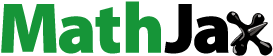
Abstract
Daunorubicin (DNR) has a broad spectrum of anticancer activity, but is limited in clinical application due to its serious side effects. The aim of this study was to explore a novel “smart” pH-responsive drug delivery system (DDS) based on titanium dioxide (TiO2) nanoparticles for its potential in enabling more intelligent controlled release and enhancing chemotherapeutic efficiency of DNR. DNR was loaded onto TiO2 nanoparticles by forming complexes with transition metal titanium to construct DNR-TiO2 nanocomposites as a DDS. DNR was released from the DDS much more rapidly at pH 5.0 and 6.0 than at pH 7.4, which is a desirable characteristic for tumor-targeted drug delivery. DNR-TiO2 nanocomposites induced remarkable improvement in anticancer activity, as demonstrated by flow cytometry, 3-(4,5-dimethylthiazol-2-yl)-2,5-diphenyltetrazolium bromide assay, and nuclear 4′,6-diamidino- 2-phenylindole staining. Furthermore, the possible signaling pathway was explored by western blot. For instance, in human leukemia K562 cells, it was demonstrated that DNR-TiO2 nanocomposites increase intracellular concentration of DNR and enhance its anticancer efficiency by inducing apoptosis in a caspase-dependent manner, indicating that DNR-TiO2 nanocomposites could act as an efficient DDS importing DNR into target cancer cells. These findings suggest that “smart” DNR delivery strategy is a promising approach to cancer therapy.
Introduction
With a broad spectrum of anticancer activity, daunorubicin (DNR) remains the first-line treatment for hematological malignancies and solid tumors. Unfortunately, its therapeutic effect is limited due to its serious side effects, including congestive heart failure,Citation1 which presents a challenge for DNR in clinical application. Therefore, efforts should be made to develop new delivery techniques that reduce its side effects by altering its biodistribution, enhancing its deposition in tumor sites, and improving its therapeutic efficacy.
Recently, a so-called “smart” stimuli-sensitive drug delivery system (DDS) that responds to certain internal or external stimuli, such as pH, temperature, and magnetism, represents a promising approach to improving the efficacy of cancer chemotherapy.Citation2,Citation3 It is well known that the extracellular pH of tumors is slightly more acidic than that of blood and normal tissue. Moreover, numerous pH gradients exist in the intracellular endocytic compartment (pH = 4.5–6.5) where cells uptake a DDS via an endocytosis process.Citation4 Therefore, an ideal anticancer DDS should retain the drug at pH 7.4 but be able to quickly release it at a relatively lower pH (eg, pH 6.0 or 5.0). An important breakthrough in this field is the preparation of a new class of nanosized DDS which can be internalized by tumor cells through the endocytosis pathway to avoid undesired drug release during drug transportation in blood circulation, thus improving effective release of the anticancer drug in tumor tissue or within tumor cells.Citation5
Furthermore, there has been growing interest in metal nanomaterials as anticancer drug carriers recently, which represents a promising approach to tumors, although it is still under investigation.Citation6,Citation7 Barick et al reported a method for entrapping drugs at sites capable of complexing with transition metal ions and suggested that drug release is dependent on the pH of the medium and nature of the materials which encapsulate the drug.Citation7 It is also suggested that this general strategy could be readily extended to other inorganic carriers.Citation7
In view of these developments, the aim of this paper was to explore a novel “smart” pH-responsive DDS based on titanium dioxide (TiO2) nanoparticles that is chemically stable, environmentally friendly, and noncytotoxic.Citation8,Citation9 This DDS could provide a simple and easy way to enable intelligently controlled release of DNR and enhance its chemotherapeutic efficiency. To the best of the authors’ knowledge, no study has been carried out on TiO2 nanoparticles for “smart” stimuli-sensitive DDS and is investigated here for the first time. In this study, DNR was loaded on TiO2 nanoparticles to construct DNR-TiO2 nanocomposites as a DDS. Loading efficiency and influence of pH value on drug release behavior of the DDS were investigated by high-performance liquid chromatography. Additionally, tumor cellular uptake and cytotoxicity of the DDS were evaluated in K562 leukemia cells.
Materials and methods
Chemicals and apparatus
DNR was purchased from Pharmacia Italia SpA (Nerviano, Italy), and its stock solution was freshly prepared and stored in the dark at 4°C. Phosphate buffer solution (PBS) with different pH value (pH 7.4, 6.0, 5.0) was prepared with double distilled water. TiO2 nanoparticles (P25; Evonik Industries AG, Essen, Germany) were suspended in sterilized PBS. Caspase 3 antibody, β-actin antibody, and horseradish peroxidase- conjugated immunoglobulin G antibody were obtained from Nanjing KeyGen Biotech Co, Ltd, (Nanjing, China). 3-(4,5-dimethylthiazol-2-yl)-2,5-diphenyltetrazolium bromide (MTT) and 4,6-Diamidino-2-phenylindole (DAPI) were obtained from Sigma-Aldrich Corporation (St Louis, MO) and stored in the dark. All other reagents were of analytical grades. The images of transmission electron microscope, electron diffraction, and high-resolution transmission electron microscope were obtained by a JEM-2100 transmission electron microscope (JEOL Ltd, Tokyo, Japan). Fluorescent microscopic images were shot on an Olympus IX51 inverted microscope (Olympus Corporation, Tokyo, Japan). Optical density at 492 nm was recorded by multiwell spectrophotometer reader (MK3; Thermo Labsystems, Vantaa, Finland).
Construction of DNR-TiO2 nanocomposites
For a typical drug loading experiment, 2 mL aqueous solution of DNR (2 mg/mL) was added to 1 mL aqueous suspension of TiO2 nanoparticles (10 mg/mL). The above reaction mixture was continuously agitated with a stirrer at 50 g and kept overnight in the dark to construct nanocomposites of DNR-loaded TiO2 nanoparticles which acted as the DDS. DNR-loaded TiO2 nanoparticles were separated from the free-standing drug molecules through centrifugation at 5000 g for 20 minutes and the supernatant was determined by high-performance liquid chromatography, allowing estimation of drug encapsulation efficiency and loading efficiency. Encapsulation efficiency and loading efficiency were calculated by the following equations:
Drug release
Release behavior of DNR from DNR-TiO2 nanocomposites was investigated at pH 5.0 (approximate pH in endosomes or lysosomes), pH 6.0 (pH of the environment around the tumor), and pH 7.4 (pH of physiological blood). DNR-loaded TiO2 nanoparticles (20 mg) were dispersed in PBS (pH 7.4, 5 mL) and transferred into a dialysis bag (Spectra/Por®; Spectrum Laboratories, Inc, Rancho Dominguez, CA). The dialysis bag was then immersed in 95 mL PBS at pH 5.0, 6.0, or 7.4. The release medium was continuously agitated with a stirrer at 50 g and 37°C. At predetermined time intervals, 2 mL of the external medium was collected and replaced with the same fresh PBS. The amount of released DNR in the medium was then determined by high-performance liquid chromatography (LC-310; Skyray Instrument, Nanjing, China).
Cell culture
K562 leukemia cells were obtained from Institute of Hematology, Chinese Academy of Medical Sciences (Beijing, China) and cultured in Gibco® Roswell Park Memorial Institute 1640 medium (Life Technologies, Grand Island, NY) supplemented with 10% heat-inactivated fetal bovine serum (Sigma-Aldrich), 100 U/mL penicillin (Sigma-Aldrich), and 100 μg/mL streptomycin (Sigma-Aldrich) at 37.0°C in humidified air with 5% carbon dioxide. Cells were in log phase prior to the following experiments to minimize experimental variations which occur with proliferative status.
Cell internalization studies
Cells (1 × 105/mL) were seeded in six-well culture plates and grown overnight. They were then treated with either DNR or DNR-TiO2 nanocomposites (DNR concentration 0.5 μg/mL) for 6 hours. To visualize the cellular uptake of DNR with different treatments, the cells were examined by fluorescent microscopy (λex 488 nm, λem 515 nm) after incubation for 6 hours. Furthermore, the cells were resuspended in PBS after being washed three times. DNR uptake was analyzed by FACSCalibur™ flow cytometer (BD Biosciences, San Jose, CA); the gate was arbitrarily set for the detection of green fluorescent DNR.
Assay of anticancer activity
Cytotoxicity of TiO2 nanoparticles, DNR, and DNR-TiO2 nanocomposites was studied in leukemia K562 cells with MTT assays. Cells (1 × 105/mL) were seeded in 96-well plates and incubated for 24 hours. Growth medium was then replaced with 200 μL of the preprepared medium containing either free DNR or DNR-TiO2 nanocomposites (DNR concentration 0, 0.125, 0.25, 0.5, and 1 μg/mL). Cells were treated by TiO2 nanoparticles alone as well to evaluate its cytotoxicity. Cells without any treatment were used as the control group. Cells were further incubated for 48 hours, and relative anticancer activity was assessed with MTT assays. After 48 hours, MTT assay was carried out. In brief, MTT solutions were added after treatment and incubated for an additional 4 hours. Dimethyl sulfoxide (Sinopharm Chemical Reagent Co Ltd, Shanghai, China) was added to solubilize the formazan crystal, and optical density 492 nm was recorded. Cell viability (%) was calculated as follows:
DAPI staining
Cells were treated with the above methods for 48 hours, and were then fixed with 4% polyoxymethylene prior to washing with PBS. The washed cells were then stained with 1 mg/mL DAPI for 15 minutes in the dark. Staining images were recorded with fluorescent microscope.
Western blot analysis
After the different treatments, caspase 3 expression was detected by western blot. In brief, total protein was isolated, subjected to sodium dodecyl sulfate polyacrylamide gel electrophoresis, and transferred to a polyvinylidene fluoride membrane. After being blocked, the membrane was incubated with primary polyclonal antibodies either anti-caspase 3 or anti-β-actin overnight at 4°C, and subsequently incubated with horseradish peroxidase-conjugated immunoglobulin G antibody as the secondary antibody for 1 hour at room temperature. The protein bands were detected by an enhanced electrochemiluminescence detection system (ECL system, Amersham, UK). After normalization by corresponding β-actin expression, protein expression levels of caspase 3 were determined by densitometry scans.
Statistical analysis
All data are presented as mean ± standard deviation. F-test was used for significance testing, and P < 0.05 was considered to be statistically significant. All tests were performed using IBM SPSS software (v 13.0; SPSS Inc, Chicago, IL).
Results and discussion
Characterization of TiO2 nanoparticles
Transmission electron microscopic images of TiO2 nanoparticles are shown in . Observed TiO2 nanoparticles had a spherical shape with a diameter of about 25 nm (). The particles had dimensions suitable for escaping rapid renal excretion, as well as avoiding components of the reticular endothelial system. Thus, potentially passive targeting of drugs to tumors was facilitated via enhanced permeation and retention effect, and drug accumulation in tumor cells after endocytosis was increased.Citation4 Also, the selected-area electron diffraction () patterns confirmed that TiO2 nanoparticles were single crystalline with a hexagonal-wurtzite structure. The fine microstructures of TiO2 nanoparticles were further characterized by high-resolution transmission electron microscope (), which showed that TiO2 nanoparticles were highly crystalline.
DNR-TiO2 nanocomposites as a DDS
A previous study showed that the analog of DNR, doxorubicin, has three potential metal binding sites.Citation7 One is the nitrogen atom in the sugar moiety and the other two are the chelating sites of quinone and phenolic oxygens on both sides of the anthracycline aromatic moiety.Citation7 In the present study, the capacity of TiO2 nanoparticles as a drug carrier for loading DNR was explored. Color change of pure DNR from red to orange was observed during the loading process, indicating that DNR molecules could form complexes with TiO2 nanoparticles. The more likely metal binding sites of DNR were deduced to be the two keto-phenolate functions: C=O and C–O− (six-membered chelate), due to the low affinity of metal ions for the nitrogen atom donor. In addition, DNR, which is positively charged, can be easily self-assembled onto the surface of TiO2 nanoparticles through electrostatic interaction as the relative surface of TiO2 nanoparticles is negatively charged in pH 7.4 aqueous solutions.Citation8,Citation10 Thus, the authors believe that DNR was absorbed onto the TiO2 nanoparticles by forming complexes with transition metal titanium and by electrostatic interaction. shows a proposed schematic representation of DNR loading onto TiO2 nanoparticles through the formation of DNR-TiO2 nanocomposites as a DDS. Encapsulation efficiency and loading efficiency of DNR-loaded TiO2 nanoparticles were assessed and calculated as 65.46% ± 6.82% and 20.63% ± 3.55%, respectively. Results show that DNR-loaded TiO2 nanoparticles may efficiently act as anticancer drug delivery carrier.
pH-triggered drug release behavior
As shown in , release of drug molecules depended on pH of the medium and release time. Drug release at pH 7.4 was slow and sustained, with release ratio at about 18% within 48 hours. However, at lower pH, DNR release rate was much faster, with approximately 67% (pH 6.0) and 86% (pH 5.0) of the drug released within 48 hours. Protonation of the drug (dissociation of DNR-TiO2 nanocomposites) occurred at lower pH, which released chemisorbed drug molecules into the medium. Furthermore, surface charges of TiO2 nanoparticles turned positive at lower pH, which blunted the electrostatic interaction of DNR and TiO2 nanoparticles and facilitated the drug release process. These results demonstrate pH-triggered drug release behavior from the novel DDS, which is of particular interest. It may be hypothesized that most DNR will remain in the carrier for a considerable time period at normal physiological conditions (pH 7.4), indicating the potential for prolonged DNR retention time in blood circulation and thereby greatly reducing the side effects to normal tissues. On the other hand, once DNR-loaded TiO2 nanoparticles are taken up by tumor cells via endocytotic process, a faster release may occur at lower local pH, ie, surrounding the tumor site or inside the endosome and lysosome of tumor cells, leading to significant improvement in cancer treatment efficacy.
Cellular uptake
Fluorescent DNR allows facile observation of the cellular uptake of free DNR and DNR-loaded TiO2 nanoparticles by K562 cells, quantitatively detected by flow cytometry without additional markers. illustrates the typical results of fluorescent microscopy of intracellular DNR. The internalization of DNR loaded onto TiO2 nanoparticles (, right panel) was much higher after 6 hours of incubation compared with the free DNR (, left panel), resulting in stronger fluorescence intensity in K562 cells. The relative intracellular fluorescence intensity of DNR analyzed by flow cytometry allows quantitative comparison of the cases mentioned above (). Intracellular fluorescence intensity for the group of DNR-TiO2 nanocomposites produced 65% ± 3.7% enhancements compared to the group of free DNR. These results clearly show that DNR-TiO2 nanocomposites as a DDS increased the cellular uptake of DNR substantially. TiO2 nanoparticles demonstrated significant properties of a drug carrier, which represents a promising approach to cancer therapy.
Figure 4 Fluorescent microscopic images of K562 cells (A) treated with free daunorubicin (left panel) and titanium dioxide nanoparticles loading daunorubicin as a drug delivery system (right panel). Comparison of the respective average intracellular fluorescence intensity is also shown (B). Concentrations of daunorubicin and titanium dioxide nanoparticles are 0.5 μg/mL and 10 μg/mL, respectively. Note: Data expressed as mean ± standard deviation (n = 3).
Abbreviations: DNR, daunorubicin; Nps, nanoparticles; TiO2, titanium dioxide.
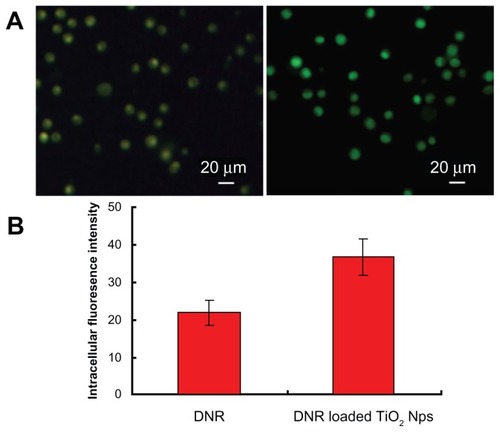
Anticancer activity in vitro
A common characteristic of a nanoparticle-based DDS is the rapid internalization and intracellular localization into acidic endosomes and/or lysosomes of cells.Citation11,Citation12 Due to this common pathway of cellular uptake, there has been significant interest in methods to engineer acid-triggered responses from DDS for rapid intracellular release of therapeutics.Citation13 To further explore the anticancer efficiency of the novel pH-responsive DDS based on TiO2 nanoparticles for DNR, K562 cells were cultured with free DNR at different concentrations (0, 0.125, 0.25, 0.5, and 1 μg/mL) and DNR-TiO2 nanocomposites with equivalent DNR concentration for 48 hours. Cytotoxicity results were estimated by MTT assay ().
Figure 5 Cytotoxic effect of daunorubicin or daunorubicin-titanium dioxide nanocomposites on K562 leukemia cells. Microscopic images of K562 cells after different treatments for 48 hours are shown inset: (A) untreated cells as control, (B) titanium dioxide nanoparticles, (C) daunorubicin alone, and (D) daunorubicintitanium dioxide nanocomposites. Concentrations of daunorubicin and titanium dioxide nanoparticles are 1 μmol/L and 10 μg/mL, respectively.
Note: Data expressed as mean ± standard deviation (n = 3).
Abbreviations: DNR, daunorubicin; DNR-TiO2, daunorubicin-titanium dioxide nanocomposites; Nps, nanoparticles; TiO2, titanium dioxide.
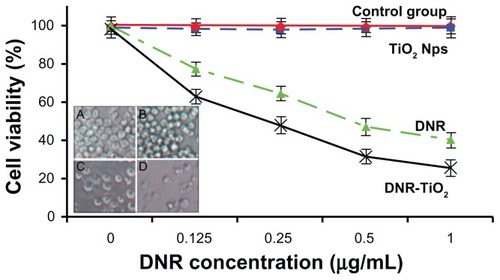
A cytotoxicity test of the nanomaterial is the first-level evaluation before its biomedical applications. When treated by TiO2 nanoparticles, about 95% of the cells survived (, blue line), which is consistent with a previous report.Citation10 The lack of cytotoxicity of TiO2 nanoparticles thus ensures a wide potential range of applications in the field of biomedical science and cancer therapy. Compared with DNR alone (, green line), viability of K562 cells treated by DNR-TiO2 nanocomposites obviously decreases (, black line). Results also indicate that with increasing concentrations of DNR, lethality increased, suggesting a dose-dependent effect in vitro. Increased cytotoxicity may be due to improved DNR cellular uptake by DNR-TiO2 nanocomposites (as illustrated above) and to the induced release of DNR from TiO2.
The process of controlled release is of particular interest in achieving DNR delivery with TiO2. At normal physiological conditions (pH 7.4), it is expected that most DNR conjugated with TiO2 will remain in the carrier for a considerable time period. As well documented, a nanoparticle-based DDS could be internalized through the endocytosis pathway.Citation14 In the endocytic compartment, pH values range 4.5–6.5;Citation15 and at this low pH, protonation of the drug occurs. Protonation will then trigger the release of chemisorbed drug molecules and turn surface charges of TiO2 nanoparticles to positive, blunting the electrostatic interaction of DNR and TiO2 nanoparticles which facilitates the drug release process. Consequently, a faster release of DNR from DNR-TiO2 nanocomposites occurs once the carrier is taken up by the tumor cells via endocytic process. Therefore, a sufficiently high concentration of DNR can be generated within a reasonably short period of time, thereby greatly promoting the cell-killing effects efficiently. Considering that a high dose of DNR may lead to serious side effects, especially congestive heart failure,Citation1 DNR-TiO2 nanocomposites as a DDS improves therapeutic efficacy without a high usage of DNR, thus potentially minimizing its toxic side effects.
As shown in the inset of , optical microscopic observations confirm MTT assay results. K562 cells without any treatment attached to the plate with normal spherical shape (). Notably, cells treated with TiO2 nanoparticles had no morphological changes (). It is obvious that DNR () and the even more effective DNR-TiO2 nanocomposites () could kill more cancer cells and cause more significant morphological changes, indicating an increased probability in cell death.
Morphologic characterization of apoptosis
To explore the mechanism of distinct improvement in anticancer activity induced by the novel pH-responsive DDS, nuclear DAPI staining was performed. To confirm the existence of apoptosis, morphological changes of the cells were studied. Evaluation of normal or apoptotic cells depends on morphological characterization. Normal nuclei (smooth nuclear) and apoptotic nuclei (condensed or fragmented chromatin) were easily distinguished. As shown in , nuclear morphology analysis showed characteristic apoptotic changes, such as chromatin condensation, convoluted nuclei with cavitations, fragmentation of the nucleus, and apoptotic bodies in K562 cells after treatment with DNR and DNR-TiO2 nanocomposites. There was almost no apoptotic evidence in the control group () and TiO2 group (). When cells were treated with DNR-TiO2 nanocomposites (), typical apoptotic morphology was more apparent than that of cells treated with DNR alone (). These findings strongly indicate that DNR-TiO2 nanocomposites killed the cancer cells by inducing apoptosis rather than necrosis.
Figure 6 Nuclear morphologic changes of K562 leukemia cells after different treatments for 48 hours: (A) untreated cells as control, (B) titanium dioxide nanoparticles, (C) daunorubicin alone, and (D) daunorubicin-titanium dioxide nanocomposites. Concentrations of daunorubicin and titanium dioxide nanoparticles are 0.5 μg/mL and 10 μg/mL, respectively.
Notes: Magnification: ×400. Arrows indicate cells with apoptotic nuclear condensation and fragmentation.
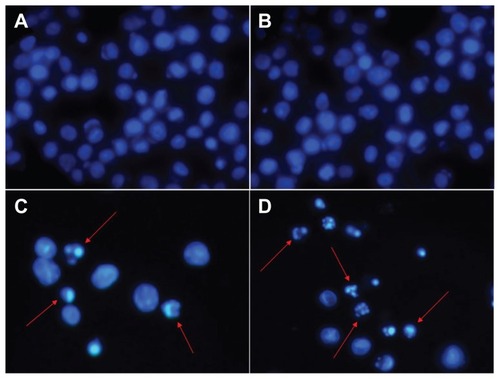
Caspase 3 activity during induced apoptosis
Nuclear DNA is the main subcellular site of DNR action for its anticancer activity. DNR can efficiently accumulate in nucleus, intercalate DNA, and act as a cytostatic and apoptotic agent against tumor cells. To explore the possible signaling pathways through which DNR-TiO2 nanocomposites induced distinct improvement in anticancer activity, the changes in expression levels of the apoptosis-regulating protein, caspase 3, were examined by western blot. As shown in , when K562 cells were treated with DNR and DNR-TiO2 nanocomposites for 48 hours, levels of caspase 3 protein were significantly upregulated compared to control group. Meanwhile, the upregulated levels of caspase 3 protein in DNR-TiO2 nanocomposites group were slightly higher than those in DNR group (P < 0.05). However, the caspase 3 protein was not obviously altered when the cells were treated with TiO2 nanoparticles (P > 0.05). Caspase activation is generally considered to be a key hallmark of apoptosis, and caspase 3 is the main effector caspase that is involved in apoptosis.Citation16 These results indicate that the studied DDS induces distinct improvement in anticancer activity in a caspase-dependent manner.
Figure 7 Expression of caspase 3 in K562 cells by western blotting analysis. After normalization by corresponding β-actin expression, protein expression levels of caspase 3 were determined by densitometry scans to obtain quantitative data. Note: Data expressed as mean ± standard deviation (n = 3). Abbreviations: DNR, daunorubicin; DNR-TiO2, daunorubicin-titanium dioxide nanocomposites; Nps, nanoparticles; TiO2, titanium dioxide.
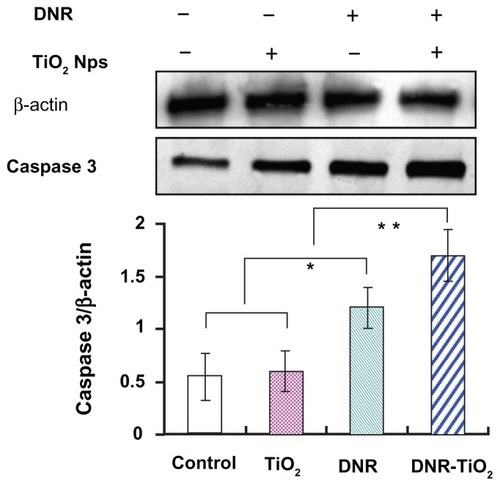
Based on the above studies, schematically illustrates the possible processes that lead to the distinct improvement in anticancer activity. DNR can be easily self-assembled onto the surface of TiO2 nanoparticles by forming complexes with transition metal titanium to construct DNR-TiO2 nanocomposites as a DDS. Drug release shows strong dependence on pH value, which is higher at lower pH. Thereby, TiO2 nanoparticles load an amount of DNR molecules and carry them into cells, increasing intracellular concentration of DNR dramatically, and thus enhancing the suppression of cancer cell proliferation. The therapeutic concentration inside cancer cells is increased, which enhances the efficiency of drugs per dosage and, accordingly, solves some of the limitations of anticancer drugs, thereby exhibiting tremendous application potential in cancer therapy. Finally, apoptosis, a preferred mode of killing cancer cells in cancer therapy, is induced synergistically, resulting in a distinct improvement in anticancer activity.
Figure 8 Schematic illustration of the possible process of distinct improvement in anticancer activity by the novel pH-responsive drug delivery system based on titanium dioxide nanoparticles for daunorubicin.
Abbreviations: DNR, daunorubicin; Nps, nanoparticles; DNR-TiO2, daunorubicin-titanium dioxide nanocomposites; TiO2, titanium dioxide.

Conclusion
In the present study, a pH-responsive nanocomposite composed of self-assembled DNR and TiO2 nanoparticles was developed for a “smart” stimuli-sensitive DDS. Drug release from the DDS was significantly accelerated by decreasing pH from 7.4 to 5.0, which is of particular interest to cancer therapy due to the acidic extracellular tumor environment and intracellular endosomal and/or lysosomal compartments. DNR-TiO2 nanocomposites increased the accumulation of DNR in tumor cells and induced caspase-dependent apoptosis, enhancing anticancer activity and potentially minimizing the toxic side effects of DNR. All these characteristics demonstrate that the novel pH-responsive DDS based on TiO2 nanoparticles for DNR is a promising strategy for clinical practice.
Acknowledgment
This work was supported by the National Key Basic Research Program (2010CB732404) and the National Nature Science Foundation of China (30740062, 30872970).
Disclosure
The authors report no conflicts of interest in this work.
References
- PetreCEDittmerDPLiposomal daunorubicin as treatment for Kaposi’s sarcomaInt J Nanomedicine20072327728818019828
- HidakaMYoshidaRSelf-oscillating gel composed of thermosensitive polymer exhibiting higher LCSTJ Control Release2011150217117621130818
- WuWWChenBAChengJABiocompatibility of Fe3O4/DNR magnetic nanoparticles in the treatment of hematologic malignanciesInt J Nanomedicine201051079108421170355
- ZhangDWZhangHNieJYangJSynthesis and self-assembly behavior of pH-responsive amphiphilic copolymers containing ketal functional groupsPolym Int2010597967974
- XiongXBMaZSLaiRLavasanifarAThe therapeutic response to multifunctional polymeric nano-conjugates in the targeted cellular and subcellular delivery of doxorubicinBiomaterials201031475776819818492
- ZhangHJChenBAJiangHWangCLWangHPWangXMA strategy for ZnO nanorod mediated multi-mode cancer treatmentBiomaterials20113271906191421145104
- BarickKCNigamSBahadurDNanoscale assembly of mesoporous ZnO: a potential drug carrierJ Mater Chem2010203164466452
- SongMZhangRYDaiYYThe in vitro inhibition of multidrug resistance by combined nanoparticulate titanium dioxide and UV irradiationBiomaterials200627234230423816600364
- LiQNWangXMLuXHThe incorporation of daunorubicin in cancer cells through the use of titanium dioxide whiskersBiomaterials200930274708471519500830
- ZhangHJJiangHWangHPZhaoJChenBAWangXMUltrasound mediated drug-loaded nanoparticles crossing cell membranes as a new strategy to reverse cancer multidrug resistanceJ Nanosci Nanotechnol20111131834184021449318
- BarefordLASwaanPWEndocytic mechanisms for targeted drug deliveryAdv Drug Delivery Rev2007598748758
- GilliesERGoodwinAPFréchetJMAcetals as pH-sensitive linkages for drug deliveryBioconjug Chem20041561254126315546191
- LuongAIssarapanichkitTKongSDFongRYangJpH-sensitive, N-ethoxybenzylimidazole (NEBI) bifunctional crosslinkers enable triggered release of therapeutics from drug delivery carriersOrg Biomol Chem20108225105510920820656
- YooHSLeeKHOhJEParkTGIn vitro and in vivo anti-tumor activities of nanoparticles based on doxorubicin-PLGA conjugatesJ Control Release200068341943110974396
- PrabaharanMGrailerJJPillaSSteeberDAGongSQAmphiphilic multi-arm-block copolymer conjugated with doxorubicin via pH-sensitive hydrazone bond for tumor-targeted drug deliveryBiomaterials200930295757576619643472
- GhavamiSHashemiMAndeSRApoptosis and cancer: mutations within caspase genesJ Med Genet200946849751019505876