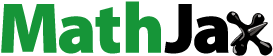
Abstract
Some previous reports have already shown the characterizations of immunomagnetic reduction (IMR). The assay technology involves the utilities of biofunctionalized magnetic nanoparticles to label target biomolecules. However, the detection threshold and interference tests for IMR have not been investigated in detail. In this study, alpha-fetoprotein (AFP) was used as a target biomolecule. The signals for AFP solutions of various concentrations, or with interfering materials, were detected via IMR. These samples were also used for characterizing the detection threshold and interference with enzyme-linked immunosorbent assay (ELISA). The results of assaying AFP level with IMR and ELISA were compared. The detection threshold for assaying AFP with IMR was found to be 3 ng/mL, which is 15 times lower than that of ELISA, and definitely suppresses false negative. For the interfering materials noted commonly in serum such as hemoglobin, bilirubin, triglyceride, and vascular endothelial growth factor, there was no detectable interfering effect when assaying AFP with IMR. Several serum samples from normal people and liver-tumor-bearing patients were used for the detections of AFP concentration via IMR. These results reveal the feasibilities of assaying AFP in blood using IMR, as well as achieving high-sensitive and high-specific assay for AFP.
Introduction
To improve the sensitivity and specificity of immunoassay, the developing trends are to lower the detection threshold and to minimize the cross reaction. Many research groups have improved in-use assay technologies.Citation1–Citation3 Some other groups have explored advanced technologies.Citation4–Citation7
Several years ago, some authors proposed an assay technology called immunomagnetic reduction (IMR).Citation5 In IMR, the reagent is a solution of homogeneously dispersed magnetic nanoparticles, which are coated with hydrophilic surfactants (eg, dextran) and bioprobes (eg, antibodies). Under external multiple alternating-current (ac) magnetic fields, magnetic nanoparticles oscillate with the multiple ac magnetic fields via magnetic interaction (). Thus, the reagents under the external multiple ac magnetic fields show a magnetic property, called mixed-frequency ac magnetic susceptibility χac. With the bioprobes on the outmost shell, magnetic nanoparticles bind with and magnetically label the biomolecules (eg, target biomolecules) to be detected. Due to the binding, magnetic nanoparticles become larger or clustered (). The responses of these larger or clustered magnetic nanoparticles to external multiple ac magnetic fields are much less intense than those of the original individual magnetic nanoparticles. Thus, the χac of the reagent is reduced due to the binding between the magnetic nanoparticles and target biomolecules. This is why the technology is referred to as immunomagnetic reduction. In principle, when greater amounts of to-be-detected biomolecules are mixed with a reagent, more magnetic nanoparticles become larger. Thus, a larger reduction in χac could be expected for the reagents.
Figure 1 Illustration of mechanism of immunomagnetic reduction to detect biotargets. (A) Each magnetic nanoparticle oscillates individually with the applied alternative-current magnetic field before binding with biotargets. (B) Portions of magnetic nanoparticles become larger due to the binding with biotargets. The bound magnetic nanoparticles in (B) contribute to the reduction in the alternative-current magnetic susceptibility of the reagent.
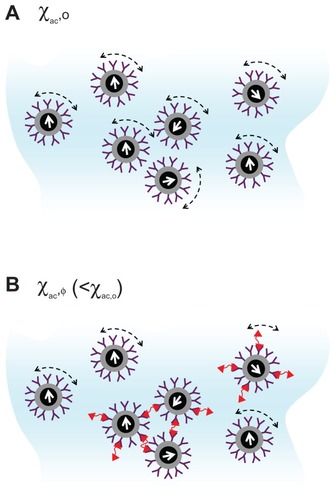
To quantify the reduction in the χac due to the binding between magnetic nanoparticles and biomolecules, this report refers to χac as χac,o for the magnetic reagent without binding with the detected biomolecules, and as χac,φ for the magnetic reagent after the binding between magnetic nanoparticles and biomolecules. The reduction in χac hereafter is defined as:
According to the description given above, IMR exhibits several unique merits. Firstly, it is not necessary to remove the unbound target biomolecules and magnetic nanoparticles. They are still in the reagent. Therefore, the assay process of IMR is simple. Secondly, only one kind of bioprobe is used. Thirdly, IMR is a direct and homogeneous assay, which usually shows high reliability. Fourth, because the amount of reduction in χac can be accurately measured to correspond to the concentration of the to-be-detected biomolecules, the concentration of the biomolecules can thus be measured quantitatively.
Several papers have demonstrated that IMR can be applied to assay proteins,Citation8 viruses,Citation9 chemicals, and nucleic acidsCitation10 once suitable bioprobes are immobilized onto the magnetic nanoparticles. However, investigations of the detection threshold and interference tests of IMR have been very rare. Moreover, few studies have made comparisons between IMR and in-use assays. These uncertainties make it difficult to evaluate the accuracy or feasibilities of using IMR for clinic applications.
This study examined the detection threshold and interference tests for IMR. Alpha-fetoprotein (AFP) was selected as the target biomolecule. To determine the detection threshold for assaying AFP, the relationship between the AFP concentration and IMR (%) was built up experimentally. As to the interference tests, the contributions from the commonly existing biomolecules in human blood to IMR (%) were clarified. All these results were compared with those obtained with certificated assays in current practice, such as enzyme-linked immunosorbent assay (ELISA).
Materials and methods
The reagent for assaying AFP consists of magnetic nanoparticles (MF-DEX-0060, MagQu, New Taipei, Taiwan, Republic of China) functionalized with antibodies (ab40942; Abcam, Cambridge, MA), against AFP (EA502-Q1053; EastCoast Bio, North Berwick, ME).Citation11 The mean diameter of the antibody-functionalized magnetic nanoparticles is 57.3 nm. These magnetic nanoparticles are dispersed in phosphoryl buffer solution (PBS). The concentration of the magnetic bioreagent is 1.2 mg-Fe/mL.
40-μL of magnetic bioreagent was mixed with 60 μL of sample solution in a glass tube. The mixed-frequency ac magnetic susceptibility χac of the mixture was detected as a function of time by using an IMR analyzer (XacPro-E; MagQu). The reduction percentage in χac, denoted as IMR (%), can be determined according to the time dependent χac of the mixture.
The commercial ELISA kit (AF064T; Calbiotech, Spring Valley, CA) for assaying AFP was used in this work. The optical density at the wavelength of 450 nm (OD450) was detected using an ELISA reader (Plus384; Spectra Max, Sunnyvale, CA). The procedures used for AFP assay followed the protocols suggested by the commercial kits.
Various amounts of AFP were spiked into PBS for IMR assay. The concentrations of AFP solution varied from 0.1 ng/mL to 2000 ng/mL. These AFP solutions were used for establishing the AFP concentration-dependent IMR (%).
For interference tests, materials including hemoglobin (Hb) (H7379-1G; Sigma-Aldrich, St Louis, MO), conjugated bilirubin (C-BL) (14370-250MG; Sigma), triglyceride (TG) (T2449-10ML, Sigma), and vascular endothelial growth factor (VEGF) (sc-7269; Santa Cruz Biotechnology, Santa Cruz, CA), were mixed with AFP solutions, respectively. The concentrations of the interference materials used here are listed in . The related diseases and normal concentration levels for these interference materials were also tabulated. The IMR (%) for each sample with interference material was compared with that of pure AFP solution. For each sample, the IMR (%) was detected in triplicate.
Table 1 Information on interference materials used for the IMR and ELISA interference tests in this work
Results and discussion
The experimental relationship between the IMR (%) and AFP concentration φAFP is shown with dots (). The IMR (%) varies from 1.07% to 2.51% as φAFP increases from 0.1 ng/mL to 2000 ng/mL. The dots in are fitted to the following logistic function:
Figure 2 AFP concentration-dependent IMR (%) (solid circles with the solid line) and OD450 (open squares with the dashed line) obtained with IMR and ELISA, respectively.
Note: Points represent mean ± standard deviation.
Abbreviations: AFP, alphafetaprotein; IMR, immunomagnetic reduction; ELISA, enzyme-linked immunosorbent assay; OD, optical density.
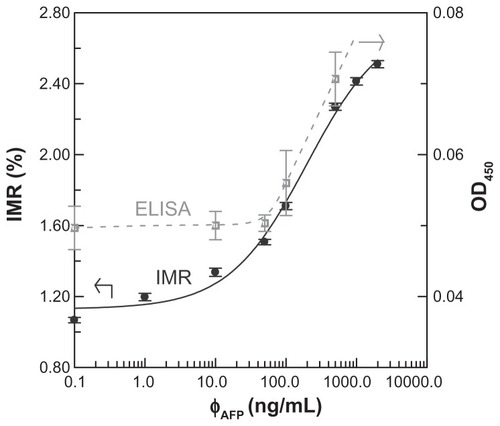
where A, B, φo, and γ are fitting parameters. These parameters are found as A = 1.13, B = 2.76, φo = 197.5, and γ = 0.78. The fitting curve is plotted with the solid line (). The coefficient of determination, R2, between the dots and the fitting curve is 0.994.
These AFP solutions were also used for ELISA assay. The detected OD450 as a function of φAFP is shown with hollow squares (). These hollow squares are guided with a dashed line. It is obvious that OD450 increases significantly as φAFP surpasses 50 ng/mL.
According to the dots (), the IMR (%)–φAFP curve follows the logistic function, as expressed in EquationEquation (2)(2) . The fitting parameter A (= 1.13) in EquationEquation (2)
(2) denotes the noise level for the IMR (%)–φAFP curve. The detection threshold in terms of the detected signal is conventionally defined as that higher than the noise level with triple standard deviations for the detected signal at low concentrations. In this work, the standard deviation of IMR (%) for 1 ng/mL is 0.02%. The detection threshold in terms of IMR (%) is 1.19%. Thus, the detection threshold in terms of AFP concentration φAFP can be determined via EquationEquation (2)
(2) , which results in 3.01 ng/mL. Obviously, the detection threshold is lowered by 15 times when using IMR instead of ELISA. The improvement in the detection threshold for IMR compared with ELISA is attributed from several factors, such as antibodies, uniformity of magnetic particles, and the detecting modules for magnetic signals. From the assay system point of view, IMR definitely shows merits in terms of the detection threshold.
In practice, the reference criteria of the AFP serum level for diagnosis of hepatocellular carcinoma (HCC) are above 20 ng/mL. According to the results (), IMR shows the detection threshold of 3.01 ng/mL, which is much lower than 20 ng/mL. This means that it is highly sensitive (or low false negative) for assaying AFP. To demonstrate this point, six serum samples of early-stage HCC patients were used for the AFP assay with both ELISA and IMR, respectively. The results are shown in . It was found that the three samples showed AFP concentrations lower than 20 ng/mL. This means that a high false negative exists. However, when using IMR, the detected AFP concentrations for these six serum samples were higher than 20 ng/mL. Moreover, all the six samples were positive according to the IMR results. Thus, the ultra-low detection threshold for assaying AFP achieved by IMR definitely suppresses the false negative.
Figure 3 AFP concentration in sera of early-stage HCC patients detected by ELISA and IMR, respectively.
Note: Points represent mean ± standard deviation.
Abbreviations: AFP, alphafetaprotein; HCC, hepatocellular carcinoma; ELISA, enzyme-linked immunosorbent assay; IMR, hepatocellular carcinoma.
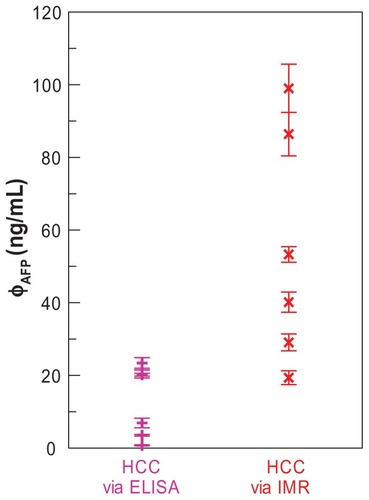
The IMR (%) for AFP solutions without or with interfering materials were detected. The results are shown in . The AFP concentration in each sample is 500 ng/mL. The pure AFP solution does not contain interfering material, and the label for interfering material is “None” for the pure AFP solution (). It is worth noting that all the samples exhibit IMR (%) around 2.27%. No definite deviations in IMR (%) were found among pure AFP solutions and those with interfering materials. Therefore, regardless of what materials were used, Hb, C-BL, TG, or VEGF, no detectable interference was shown for IMR assay on AFP.
Figure 4 Contributions from various kinds of interfering materials to (A) IMR (%) and (B) OD450 measured with IMR and ELISA, respectively. The AFP concentration in each sample is 500 ng/mL.
Notes: The label “None” means there is no interfering material in the 500-ng/mL AFP solution; bars represent mean ± standard deviation.
Abbreviations: AFP, alphafetaprotein; IMR, immunomagnetic reduction; ELISA, enzyme-linked immunosorbent assay; OD, optical density; VEGF, vascular endothelial growth factor.
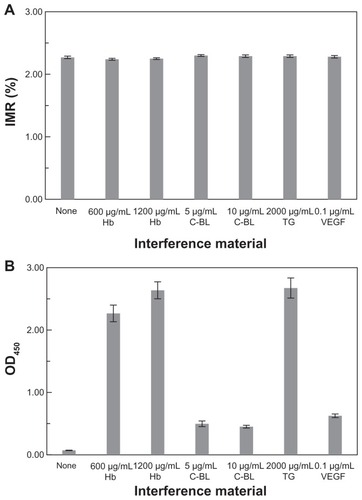
The interference tests for AFP assay with ELISA were also investigated. The OD450 for each of the samples used in are shown in . For the pure AFP solution labeled with “None” in , the OD450 is 0.07 ± 0.003. However, for the AFP solution with interfering materials, the signals of OD450 are higher than 0.45. The results shown in reveal that materials such as Hb, C-BL, TG, and VEGF contribute a lot of interference to OD450 in the ELISA on AFP.
The nonsignificant interference from Hb, C-BL, TG, and VEGF for AFP assay with IMR is shown in . This evidence suggests that a high specificity could be achieved for assaying AFP with IMR. The interference for immunoassay is mainly attributed to sample color and cross reaction. The AFP solution with Hb/C-BL/TG is faintly red/orange/white. The sample color affects the emission/transmission/absorption of fluorescent markers in ELISA. In addition, non-specific binding exists between antibodies and nontarget molecules. Therefore, the OD450 of the AFP solution with interfering materials differs from that of the pure AFP solution. However, the signal detected with IMR is magnetic. The magnetic signals are transparent to any sample color. Hence, the sample color does not cause interference for IMR.
As for the nonspecific binding between the antibodies and nontarget molecules, it is significantly depressed with the centrifugal force acting on the nontarget molecules under ac magnetic fields. The detailed mechanism for the depression in the nonspecific binding for IMR has been clarified in a previous study.Citation12 Briefly speaking, magnetic nanoparticles oscillate with the external ac magnetic fields when detecting the χac of the magnetic reagent. The nontarget molecules originally bound with antibodies on the oscillating magnetic nanoparticles experience centrifugal forces. At high oscillating frequencies, the centrifugal force is enhanced to break down the nonspecific binding. However, it is still weaker than the specific binding force between anti-AFP and AFP molecules. As a result, the cross reactions are inhibited for IMR.
Nineteen serum samples of patients with HCC are used for AFP assay via IMR. The results are shown with cross symbols (). It was found that the AFP concentrations, φAFP, for these patients ranged from 19 to 100 ng/mL. As the AFP concentrations in the serum samples of 17 normal people were detected via IMR, it was found that the AFP concentrations were well below 15 ng/mL, as shown with dots (). The preliminary results shown in evidence the promising applications of IMR in diagnosing hepatocellular carcinoma.
Figure 5 AFP concentrations in sera of normal people and HCC patients. The AFP concentrations are detected via IMR. The sample numbers are 17 for the normal group and 19 for the HCC group.
Note: Points represent mean ± standard deviation.
Abbreviations: AFP, alphafetaprotein; HCC, hepatocellular carcinoma; IMR, immunomagnetic reduction.

Conclusion
In summary, it has been demonstrated that IMR shows the merits of a low detection threshold and nonsignificant interference. The low detection threshold for assaying AFP is improved by 15 times by using IMR instead of ELISA. This ultra-low detection threshold definitely suppresses the false negative, which is usually found for ELISA, when assaying low-concentration AFP at early-stage HCC. It was proven that jaundice, hemolysis, or hypertriglyceridemia did not interfere with the detection of targeted molecules in blood by IMR. Furthermore, the clinic tests for ten samples of human blood using IMR evidence the high sensitivity and high specificity for assaying AFP with IMR. These results reveal the high feasibility for assaying AFP in blood with IMR.
Acknowledgments
This work was supported by the National Science Council of Taiwan under grant numbers 98-2112-M-003-003, 98-2323-B-003-001-CC2, NSC98-02752-M-002-016-PAE, and NSC 100-2120-M-002-015, the Department of Health under grant number DOH99-TD-N-111-008 and DOH100-TD-N-111-008, and the Ministry of Economic Affairs of Taiwan under grant numbers 1Z970688, 1Z0990415 (SBIR), and S09800226-203 (JAID).
Disclosure
The authors report no conflicts of interest in this work.
References
- AllanJCMencosFGarcia-NovalJDipstick dot ELISA for the detection of Taenia coproantigens in humansParasitol19931077985
- MizeTHTabanIDuursmaMA modular data and control system to improve sensitivity, selectivity, speed of analysis, ease of use, and transient duration in an external source FTICR-MSIntl J Mass Spect20042353243253
- LiMAlnoutiYLeverenceRIncrease of the LC–MS/MS sensitivity and detection limits using on-line sample preparation with large volume plasma injectionJ Chrom B20058252152160
- WuCMJianZCJoeSFChangLBHigh-sensitivity sensor based on surface plasmon resonance and heterodyne interferometrySens Actu B Chem2003921–2133136
- HongCYWuCCChiuYCMagnetic susceptibility reduction method for magnetically labeled immunoassayAppl Phys Lett20068821212512-13
- RothackerJRamsayRGCiznadijaDA novel magnetic bead-based assay with high sensitivity and selectivity for analysis of telomerase in exfoliated cells from patients with bladder and colon cancerElectrophoresis200728234435444617987629
- SilvaECGusmãoLAPBarbosaCRHHigh sensitivity giant magnetoimpedance (GMI) magnetic transducer: magnitude versus phase sensingMeas Sci Technol20112233520435208
- HongCYChenWHJianZFWash-free immunomagnetic detection for serum through magnetic susceptibility reductionAppl Phys Lett200790774105-13
- YangSYJianZFChiehJJWash-free, antibody-assisted magnetoreduction assays on orchid virusesJ Virol Methods2008149233433718367255
- YangSYChiehJJWangWCMagnetic nanoparticles for highsensitivity detection on nucleic acids via superconducting-quantuminterference- device-based immunomagnetic reduction assayJ Magn Magn Mater20113236681685
- YangSYJianZFHorngHEDual immobilization and magnetic manipulation of magnetic nanoparticlesJ Magn Magn Mater20083202126882691
- YangSYWangWCLanCBMagnetically enhanced high-specificity virus detection using bio-activated magnetic nanoparticles with antibodies as labeling markersJ Virol Methods20101641–2141819944721