisMicrobes Infect20091191992719615460
- GomezMAOlivierMProteases and phosphatases during Leishmania- macrophage interaction: paving the road for pathogenesisVirulence2010131431821178462
- GotoHAngeloJLindosoLCurrent diagnosis and treatment of cutaneous and mucocutaneous leishmaniasisExpert Rev Anti Infect Ther2010841943320377337
- SadanandSMalaria: an evaluation of the current state of research on pathogenesis and antimalarial drugsYale J Biol Med20108318519121165337
- BrionesEColinoCILanaoJMDelivery systems to increase the selectivity of antibiotics in phagocytic cellsJ Control Release200812521022718077047
- ChiangCYSchaafHSManagement of drug-resistant tuberculosisInt J Tuberc Lung Dis20101467268220487603
- NguyenHMGraberCJLimitations of antibiotic options for invasive infections caused by methicillin-resistant Staphylococcus aureus: is combination therapy the answerJ Antimicrob Chemother200965243619861337
- WoodfordNLivermoreDMInfections caused by gram-positive bacteria: a review of the global challengeJ Infect200959Suppl 1S41619766888
- FinchRBacterial resistance-the clinical challengeClin Microbiol Infect20028Suppl 3213212519346
- HawkeyPMJonesAmThe changing epidemiology of resistanceJ Antimicrob Chemother200964Suppl 1310
- GoossensHAntibiotic consumption and link to resistanceClin Microbiol Infect200915Suppl 3121519366364
- Margeridon-ThermetSShaferRWComparison of the mechanisms of drug resistance among HIV, hepatitis B and hepatitis C virusesViruses201022696273921243082
- KaufmanSHEFuture vaccination strategies against tuberculosis: thinking outside the boxImmunity20103356757721029966
- LallooUGAmbaramANew antituberculosis drugs in developmentCurr HIV/AIDS Rep2010714315120559756
- Signs and symptoms of TB diseaseAtlanta, GACenters for Disease Control and Prevention2010 Available from: http://www.cdc.gov/tb/topic/basics/signsandsymptoms.htmAccessed November 10, 2011
- Tuberculosis: general informationAtlanta, GACenters for Disease Control and Prevention2010 Available from: http://www.cdc.gov/tb/publications/factsheets/general/tb.htmAccessed November 10, 2011
- AhmadSPathogenesis, immunity and diagnosis of latent Mycobacterium tuberculosis infectionClin Dev Immunol2011201181494321234341
- LinPLFlynnJLUnderstanding latent tuberculosis: a moving targetJ Immunol2010185152220562268
- KumarDRaoKVSRegulation between survival, persistence, and elimination of intracellular mycobacteria: a nested equilibrium of delicate balancesMicrobes Infect20111312113320971210
- GasparMCruzAFragaACastroACruzMEMPedrosaJDevelopments on drug delivery systems for the treatment of mycobacterial infectionsCurr Top Med Chem2008857959118473884
- GarnerPSmithHMunroSVolminkJPromoting adherence to tuberculosis treatmentBull World Health Organ20078540440617639229
- AsplerAMenziesDOxladeOBandaJMwengeLGodfrey-FaussettPCost of tuberculosis diagnosis and treatment from the patient perspective in Lusaka, ZambiaInt J Tuberc Lung Dis20081292893518647453
- OnoshitaTShimizuYYamayaNMiyazakiMYokoyamaMFujiwaraNThe behavior of PLGA microspheres containing rifampicin in alveolar macrophagesColloids Surf B Biointerfaces20107615115719954935
- Global summary of the AIDS epidemic2009Geneva, SwitzerlandWorld Health Organization2009 Available from: http://www.who.int/hiv/data/2009_global_summary.pngAccessed November 10, 2011
- WohlDADeconstructing most recent antiretroviral recommendationsCurr HIV/AIDS Rep20107778420425561
- ClercqEDHighlights in the discovery of antiviral drugs: a personal retrospectiveJ Med Chem2010531438145019860424
- ZolopaARThe evolution of HIV treatment guidelines: current state-of- the-art of ARTAntiviral Res20108524124419883695
- GuptaUJainNKNon-polymeric nano-carriers in HIV/AIDS drug delivery and targetingAdv Drug Deliv Rev20106247849019913579
- WatersLNelsonMWhy do patients fail HIV therapyInt J Clin Pract20076198399017504360
- LavanchyDEvolving epidemiology of hepatitis C virusClin Microbiol Infect20111710711521091831
- CastelloGScalaSPalmieriGCurleySAIzzoFHCV-related hepatocellular carcinoma: from chronic inflammation to cancerClin Immunol201013023725019910258
- YuCIChiangBLA new insight into hepatitis C vaccine developmentJ Biomed Biotechnol2010201054828020625493
- ZhangXLJezaVTPanQSalmonella typhi: from a human pathogen to a vaccine vectorCell Mol Immunol20085919718445338
- Typhoid fever: general informationAtlanta, GACenters for Disease Control and Prevention2010 Available from: http://www.cdc.gov/nczved/divisions/dfbmd/diseases/typhoid_feverAccessed November 10, 2011
- TrebichavskyISplichalISplichalovaAInnate immune response in the gut against Salmonella-reviewFolia Microbiol20105529530020526845
- BuchmeierNAHeffronFInhibition of macrophage phagosome-lysosome fusion by Salmonella typhimuriumInfect Immun199159223222382050395
- GuzmanCABorsutzkySGiot-WentMMetcalfeICPearmanJCollioudAVaccines against typhoid feverVaccine2006243804381116278037
- ThaverDZaidiAKMCritchleyJAzmatullahAMadniSAButtaZAA comparison of fluoroquinolones versus other antibiotics for treating enteric fever: meta-analysisBMJ2009338b186519493939
- LawlorCKellyCO’LearySO’SullivanMPGallagherPJKeaneJCellular targeting and trafficking of drug delivery systems for the prevention and treatment of MTbTuberculosis201091939721237714
- Al-KhodorSKwalkYATriggering Ras signaling by intracellular Francisella tularensis through recruitment of PKC-alpha and beta-I to the SOS2/GrB2 complex is essential for bacterial proliferation in the cytosolCell Microbiol2010121604162120618341
- CollinsCABrownEJCytosol as a battle ground: ubiquitination as a weapon for both host and pathogenTrends Cell Biol20102020521320129784
- D’OrsognaMRChouTOptimal cytoplasmic transport in viral infectionsPLoS One2009481658172
- CalderJMStowECStowNDOn the cellular localization of the components of the herpes simplex virus type. 1 helicase-primase complex and the viral origin-binding proteinJ Gen Virol1992735315381312116
- HeuerDLipinskiARMachuyNKarlasAWehrensASiedlerFChlamydia causes fragementation of the golgi compartment to ensure reproductionNature200945773173519060882
- TilneyLGHarbOSConnellyPSRobinsonCGRoyCRHow the parasitic bacterium Legionella pneumophilia modifies its phagosome and transforms it into rough ER: implications for conversion of plasma membrane to the ER membraneJ Cell Sci20011144637465011792828
- CelliJChastellierCFranchiniDMPizaroo-CerdaJMorenoEGorvelJPBrucella evades macrophage killing via VirB-dependent sustained interactions with the endoplasmic reticulumJ Exp Med200319854555612925673
- RoyCRSalcedoSPGorvelJPEPathogen-endoplasmic reticulum interactions: in through the out doorNat Rev immunol2006613614716491138
- MoradpourDGosertREggerDPeninFBlumHEBienzKMembrane association of hepatitis C virus nonstructural proteins and identification of the membrane alteration that harbors the viral replication complexAntiviral Res20036010310914638405
- SinaiAPWebsterPJoinerKAAssociation of host cell endoplasmic reticulum and mitochondria with the Toxoplasma gondii parasitophorous vacuole membrane: a high affinity interactionJ Cell Sci1997110211721289378762
- SandegrenLAnderssonDIBacterial gene amplification: implications for the evolution of antibiotic resistanceNat Rev Microbiol2009757858819609259
- SendiPProctorRAStaphylococcus aureus as an intracellular pathogen: the role of small colony variantsTrends Microbiol200917545819162480
- GarzoniCKellyWLStaphylococcus aureus: new evidence for intracellular persistenceTrends Microbiol200917596519208480
- HudsonMCRampWKNicholsonNCWilliamsASNousiainenMTInternalization of Staphylococcus aureus by cultured osteoblastsMicrob Pathog1995194094198852281
- JevonMGuoCMaBCMordanNNairSPHarrisMMechanisms of internalization of Staphylococcus aureus by cultured human osteoblastsInfect Immun1999672677268110225942
- SinhaBFraunholzMStaphylococcus aureus host cell invasion and post-invasion eventsInt J Med Microbiol201030017017519781990
- EllingtonJHarrisMWebbLSmithBSmithTTanKIntracellular Staphylococcus aureus: a mechanism for the indolence of osteomyelitisJ Bone Joint Surg Br20038591892112931819
- FreitasRAWhat is nanomedicineNanomedicine200512917292052
- Seale-GoldsmithMMLearyJFNanobiosystemsNanomed Nanobiotechnol20091553567
- AsteteCESavliovCMSynthesis and characterization of PLGA nanoparticlesJ Biomater Sci Polymer Edn200617247289
- AvgoutstakisKPegylated poly(lactide) and poly(lactide-co-glycolide) nanoparticles: preparation, properties and possible applications in drug deliveryCurr Drug Deliv2004132133316305394
- LuJMWangXMarin-MullerCWangHLinPHYaoQCurrent advances in research and clinical application of PLGA–based nanotechnologyExpert Rev Mol Diagn2009932534119435455
- PanyamJZhouWZPrabhaSSahooSKLabhasetwarVRapid endolysosomal escape of poly(DL-lactide-co-glycolide) nanoparticles: implications for drug and gene deliveryFASEB J2002161217122612153989
- QaddoumiMGUedaHYangJDavdaJLabhasetwarVLeeVHLThe characteristics and mechanisms of uptake of PLGA nanoparticles in rabbit conjunctival epithelial cell layersPharm Res20042164164815139521
- KohaneDSTseJYYeoYPaderaRShubinaMLangerRBiodegradable polymeric microspheres and nanospheres for drug delivery in the peritoneumJ Biomed Mater Res200677A351361
- WangGLiuSJUengSWChanECThe release of cefazolin and gentamicin from biodegradable PLA/PGA beadsInt J Pharm200427320321215010144
- XiaoRZZengZWZhouGLWangJJLiFZWangAMRecent advances in PEG-PLA block copolymer nanoparticlesInt J Nanomedicine201051057106521170353
- LorenzSHauserCPAutenriethBWeissCKLandfesterKMailanderVThe softer and more hydrophobic the better: influence of the side chain of polymethacrylate nanoparticles for cellular uptakeMacromol Biosci2010101034104220572275
- SajeeshSSharmaCPCyclodextrin-insulin complex encapsulated in polymethacrylic acid based nanoparticles for oral insulin deliveryInt J Pharm200632514715416859846
- JoralemonMJMcRaeSEmrickTPEGylated polymers for medicine: from conjugation to self-assembled systemsChem Comm2010461377139320162127
- VlerkenLEVyasTKAmijiMMPoly(ethylene glycol)-modified nanocarriers for tumor-targeted and intracellular deliveryPharm Res2007241405141517393074
- PanosIAcostaNHerasANew drug delivery systems based on chitosanCurr Drug Discov Technol2008533334119075614
- ParkJHSaravanakumarGKimKKwonICTargeted delivery of low molecular drugs using chitosan and its derivativesAdv Drug Deliv Rev200962284119874862
- KumariAYadavSKYadavSCBiodegradable polymers nanoparticles based drug delivery systemsColloids Surf B Biointerfaces20107511819782542
- SaraogiGKGuptaPGuptaUDJainNKAgrawalGPGelatin nanocarriers as potential vectors for effective management of tuberculosisInt J Pharm200938514314919819315
- HamidiMAzadiARafieiPHydrogel nanoparticles in drug deliveryAdv Drug Deliv Rev2008601638164918840488
- ReisCPNeufeldRJVilelaSRibeiroAJVeigaFReview and current status of emulsions/dispersions technology using an internal gelation process for the design of alginate particlesJ Microencapsul20062324525716801237
- SchiffelersRStormGBakker-WoudenbergILiposome-encapsulated aminoglycosides in pre-clinical and clinical studiesJ Antimicrob Chemother20014833333411532996
- JungSKLimDHJungSHLeeJEJeongKSSeongHAmphotercin B-entrapping lipid nanoparticles and their in vitro and in vivo characteristicsEur J Pharm Sci20093731332019491021
- HuynhNTPassiraniCSaulnierPBenoitJPLipid nanocapsules: a new platform for nanomedicineInt J Pharm200937920120919409468
- PissuwanDCortieCHBalenzuelaSMCortieMBFunctionalized gold nanoparticles for controlling pathogenic bacteriaTrends Biotechnol20102820721320071044
- SeleemMNMunusamyPRanjanAAlqublanHPickrellGSriragnanathanNSilica-antibiotic hybrid nanoparticles for targeting intracellular pathogensAntimicrob Agents Chemother2009534270427419667284
- Izquierdo-BarbaIVallet-RegiMKupferschmidtNTerasakiOSchmidtchenAMalmstenMIncorporation of antimicrobial compounds in mesoporous silica film monolithBiomaterials2009305729573619628277
- RosenholmJMPeuhuEErikssonJESahlgrenCLindenMTargeted intracellular delivery of hydrophobic agents using mesoporous hybrid silica nanoparticles as carrier systemsNano Lett200993308331119736973
- WanXWangDLiuSFluorescent pH-sensing organic/inorganic hybrid mesoporous silica nanoparticles with tunable redox-responsive release capacityLangmuir201026155741557920839827
- VasirJKLabhasetwarVBiodegradable nanoparticles for cytosolic delivery of therapeuticsAdv Drug Deliv Rev20075971872817683826
- DavdaJLabhasetwarVCharacterization of nanoparticle uptake by endothelial cellsInt J Pharm2002233515911897410
- FerratiSMackAChiappiniCLiuXBeanAJFerrariMIntracellular trafficking of silicon particles and logic-embedded vectorsNanoscale201021512152020820744
- MartinsMViveirosMCoutoIAmaralLTargeting human macrophages for enhanced killing of intracellular XDR-TB and MDR-TBInt J Tuberc Lung Dis20091356957319383188
- LecarozCBlanco-PrietoMJBurrellMAGamazoCIntracellular killing of Brucella melitensis in human macrophages with microsphere encapsulated gentamicinJ Antimicrob Chemother20065854955616799160
- KamatMEl-BoubbouKZhuDCLansdellTLuXLiWHyaluronic acid immobilized magnetic nanoparticles for active targeting and imaging of macrophagesBioconj Chem20102121282135
- LeeHFongeHJoangBReillyRMAllenCThe effects of particle size and molecular targeting on the intratumoral and subcellular distribution of polymeric nanoparticlesMol Pharm201071195120820476759
- PassarellaRJSprattDEvan der EndeAEPhillipsJGWuHSathiyakumarVTargeted nanoparticles that deliver a sustained, specific release of paclitaxel to irradiated tumorsCancer Res2010704550455920484031
- RuoslahtiEBhatiaSNSailorMGTargeting of drugs and nanoparticles to tumorsJ Cell Biol201018875976920231381
- VazquezECubasriRUnzuetaURoldanMDomingo-EspanJFerrer-MirallesNInternalization and kinetics of nuclear migration of protein-only, arginine-rich nanoparticlesBiomaterials2010319333933920869766
- HuCMJKaushalSCaoHSTAryalSSartorMEsenerDHalf-antibody functionalized lipid-polymer hybrid nanoparticles for targeted drug delivery to carcinoembryonic antigen presenting pancreatic cancer cellsMol Pharm2010791492020394436
- YanYJohnstonAPRDoddsAJKamphuisMMJFergusonCPartonRGUptake and intracellular fate of disulfide-bonded polymer hydrogel capsules for doxorubicin delivery to colorectal cancer cellsACS Nano201042928293620420377
- WangMThanouMTargeting nanoparticles to cancerPharmacol Res201062909920380880
- NobsLBucheggerFGurnyRAllemannECurrent methods for attaching targeting ligands to liposomes and nanoparticlesJ Pharm Sci20079019801992
- GuFZhangLTeplyBAMannNWangARadovic-MorenoAFPrecise engineering of targeted nanoparticles by using self-assembled biointegrated block copolymersProc Natl Acad Sci U S A20081052586259118272481
- MurthyNCampbellJFaustoNHoffmannASStaytonPSBio- inspired pH-responsive polymers for the intracellular delivery of biomolecular drugsBioconj Chem200314412419
- MkandawireMPohlAGubarevichTLapinaVApplehansDRodelGSelective targeting of green fluorescent nanodiamond conjugates to mitochondria in HeLa cellsJ Biophotonics2009259660619504515
- MisraRSahooSKIntracellular trafficking of nuclear localization signal conjugated nanoparticles for cancer therapyEur J Pharm Sci20103915216319961929
- BallandOPinto-AlphandarHVironAPuvionEAndremontACouvreurPIntracellular distribution of ampicillin in murine macrophages infected with Salmonella typhimurium and treated with (3H) ampicillin-loaded nanoparticlesJ Antimicrob Chemother1996371051158647751
- StavruFBouillaudFSartoriARicquierDCossartPListeria monocytogenes transiently alters mitochondrial dynamics during infectionProc Natl Acad Sci U S A20111083612361721321208
- ZhangLGuFXChanJMWangAZLangerRSFarokhzadOCNanoparticles in medicine: therapeutic applications and developmentsClin Pharmacol Ther20088376176917957183
- LamprechtAUbrichNYamamotoHSchaferUTakeuchiHMaincentPBiodegradable nanoparticles for targeted drug delivery in treatment of inflammatory bowel diseaseJ Pharmacol Exp Ther200129977578111602694
- LecarozCGamazoCRenedoMJBlanco-PrietoMJBiodegradable micro- and nanoparticles as long-term delivery vehicles for gentamicinJ Microencapsul20062378279217123922
- Blanco-PrietoMJLecarozCRenedoMGKunkovaJGamazoCIn vitro evaluation of gentamicin released from microparticlesInt J Pharm200224220320612176247
- PanyamJLabhasetwarVSustained cytoplasmic delivery of drugs with intracellular receptors using biodegradable nanoparticlesMol Pharm20041778415832503
- CloutierMJAntibiotics: mechanisms of action and the acquisition of resistance-when magic bullets lose their magicAm J Pharm Educ199559167172
- WangHXuKLiuLTanJPKChenYLiYThe efficacy of self-assembled cationic antimicrobial peptides nanoparticles against Cryptococcus neoformans for the treatment of meningitisBiomaterial20103128742881
- BuckiRLeszczynskaKNamiotASolokowskiWCalthelicidin LL-37: a multitask antimicrobial peptideArch Immunol Ther Exp2010851525
- EbyDMFarringtonKEJohnsonGRSynthesis of bioinorganic antimicrobial peptide nanoparticles with potential therapeutic propertiesBiomacromolecules2008924812494
- LiuLXuKWangHTanJPKFanWVenkatramanSSSelf- assembled cationic peptide nanoparticles as an efficient antimicrobial agentNat Nanotechnol2009445746319581900
- ChoWMJoshiBPChoHLeeKHDesign and synthesis of novel antibacterial peptide-resin conjugatesACS Med Chem Lett20071757725776
- DurrUHNSudheendraUSRamamoorthyALL-37, the only human member of the cathelicidin family of antimicrobial peptidesBiochim Biophys Acta200617581408142516716248
- LeszczynskaKNamiotAHanmeyPABuckiRModulation of exogenous antibiotic activity by host cathelicidin LL-37APIMS2010118830836
- FonsecaSBPereiraMPKelleySORecent advances in the use of cell-penetrating peptides for medical and biological applicationsAdv Drug Deliv Rev20096195396419538995
- JulianoRLAlamRDixitVKangHMCell-targeting and cell-penetrating peptides for delivery of therapeutic and imaging agentsWiley Interdiscip Rev Nanomed Nanobiotechnol20091332433520049800
- SchmidtNMishraALaiGHWongGCLArginine-rich cell-penetrating peptidesFEBS Lett20105841806181319925791
- MurdayJSSiegelRWSteinJWrightJFTranslational nanomedicine: status assessment and opportunitiesNanomedicine NBM20095251273
- AllhoffFThe coming era of nanomedicineAm J Bioeth200991031119998074