Abstract
Background
Acceleration of skin regeneration is still an unsolved problem in the clinical treatment of patients suffering from deep burns and scalds. Although erythropoietin (EPO) has a protective role in a wide range of organs and cells during ischemia and after trauma, it has been recently discovered that EPO is not tissue-protective in the common β subunit receptor (βCR) knockout mouse. The protective capacity of EPO in tissue is mediated via a heteroreceptor complex comprising both the erythropoietin receptor (EPOR) and βCR. However, proof of coexpression of these heterogenic receptors in regenerating skin after burns is still lacking.
Methods
To understand the role of nanosized recombinant human erythropoietin (rhEPO) in wound healing, we investigated the effects of subcutaneous injections of EPO on skin regeneration after deep second-degree scalding injuries. Our aim was to determine if joint expression of EPOR and βCR is a prerequisite for the tissue-protective effect of rhEPO. The efficiency in wound regeneration in a skin scalding injury mouse model was examined. A deep second-degree dermal scald injury was produced on the backs of 20 female Balb/c mice which were subsequently randomized to four experimental groups, two of which received daily subcutaneous injections of rhEPO. At days 7 and 14, the mice were sacrificed and the effects of rhEPO were analyzed with respect to grade of re-epithelialization (wound closure) and stage of epidermal maturation. This was investigated using different histological parameters of epithelial covering, such as depth of the epidermal layer, epidermal stratification, and presence of conical and hair follicle structures.
Results
Expression of EPOR, βCR, and growth hormone receptor at the mRNA and protein levels was demonstrated with reverse transcriptase polymerase chain reaction and Western blot analysis. After rhEPO treatment, the rate of re-epithelialization of the scalding injury was increased and the time to final wound closure was reduced. In addition, the quality of regenerated skin was improved. In this investigation, for the first time, we demonstrated coexpression of EPOR and βCR at the RNA and protein levels in vivo using a deep second-degree scalding injury mouse model. These results highlight the potential role of rhEPO in the improved treatment of burns patients, which might be crucial for the development of innovative new therapy regimes.
Conclusion
Local injection of nanosized rhEPO directly to the injury site rather than systemic administration for deep second-degree scalding injuries achieved complete skin regeneration with conical and hair follicle structure via combined expression of EPOR and βCR.
Introduction
Despite modern intensive care for burns patients, there has been no significant improvement of complete skin regeneration with conical and hair follicle structure. The delayed healing process associated with large, deep thermal dermal injuries is often accompanied by complications, such as infections and sepsis; pulmonary, hepatic, or renal failure; loss of bone and muscle mass; poor wound healing; and hormonal imbalance.Citation1 Just recently, Avni et alCitation2 reviewed topical antibiotic application in burns and concluded that there were no beneficial effects. In contrast with topical application of other growth factors used clinically for wound care, recombinant human erythropoietin (rhEPO) plays an important role in revascularization during the early and late stages of injury and increases the capillary density in damaged wound areas. In recent years, several studies have been conducted in which rhEPO was used successfully to stimulate angiogenesis and enhance wound healing, for example, by systemic application in both normal and genetically diabetic mice,Citation3,Citation4 via topical treatment in a diabetic rat model,Citation5 and in our previous study of acute and chronic wounds in humans.Citation6 Moreover, a number of studies have reported that rhEPO affects the proliferation and differentiation of erythroid progenitor cells in hematopoietic organs, as well as nonhematopoietic cell types and organs, and plays a central role in tissue protection.Citation7–Citation11
Although these reports have had encouraging outcomes, the lack of proof of common β subunit receptor (βCR) expression as the relevant tissue-based target for rhEPO in wound repair has been an important but missing link to explain the success of rhEPO in skin wound regeneration. In contrast with the homodimer hematopoietic EPO receptor (EPOR),Citation12 the tissue protective receptor is postulated to be a heterodimer formed by the EPO receptor in assembly with the βCR subunit, otherwise known as CD131, which is also used by other type I cytokines.
In 2004, Brines et alCitation10 found that EPOR and βCR in nonhematopoietic cells together comprise a tissue-protective heteroreceptor as components of receptor-mediated tissue protection instead of only EPOR in the case of cardiac trauma. They evaluated the cytoprotective role of βCR in cardiomyocytes isolated from βCR knockout mice and found that, although there was an adequate presence of EPOR, it was not sufficient to achieve a cytoprotective effect for rhEPO, suggesting that βCR has a vital role in tissue protection to reduce the potential for apoptosis. Further, it has been demonstrated that the heterodimer (EPOR and βCR) is not needed for erythropoiesis in βCR knockout mice; a normal erythropoietic response to rhEPO is existing although these mice lack the heterodimer.Citation13
The tissue-protective role of EPO in skin regeneration has not yet been examined fully.
In 2007, LeBaron et alCitation14 demonstrated that hair follicle dermal papilla fibroblasts are EPO target cells and respond to EPO treatment by activation of EPOR signaling. As mentioned above, in some animal studies after burns injuries,Citation5,Citation15 improvement of skin wound healing and stimulation of neoangiogenesis was analyzed in addition to other parameters, such as expression of endothelial and inducible nitric oxide synthases via detection of vascular endothelial growth factor (VEGF) wound content. However, the tissue-protective coexpression of EPOR and βCR in burn injury models has not been examined thus far. Common protein expression of EPOR and βCR was analyzed by Western blotting in only one experimental study with surgically created ventral hernias in pigs. This protein expression could be demonstrated in wound granulation tissue and wound fluids,Citation16 but not in normal skin, as in our study. Our present study was designed as a mice scalding injury in vivo model to detect the expression of βCR in normal and scalded skin tissue and to evaluate the tissue protective role of rhEPO in such a condition, with a focus on the re-epithelialization potential of subhematopoietic EPO concentrations.
Materials and methods
Animal model
The experiments were performed in adherence with the Guide for the Care and Use of Laboratory Animals and were approved by the Ethics Committee of the University of Lübeck. Twenty female Balb/c mice, (6–8 weeks old, bodyweight 20–25 g, Takomi, Copenhagen, Denmark) were included in these experiments. The animals were kept separately in cages under controlled environmental conditions and had free access to standard laboratory rodent feed and water ad libitum. After thermal injury, the mice were randomly divided into five experimental groups of four animals each. In Groups 1 and 3, mice with dermal wounds received a daily subcutaneous 200 μL injection of 400 IU nanosize rhEPO/kg (NeoRecormon®, Roche Ltd, Mannheim, Germany). In Groups 2 and 4, control animals with dermal wounds received equivalent volumes of vehicle only (distilled water). The treatment duration was 7 days for Groups 1 and 2 and 14 days for groups 3 and 4. In addition, a fifth group of mice without dermal wounds served as controls. EPO administration was carried out according to other studies in mice.Citation15
Scalding injury model
The animals were anesthetized with a mixture of ketamine hydrochloride 10 mg/kg (Bela-Pharm, Vechta, Germany) and xylazine 2.4 mg/kg (Bayer Health Care, Leverkusen, Germany) via intraperitoneal injection; these are suitable anesthetic agents for inducing skin-deep second-degree scalding injuries in a mouse model. After general anesthesia, the hair on the back was shaved and the skin washed and rinsed with sterile water. Creation of a second-degree scald injury was achieved after the area was marked with ink by a 2 × 3 cm window on the back of the mice and immersed in 80°C water for 6 seconds. Mice received 1 ml/kg saline Ringer’s solution immediately after scalding. The scald injury was covered with moist saline dressings to prevent animals from grooming. After thermal injury, the animals were randomized into the five treatment groups and received subcutaneous application of nanosized rhEPO or its vehicle in a blinded fashion. Post- scald sedation and analgesia was provided for the first 7 days with metamizole (1000 mg/L added to the drinking water). At day 7 or 14, respectively, the mice were sacrificed with a barbiturate overdose. The wound tissue or regenerated tissue was obtained for further gene expression, Western blot analyses, and extensive histological studies. The skin was excised from the scalded areas at the back of each mouse, divided into two segments, separately embedded, and frozen in liquid nitrogen as described below.
Histological analysis
All evaluations were performed on routine hematoxylin and eosin stainings because these enable a qualitative description of distinct features of the wound areas and the tissue reaction upon the scald trauma, and morphometric evaluation of distinct parameters, such as presence or absence of re-epithelialization, width of epidermal layer, epidermal stratification, formation of rete ridges, presence of skin appendages (such as hair follicles), and presence of regeneration zones. All criteria used for histological scoring of wound healing are summarized in .
Table 1 Histological scoring of wound regeneration
Gene expression analysis
After preparation of the skin samples, the RNA was obtained by precipitation with isopropanol. After centrifugation, the pellet was washed with 75% ethanol, dried, and diluted in RNase-free water.
RNA was purified and reverses transcribed using standard protocols (Qiagen, Promega, Madison, WI). Target gene products like EPOR (ENSMUST00000006397), βCR (ENS-MUST00000096356), cytokeratin 14 (CK14, ENSMUST00000007272), and glyceraldehyde-3-phosphate-dehydrogenase (GAPDH, ENSMUST00000073605, the housekeeping gene) were amplified by reverse transcriptase polymerase chain reaction using the following intron spanning primers: EPOR forward 5′GCGTTTCTGGTGTTCACTGC-3′ and reverse 5′-GGATGTGGGTGGTCATAGG-3′ (product length 334 base pairs, 40 cycles), βCR forward 5′- -3′ and reverse 5′- -3′ (product length 226 base pairs, 40 cycles), CK14 forward 5′- -3′ and reverse 5′- -3′ (product length 306 base pairs, 30 cycles), Col1 forward 5′- -3′ and reverse 5′- -3′ (product length 244 base pairs, 40 cycles), and GAPDH forward 5′- -3′ and reverse 5′- -3′ (product length 219 base pairs, 30 cycles).
Western blot analysis
Total protein and RNA extraction from the skin samples was performed using the peqGOLD Trifast method according to the manufacturer’s protocol (peqlab, Erlangen, Germany). After skin sample preparation and precipitation of protein, the resulting pellet was dissolved in 1% sodium dodecyl sulfate solution. The concentration of soluble proteins was determined using the bicinchoninic acid assay (Pierce, Rockford, IL). We prepared the gel buffer for electrophoresis which comprises 120 mL and 200 mL of 1× sodium dodecyl sulfate buffer (prepared from 10× sodium dodecyl sulfate buffer, whereby 1 L contained 30 g Tris, 144 g glycine, 15 g sodium dodecyl sulfate) and filled the inner and lower chamber of electrophoresis apparatus. We checked the assembled electrophoresis apparatus with water for leakage. If no leakage is detected in 10 minutes, we removed the water. We inserted a filter paper to remove the remaining water from the electrophoresis apparatus. The wells were washed with sodium dodecyl sulfate buffer. The protein sample (up to 10 μg) solved in 1× sample buffer (2× sample buffer, ie, 2 mL 0.5 M Tris/HCl pH 6.8, 3.6 mL 86% glycerin, 0.31 g sodium dodecyl sulfate, 0.124 g DTT, a pinch of Bromophenol blue, adjusted to 10 mL with distilled water) was heated at 95°C for 5 minutes. Protein samples (20 μg) were analyzed by sodium dodecyl sulfate polyacrylamide gel electrophoresis in a 10% resolving gel at 120 V for one hour using the Thermo Scientific EC Classic Series Submarine gel system (Thermo Fisher Scientific, Schwerte, Germany) and visualized by the Coomassie brilliant blue staining method and then transferred by blotting to a 0.45 μm nitrocellulose membrane (Cell Signaling Technology, Danvers, MA) in cold transfer buffer (48 mM Tris pH 9, 39 mM glycine, 0,037% sodium dodecyl sulfate, 20% methanol). The primary antibodies against EPOR (sc-697), βCR, (sc-678), and growth hormone receptor (sc-20747) were from Santa Cruz Biotechnology (Santa Cruz, CA), against β-actin from Sigma (clone AC-74) and secondary horseradish peroxidase-conjugated antibodies (goat antirabbit immunoglobulin G) were from Dianova (Jackson) ImmunoResearch. The housekeeping β-actin signal, detected with secondary horseradish peroxidase-conjugated antibodies (goat antimouse immunoglobulin G) from Dianova, was used as the control for protein loading. The membranes were blocked with 5% nonfat dry milk in Tris-buffered saline 0.1% Tween-20 and 0.02% NaN3, for one hour at room temperature. The immunoblots were then incubated overnight at 4°C with the primary antibody and for one hour with the secondary antibody, analyzed using an enhanced chemiluminescence system (Cell Signaling Technology), and exposed on a Molecular Imager ChemiDoc XRS system (Bio-Rad, Hercules, CA). Appropriate mouse skin tissue specimens from the scalding experiments were freshly removed, immediately embedded in TissueTec (Jung, Nussloch, Germany), and frozen in liquid nitrogen. Frozen tissue sections of 10–14 μm thickness were cut on a cryotome (Leica CM 3050 S, Nussloch, Germany) and stained with hematoxylin (Mayer’s modification, Roth, Karlsruhe, Germany) and eosin using standard protocols.
The stainings were analyzed by light microscopy and images were taken on an Olympus IX 51 microscope (Olympus, Tokyo, Japan) using a CC12 camera and imaging software of the Olympus cell* family (cell* Imaging Software for Life Sciences Microscopy, Soft Imaging System GMbH, Münster, Germany). The mean values of all sections were allocated to the relevant score values for all individual specimens and all relevant groups, and used for statistical analysis. The scoring data served as a reference for comparison of both experimental groups. The histological scoring system ranged between 0 and 4 for wound closure and 0 and 2 for each of the three partial scores of epithelial covering (). The score values for epithelial covering were summarized (up to 6) and are represented graphically (). The specimens were evaluated in parallel by two independent researchers, including one pathologist, in a blinded manner ().
Comparable sections were evaluated every 100–200 μm depth at low-power (40×) and high-power magnification (100×). All tissue sections for each specimen (≥20) were systematically examined using a modified histological scoring system according to data in the literature.Citation3 The histological parameters were examined and scored as wound closure in the form of assessment of the remaining wound area and re-epithelialization (presence or absence of epithelial covering, degree of epithelial covering relative to the number of epithelial cell layers as a monolayer or multilayer, and presence of conical structures).
In detail, the semiquantitative score for the epithelial covering with the described three parameters was made by systematic analysis. This was done on microscopic visual fields of whole sections with 40× or 100× magnification of each specimen (). The data for every section of the specimen were summarized, and the mean value was calculated and allocated to the relevant partial score value. The sum of the three partial score values was used as the final score value for each animal specimen, and was used for statistical analysis of the entire experimental group. We defined the quantitative score for wound closure to be the main criterion of wound healing, defined as the percentage of the remaining wound area when compared with the total skin area in the microscopic visual field of the section by 40× magnification. These analyses were performed on three random visual fields of each section.
Figure 1 Photographic example of the histological scoring method. The histological parameters of epidermal regeneration of mouse skin scored were (A) the presence or absence of epithelial covering, (B) presence of conical epithelial covering, and (C) degree of epithelial cell layers (monolayer or multilayer).
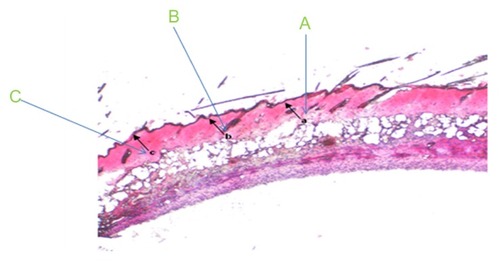
Statistical analysis
The data for the histological scores were analyzed using the unpaired Student’s t-test to compare the different experimental groups. The results are expressed as the mean ± standard error of the mean. Statistical significance was set at P < 0.05.
Results
Histology
The process of wound healing was assessed during the animal’s lifetime as well as macromorphologically after sacrifice. The parameters of skin contraction, tissue regeneration, re-epithelialization, shrinking of the wound area, and wound closure were evaluated and compared between the EPO-treated and control groups. Markedly less skin contraction and considerably better skin regeneration were found as early as day 3 in EPO-treated mice as compared with controls. An overgrowth of the wound area with newly generated skin was observed without EPO treatment. When rhEPO was administered, examination revealed accelerated wound closure and re-epithelialization in contrast with controls. These findings were confirmed by subsequent histological evaluation.
As a first step, routine hematoxylin and eosin stainings 7 and 14 days after the scalding trauma were systematically examined according to the histological score of wound regeneration described above (, ). The results of histological evaluation indicated that treatment with rhEPO led quantitatively and qualitatively to a visible improvement in wound healing with respect to wound closure and epithelial covering (). Specifically, we detected a significant reduction of wound size in the EPO-treated animals, associated with faster wound closure when compared with controls at day 7. In addition, an increase in parameters defined as “presence”, “degree”, “thickness”, and “re-epithelialization” of the epidermal layer was found, but the changes were less pronounced than those related to wound size. At day 14, the EPO group showed significant (P = 0.0129) improvement in overall wound regeneration for the quantitative and qualitative characteristics of epithelial covering. However, the differences in wound closure of both groups were smaller. In the EPO-treated animals, there were more areas with conical structures and hair follicles observed than in controls. Furthermore, a blinded evaluation which was carried out for additional criteria () showed considerable differences between the EPO-treated and untreated animals in both the dermal and epidermal layers. These differences covered the superficial defect zone, marginal epidermal regeneration, skin appendages, and extent and type of inflammation.
Figure 2 Histological scoring of the results of scald regeneration in mice treated with rhEPO or vehicle at different healing times (7 and 14 days). The final score of epithelial covering for each animal specimen is demonstrated as the sum of the three partial score values in .
Notes: Values are the mean ± standard error of the mean of each group. *P < 0.05 versus control group. In the EPO groups, we noticed a significant decrease in wound size and quicker wound closure than in the control groups at day 7. In addition, we found increased epithelial covering in the EPO treatment group than in the control group.
Abbreviations: EPO, erythropoietin; rhEPO, recombinant human erythropoietin.
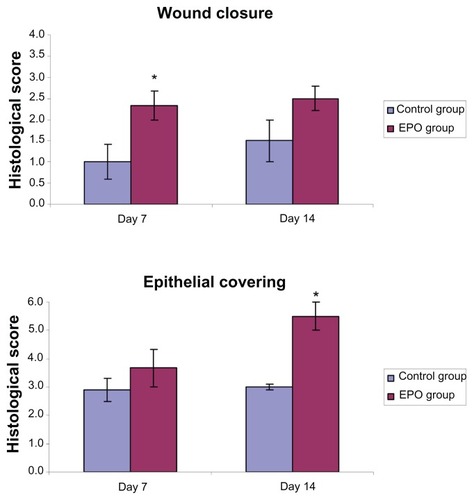
Figure 3 Representative hematoxylin and eosin stainings of scalded skin tissue samples from mice on day 7 after injury and continuous application of rhEPO or vehicle by subcutaneous injection. (A) Control group without EPO (vehicle only), mouse 1 (a–f); (B) EPO group, mouse 2 (a–f) on day 14; (C) control group, mouse 5 (a–f); (D) EPO group, mouse 8 (a–f). Magnification 40× or 100×, scale bar is 500 μm or 200 μm, respectively. In order to obtain a three-dimensional view of the wound area, each 100 μm thickness comparative slides were subjected to qualitative and quantitative evaluation. All evaluations were performed on hematoxylin and eosin routine stainings. The following histological parameters were examined and scored: wound closure in the form of assessment of remaining wound area and re-epithelialization (presence or absence of epithelial covering, degree of epithelial covering relative to number of epithelial cell layers as monolayer or multilayer designed, presence of conical structures). All criteria used for histological scoring of wound healing are summarized in . In order to render the observations comparable, sections every 100–200 μm in depth were evaluated at low-power (40×) and high-power magnification (100×).
Notes: a–f: from a to b; b to c: c to d; d to e; e to f is a 100–200 μm distance of the cryosection.
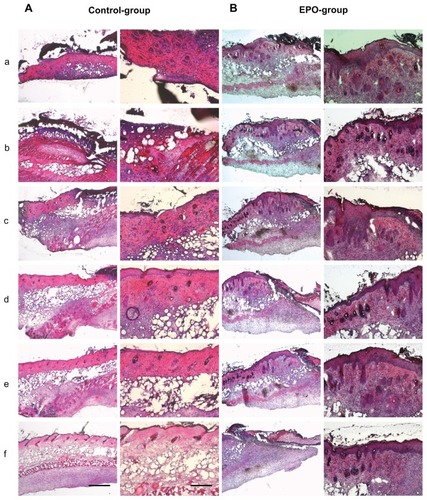

On day 7, the controls showed extensive skin defects, covered by inflammatory exudate, with only little epidermal cell proliferation at the defect margins. The skin appendages, mainly hair follicles, showed significant necrosis or at least major necrobiosis, as evidenced by enhanced apoptotic cell changes. In the central areas of the defect zone, the skin appendages were completely lost. Furthermore, the controls showed strong inflammation with mixed granulocyte-lymphocyte infiltration accumulated at the defect interface, while early granulation tissue was seen at the marginal zone.
Simultaneously, the rhEPO-treated animals showed enhanced epidermal regeneration with a hyperplastic epidermis, moderately sized defect formation, and also moderately expressed mixed inflammation in contrast with the controls. Skin appendages were necrobiotic in the control group (). Fourteen days after scalding, the controls demonstrated partial coverage of the defects by epidermis; skin appendages were slightly regenerating and a strong lymphocytic inflammation was present.
Finally, rhEPO-treated animals showed nearly complete covering of the defect zone by broadened regenerating epidermis along with strong regeneration of the hair follicles. The extent of inflammation was smaller than in the controls (). The control animals showed less extensive recovery of the epidermis and lower levels of re-epithelialization at both assessments (day 7 and day 14). These results suggest that administration of rhEPO led to accelerated wound closure and re-epithelialization as well as skin regeneration. The results of this study suggest the potential efficacy of a subcutaneous EPO injection in accelerating skin regeneration following scalding injuries.
Gene expression
The data presented here show common expression and upregulation of EPOR-βCR in scalded skin during the healing process (). The qualitative and quantitative differences in healing of scalding wounds between the experimental groups could only be evaluated by the histological score (), where we found major differences. Impaired wound healing in the scalds in relation to wound closure (as the main criteria) described above was found in control mice when compared with EPO-treated mice. Furthermore, the quality of the newly formed epithelial covering in the control mice had not improved, even after an extended healing period. In comparison, we found significantly better wound closure (P = 0.0311 at day 7) and quality of re-epithelialization (P = 0.0129 at day 14) in the EPO-treated mice.
Figure 4 Analysis of gene and protein expression of EPOR, βCR, and growth hormone receptor. (A) Reverse transcriptase polymerase chain reaction analysis of gene expression of EPOR, βCR, and growth hormone receptor, and characteristic markers of scalded mouse skin tissue samples on days 7 and 14 after injury and continuous application of rhEPO or its vehicle (control) by subcutaneous injection. DNA bands 1–4 scalded mice of EPO group (+EPO) on day 7; bands 5–8, scalded mice of control group (−EPO, vehicle) on day 7; band 9, nonscalded mouse with EPO application (control); band 10, nonscalded mouse without EPO application (control) on day 7; bands 11–14, scalded mice of EPO group (+EPO) on day 14; bands 15–16, scalded mice of control group (−EPO, vehicle) on day 14; bands 17–19, three additional tissues as comparable controls; band 17, skin nonscalded control; band 18, spleen nonscalded control; band 19, kidney nonscalded control. Polymerase chain reaction products for EPOR, βCR, and other markers were visualized on 1.5% agarose gel with ethidium bromide. (B) Western blot analysis of protein expression of EPOR, βCR, and growth hormone receptor of scalded skin tissue samples of mice on days 7 and 14 after injury and continuous application of rhEPO or its vehicle (control) by subcutaneous injection. Bands 1–4, mice of EPO group (+EPO) on day 7; bands 5–8, mice of control group (EPO, vehicle) on day 7; band 9, nonscalded mouse with EPO application (control); band 10, nonscalded mouse without EPO application (control) on day 7; bands 11–14, mice of EPO group (+EPO) on day 14; bands 15–16, mice of control group (−EPO, vehicle) on day 14; band 17, additional mouse liver tissue as nonscalded comparable control (−EPO). β-actin was used as loading control.
Abbreviations: EPO, erythropoietin; rhEPO, recombinant human erythropoietin; EPOR, erythropoietin receptor; βCR, common β subunit receptor.
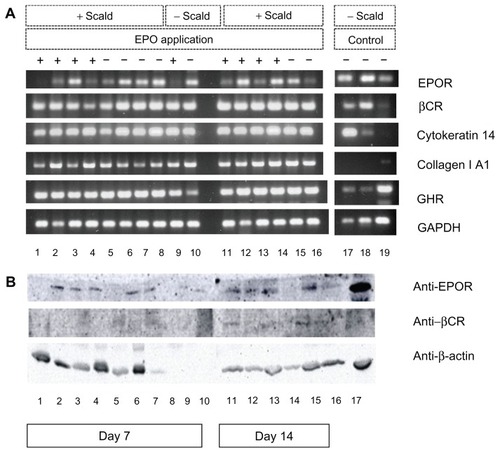
The tissue-protective heterodimer receptor is induced in the case of wounding under hypoxic conditions. However, we found common EPOR-βCR expression also in uninjured skin. According to our previous findings and the evidence of Bodó et al,Citation8 normal human skin expresses EPO and functional EPOR in situ, and human scalp hair follicles are important extrarenal sources and extrahematopoietic targets of EPO-induced signaling. In contrast with the EPOR expression described for injured skin samples and nonscalded skin controls, we found that application of EPO caused a downregulation of EPOR expression in the healthy nonscalded mouse (). This result was consistent with our previous findings.
Protein expression
We investigated protein expression in the tissue-protective EPO receptor, characterized by the heterodimer isoform in combination with βCR, and found slight expression of both receptors in the majority of samples (lower for βCR due to the deficient antibody reaction, ), which confirms the results of our gene expression analysis. Due to some loading problems, the expression of β actin was very negligible in 7–10 of the samples.
In these analyses, we used the antimouse EPOR antibody M-20 from Santa Cruz Biotechnology. Elliott et alCitation19 examined the specificity of common available anti-EPOR antibodies used in the immunoblotting and immunohistochemical analyses of various investigators, and demonstrated that almost all antibodies have limited utility for detecting EPOR because of cross-reactivity with non-EPOR proteins. They concluded that M-20 alone was suitable for detection of EPOR in Western blots, so we used the same in our present study.
Discussion
Normally in skin injuries, EPO is locally produced in a non-hematopoietic manner, and is an effective natural way to achieve adequate wound healing of the injury site, even without any medication. However, a condition such as a second-degree scalding injury involves healing processes additional to those for less traumatic skin injuries, where tissue ischemia may contribute to increased endogenous production of EPO, both locally and systemically. In extensive wound situations, there is a systemic and probably local deficiency of EPO. More important is the fact that a deep second-degree burn is accompanied by destruction of the entire epidermis and also partially the dermal skin structures along with the stem cells contained therein, which causes impaired wound healing and decreased local synthesis of EPO, characterized by delayed formation of granulation tissue, decreased deposition of collagen, and angiogenesis.
This results in large-scale reduction of stem cell numbers in the basal layer, of the hair follicle structures, and of the dermis, which might be directly related to EPO synthesis and its tissue-protective function. Bodó et alCitation8 have previously reported on immunohistological and RNA expression of EPO and EPOR transcripts in the outer root sheath stem cells of the hair follicle and further on EPOR expression in human dermally derived fetal mesenchymal stem cells.Citation6 Therefore, it might be a promising approach to restore the deficiency of EPO using an exogenous supply to enhance healing of dermal thermal injuries.
EPO is a cytokine that has received great attention in the context of wound repair by topical or systemic application in animals as well as in acute and chronic wounds in humans.Citation5,Citation6 So far, no publications could be found concerning the coexpression of βCR in addition to EPOR in dermal thermal injuries. Here we describe for the first time RNA expression of βCR during scald healing and report our results for the detection of EPOR and βCR expression in deep second-degree scalds (). Receptor expression was independent of EPO administration. The EPOR expression level varied between the experimental groups in this study, but did not show a distinct relationship with EPO treatment (). EPOR and βCR expression could only be determined semiquantitatively but not immunohistochemically with respect to localization of EPO-synthesizing structures, probably due to a well known deficient antibody reaction.Citation19
We have previously demonstrated that EPO has an inhibitory effect on skin cells and stem cells in the normal (“healthy”) environment, but the presence of inflammatory cytokines in the case of hypoxic skin conditions changes the function of EPO to one of stimulating stem cell proliferation and differentiation.Citation6,Citation17 We were able to confirm this inhibitory effect of EPO by the above-described gene and protein expression results. Herndon et alCitation18 investigated growth hormone enhancement for re-epithelialization in burns patients and concluded that growth hormone affects wound healing and tissue repair. An aspect of interest is the fact that we found RNA expression of the growth hormone receptor in both intact and injured skin (). In addition to the findings of Bodó et al,Citation8 our present results concerning the tissue-protective role of EPO support the hypotheses about the intracutaneous functions of EPO and the potential role of the skin in the control of EPO production or upregulation in response to hypoxia.Citation20 EPO seems most likely to be a modulator between stem cells and the tissue response, leading to site-specific tissue formation in response to trauma. As a result of our study, we assume that mouse skin stem cells as well as human skin stem cells are the specific responsive elements in uninjured skin containing the heterodimeric EPO receptor and thus show a special readiness for action in the event of traumatic skin injuries, especially thermal trauma.
Recent encouraging evidence suggests that nanosized PEGylated EPO has remarkable advantages over rhEPO.Citation21 Nanosized EPO31-PEG has a five-fold longer terminal half-life and similar biologic activity to that of unmodified rhEPO. The extended terminal half-life of rhEPO confers longer therapeutic benefits, but there is an increased risk of systemic complications with such a long terminal half-life. Generally the half-life of EPO in plasma is only around 5 hours. We preferred to inject rhEPO with its short half-life in patients with local burns injuries so that rhEPO can be degraded by protease without the risk of systemic complications. rhEPO is a nanosized drug because rhEPO is a nanosized highly glycosylated protein comprising 165 amino acids. It has been reported about the three-dimensional structure of other derivatives of rhEPO such as MKLs EPO shows the protein is 25Å×35Å×47Å.Citation22
The potential role of a single intraperitoneal injection of EPO-loaded oligochitosan nanoparticles has been investigated for the treatment for periventricular leukomalacia, and the average diameter of the EPO nanoparticles was 266 nm.Citation23 EPO-loaded oligochitosan nanoparticles were designed to protect EPO from protease digestion and prolong the treatment effect in periventricular leukomalacia by controlling and prolonging the release of EPO. Meloxicam-loaded Eudragit EPO nanoparticles have a particle size of approximately 100 nm.Citation24 Herein, to the best of our knowledge, we show for the first time by both gene and protein expression that normal and scalded skin belong to the tissues that recruit the EPOR/βCR system for tissue protection and that they are an extrahematopoietic site of EPOR expression.
Conclusion
We examined tissue protection as well as skin regeneration via coexpression of EPOR and βCR in a burns injury model. Complete skin regeneration with conical and hair follicle structure at the burned injury site occurred upon local nanosized rhEPO injection. This finding has important clinical implications with regard to the potential use of local subcutaneous injection at the injury site in burns patients. Additionally, we speculated that coexpression of EPOR and βCR constitutes a tissue protective heteroreceptor in the scald healing process. We investigated the role of nanosized rhEPO in tissue regeneration of scalds for the first time by expression of tissue-protective EPOR, characterized as the heterodimer isoform in combination with the βCR on mRNA as well as at protein level. The concept of local subcutaneous injection of rhEPO might be beneficial for prevention and treatment of moderate to severe post-burn patients for complete skin regeneration with conical and hair follicle structure.
Disclosure
The authors report no conflicts of interest in this work.
References
- JeschkeMGChinkesDLFinnertyCCPathophysiologic response to severe burn injuryAnn Surg200824838740118791359
- AvniTLevcovichAAd-ElDDLeiboviciLPaulMProphylactic antibiotics for burns patients: systematic review and meta-analysisBMJ2010340c24120156911
- GaleanoMAltavillaDCucinottaDRecombinant human erythropoietin stimulates angiogenesis and wound healing in the genetically diabetic mouseDiabetes2004532509251715331568
- SorgHKruegerCSchulzTMengerMDSchmitzFVollmarBEffects of erythropoietin in skin wound healing are dose relatedFASEB J2009233049305819403513
- HamedSUllmannYMasoudMHellouEKhamaysiZTeotLTopical erythropoietin promotes wound repair in diabetic ratsJ Invest Dermatol201013028729419626038
- BaderALorenzKRichterAInteractive role of trauma cytokines and erythropoietin and their therapeutic potential for acute and chronic woundsRejuvenation Res201114576621329452
- ParganasEWangDStravopodisDJak2 is essential for signalling through a variety of cytokine receptorsCell1998933853959590173
- BodóEKrommingaAFunkWHuman hair follicles are an extrarenal source and a nonhematopoietic target of erythropoietinFASEB J2007213346335417540710
- JelkmannWMolecular biology of erythropoietinIntern Med2004864965915468961
- BrinesMGrassoGFiordalisoFErythropoietin mediates tissue protection through an erythropoietin and common beta-subunit heteroreceptorProc Natl Acad Sci U S A2004101149071491215456912
- BrinesMCeramiAErythropoietin mediated tissue protection: reducing collateral damage from the primary injury responseJ Intern Med200826440543219017170
- JelkmannWBohliusJHallekMSytkowskiAJThe erythropoietin receptor in normal and cancer tissuesCrit Rev Oncol Hematol200867396118434185
- ScottCLRobbLPapaevangeliouBMansfieldRNicolaNABegleyCGReassessment of interactions between hematopoietic receptors using common beta-chain and interleukin-3-specific receptor beta-chain-null cells: no evidence of functional interactions with receptors for erythropoietin, granulocyte colony stimulating factor, or stem cell factorBlood2000961588159010942411
- LeBaronMJAhonenTJNevalainenMTRuiHIn vivo response-based identification of direct hormone target cell populations using high-density tissue arraysEndocrinology2007148989100817138649
- GaleanoMAltavillaDBittoARecombinant human erythropoietin improves angiogenesis and wound healing in experimental burn woundsCrit Care Med2006341139114616484928
- SaqibNDulinLKrierKHowdieshellTRTemporal and spatial expression of erythropoietin, erythropoietin receptor, and common β receptor in wound fluid and granulationWounds2009217
- LorenzKSickerMSchmelzerEMultilineage differentiation potential of human dermal skin-derived fibroblastsExp Dermatol20081192593218557932
- HerndonDNHawkinsHKNguyenTTPierreECoxRBarrowRECharacterization of growth hormone enhanced donor site healing in patients with large cutaneous burnsAnn Surg19952216496567794069
- ElliottSBusseLBassMBAnti-Epo receptor antibodies do not predict Epo receptor expressionBlood20061071892189516249375
- PausRBodóEKrommingaAJelkmannWErythropoietin and the skin: a role for epidermal oxygen sensingBioessays20093134434819260015
- CohanRAMadadkar-SobhaniAKhanahmadHDesign, modeling, expression, and chemoselective PEGylation of a new nanosize cysteine analog of erythropoietinInt J Nanomedicine201161217122721753873
- CheethamJCSmithDMAokiKHNMR structure of human erythropoietin and a comparison with its receptor bound conformationNat Struct Biol199858618669783743
- WangTHuYLeachMKErythropoietin-loaded oligochitosan nanoparticles for treatment of periventricular leukomalaciaInt J Pharm201242246247122101293
- KhachanePDateAANagarsenkerMSEudragit EPO nanoparticles: application in improving therapeutic efficacy and reducing ulcerogenicity of meloxicam on oral administrationJ Biomed Nanotechnol2011759059721870464