Abstract
Background
Amorphous silica nanoparticles (SiNP) can be used in medical technologies and other industries leading to human exposure. However, an increased number of studies indicate that this exposure may result in cardiovascular inflammation and damage. A high ratio of nitric oxide to peroxynitrite concentrations ([NO]/[ONOO−]) is crucial for cardiovascular homeostasis and platelet hemostasis. Therefore, we studied the influence of SiNP on the platelet [NO]/[ONOO−] balance and platelet aggregation.
Methods
Nanoparticle–platelet interaction was examined using transmission electron microscopy. Electrochemical nanosensors were used to measure the levels of NO and ONOO− released by platelets upon nanoparticle stimulation. Platelet aggregation was studied using light aggregometry, flow cytometry, and phase contrast microscopy.
Results
Amorphous SiNP induced NO release from platelets followed by a massive stimulation of ONOO− leading to an unfavorably low [NO]/[ONOO−] ratio. In addition, SiNP induced an upregulation of selectin P expression and glycoprotein IIb/IIIa activation on the platelet surface membrane, and led to platelet aggregation via adenosine diphosphate and matrix metalloproteinase 2-dependent mechanisms. Importantly, all the effects on platelet aggregation were inversely proportional to nanoparticle size.
Conclusions
The exposure of platelets to amorphous SiNP induces a critically low [NO]/[ONOO−] ratio leading to platelet aggregation. These findings provide new insights into the pharmacological profile of SiNP in platelets.
Introduction
It is increasingly recognized that engineered amorphous silica nanoparticles (SiNP) may have important implications for vascular homeostasis. We have recently demonstrated the ability of SiNP to induce endothelial cell dysfunction linked to increased peroxynitrite (ONOO−) production.Citation1 As human blood platelets along with endothelium play a critical role in vascular homeostasis, we have now examined the biocompatibility of amorphous SiNP (10 nm, 10SiNP; 50 nm, 50SiNP; 150 nm, 150SiNP; 500 nm, 500SiNP) with human platelets.
The vascular NO, synergistically with prostacyclin, inhibits platelet activation and aggregation.Citation2–Citation5 This vasorelaxant and platelet regulator is essential for vascular homeostasis and an impairment of NO bioavailability is one of the earliest events in vascular diseases.Citation6,Citation7 Superoxide anion reacts rapidly with NO producing ONOO−, a major component of oxidative stress, rapidly decreasing NO bioavailability.Citation8 A high ONOO− production and depleted NO availability resulting in a low ratio of maximal NO to maximal ONOO− concentrations ([NO]/[ONOO−]) can be accurately used as an indicator of nitroxidative/oxidative stress and dysfunction of the cardiovascular system.Citation1,Citation9–Citation11 The high [NO]/[ONOO−] balance is essential for maintaining platelet regulation, as high amounts of ONOO− counteract the platelet-inhibitory effects of NO leading to platelet activation and aggregation.Citation12
In the current studies, a direct release of NO and ONOO− during nanoparticle-platelet interactions was measured electrochemically by nanosensors. The NO generation by platelets was followed by a greater ONOO− production leading to a low [NO]/[ONOO−] ratio. Indeed, we demonstrated that amorphous SiNP induced activation of the glycoprotein IIb/IIIa (GPIIb/IIIa) and expression of the selectin P (SELP) on the platelet surface membrane leading to platelet activation and aggregation. The activation of GPIIb/IIIa is triggered off by the release of major platelet activators that mediate pathways of aggregation such as thromboxane A2 (TXA2),Citation13 adenosine diphosphate (ADP)Citation14 and matrix metalloproteinase 2 (MMP2)Citation15 which amplify aggregation. Our studies showed that SiNP induce platelet aggregation via stimulation of ADP and MMP2 released from platelets.
Materials and methods
Reagents
All reagents were purchased from Sigma-Aldrich (Arklow, Ireland) unless otherwise indicated. Engineered amorphous SiNP with different sizes (10 nm, 50 nm, 150 nm, and 500 nm) were purchased from Polysciences (Eppelheim, Germany).
Zeta potential measurement
The zeta potentials of all the SiNP were determined by a Zetasizer Nano ZS (Malvern Instruments, Malvern, UK). Measurements were performed six times for each particle size (100 μg/mL in ultrapure water) at room temperature.
Transmission electron microscopy (TEM) of SiNP
Nanoparticle suspensions (10 μg/mL in ultrapure water) were examined by a JEM 2100 transmission electron microscope (JEOL, Herts, UK). The diameter of 100 nanoparticles of each size on three TEM micrographs was measured by ImageJ an open-source, Java-based imaging software (http://rsbweb.nih.gov/ij/).
Isolation of human platelets
Blood was obtained from healthy volunteers who had not taken any drugs known to affect platelet function for 2 weeks prior to the study. Washed platelets were obtained as described previously.Citation16 Briefly, blood was collected and anticoagulated using trisodium citrate (3.15% w/v; 9:1 v/v). The citrated blood was centrifuged at room temperature to prepare platelet-rich plasma (PRP). Washed platelets were prepared by centrifugation of PRP, containing prostacyclin at room temperature. Following isolation, platelets were resuspended in prostacyclin-free Tyrode’s salt solution. Washed platelet concentrations were adjusted using Tyrode’s salt solution to a final concentration of 2.5 × 108 platelets per milliliter.
Aggregation studies
Immediately before use, commercial stock suspensions of SiNP were sonicated for 2 minutes and nanoparticle dispersions in Tyrode’s salt solution were prepared by serial dilution from these stock suspensions by vortexing. We tested the effect of different SiNP (10SiNP, 50SiNP, 150SiNP and 500SiNP) at different concentrations (1 to 200 μg/mL).
Platelets were incubated for 2 minutes at 37°C in a whole blood/optical lumi-aggregometer (model 700; Chrono-Log, Manchester, UK) prior to the addition of nanoparticles to test spontaneous aggregation. Platelet aggregation was studied for 15 minutes against blanks (Tyrode’s salt solution) containing the same particle concentration as the test tubes. Collagen was used as a positive control for platelet aggregation studies. Aggregation data was analyzed by Chrono-Log AGGRO-LINK 8 sofware.Citation17–Citation19
Selective platelet aggregation inhibitors (acetylsalicylic acid [ASA], phenanthroline, and apyrase) were added to test tubes. After 1 minute, SiNP were added to the appropriate test tubes.
TEM of human platelets
Platelet aggregation was terminated at 30% maximal response, as determined using an aggregometer, in platelets exposed to 10 μg/mL 10SiNP. Unstimulated (resting) platelets were also prepared. Samples were fixed by mixing them with an equal volume of 3% glutaraldehyde in 0.1 M phosphate buffer for 1.5 hours at room temperature. After primary fixation, samples were centrifuged and pellets washed six times with phosphate buffer. Then, pellets were post-fixed with 2% osmium tetroxide in phosphate buffer for 30 minutes. Pellets were then dehydrated in a series of graded ethanol solution, infiltrated and embedded in agar 100 epoxy resin, using propylene oxide as a transitional fluid. Next, ultrathin sections were cut from dried blocks with a diatom diamond knife on a LKB ULTRATOM III (LKB, Uppsala, Sweden), stained with 0.5% aqueous uranyl acetate followed by Reynold’s lead citrate. Finally, sections were examined by a JEM 2100 transmission electron microscope.
Measurement of NO and ONOO− concentrations using nanosensors
Concurrent NO and ONOO− measurements were performed with electrochemical nanosensors (diameter: 300–500 nm). The designs are based on previously developed and well-characterized chemically modified carbon-fiber technology. Citation20,Citation21 Each of the sensors was made by depositing a sensing material on the tip of the carbon fiber: conductive film of polymeric nickel (II) tetrakis (3-methoxy-4hydroxyphenyl) porphyrinic for the NO sensorCitation20 and a polymeric film of Mn (III)–paracyclophanyl-porphyrin for the ONOO− sensorCitation22. Amperometry was used to measure changes in NO and ONOO− concentrations from its basal level with time (detection limit of 1 nM and resolution time <10 μs for each sensor). Linear calibration curves were constructed for each sensor from 5 nM to 3 μM before and after measurements with aliquots of NO and ONOO− standard solutions, respectively. Measurements were performed into platelet suspensions. We tested the effect of different SiNP (10SiNP, 50SiNP, 150SiNP, and 500SiNP) at different concentrations (1 to 200 μg/mL).
Selectin P and GPIIb/IIIa analyses by flow cytometry
Flow cytometry was performed using a FACSArray™ bioanalyzer (Becton, Dickinson and company [BD], Oxford, UK) on single stained platelet samples as described previously.Citation17,Citation23 Briefly, to analyze SELP expression, platelet aggregation was terminated at 30% maximal response, as determined using an aggregometer, in platelet exposed to different SiNP (10SiNP, 50SiNP, 150SiNP, and 500SiNP) at different concentrations (1 to 200 μg/mL). Platelet suspensions and anti-selectin-P antibody (BD) were then mixed and incubated in the dark at room temperature for 5 minutes. Platelets were identified by forward and side scatter signals, and 10,000 platelet-specific events were analyzed by the flow cytometer for fluorescence.
To analyze GPIIb/IIIa activation, platelet aggregation was terminated at 30% maximal response, as determined using an aggregometer, in platelets exposed to different SiNP as above. Platelet suspensions and PAC-1 antibody (BD) were mixed and incubated in the dark at room temperature for 15 minutes. Afterwards, an APC Rat Anti-Mouse IgM (secondary antibody) was added and samples incubated in the dark at room temperature for another 15 minutes. Platelets were identified by forward and side scatter signals, and 10,000 platelet-specific events were analyzed by the flow cytometer for fluorescence.
Nonactivated and activated platelets were gated so as not to analyze platelet aggregates. Silica nanoparticles were not detected by the bioanalyzer.
Statistical analyses
All data are presented as group of means ± standard error of the mean of n > 3. Statistical analysis of the mean difference between multiple groups was determined by one-way ANOVA followed by Tukey-Kramer multiple comparison tests; and between two groups by two-tailed Student’s t-tests. The alpha level for all tests was 0.05. A P value < 0.05 was considered to be statistically significant. All statistical analyses were performed using GraphPad Prism (version 5.00 for Windows; GraphPad Software, San Diego, CA) and Origin (version 6.1 for Windows; OriginLab, Northampton, MA).
Results
Nanoparticle characterization
In order to confirm the commercially-provided nanoparticle size, we used TEM to measure the size of SiNP. The TEM analysis of 10SiNP, 50SiNP, 150SiNP, and 500SiNP resulted in sizes of 10.50 ± 0.19 nm, 52.67 ± 0.66 nm, 148.20 ± 2.19 nm, and 495.90 ± 5.87 nm, respectively.Citation1 Furthermore, Zetasizer reported high negative zeta potentials (more negative than −30 mV) for all SiNP tested in ultrapure water.Citation1
Silica nanoparticle–platelet interaction
We studied nanoparticle–platelet interactions and uptake using TEM (). Electron micrographs showed amorphous SiNP forming agglomerates. These agglomerates were located within platelet aggregates, dispersed among platelets and interacting with the plasma membrane (). Nanoparticles also internalized and distributed into the platelet cytoplasm (). We also observed degranulated platelets indicating platelet activation ().
Figure 1 Amorphous SiNP interact with human platelets. As shown by TEM, after exposure of human platelets to 10SiNP (10 μg/mL), nanoparticles rapidly interacted with the platelet surface membrane (B) and induced aggregation (C and D). Unstimulated (resting) platelets were also presented (A).
Notes: Scale bars represent 500 nm in A, C, and D; and 100 nm in B.
Abbreviations: SiNP, silica nanoparticles; gr, platelet granule; TEM, transmission electron microscopy.

Silica nanoparticles induce a [NO]/[ONOO−] imbalance in platelets
Using electrochemical nanosensors, we examined in situ, real-time dynamic interaction of amorphous SiNP with human platelets by measuring directly NO and ONOO− concentrations in a platelet suspension. We demonstrated with time resolution better than 10 μs that after collision with platelets, these nanoparticles rapidly stimulated NO release () followed by a greater production of ONOO−. An increased ONOO− production was observed when nanoparticle concentration was increased and size decreased (). The exposure of human platelets to SiNP induced an unfavorable shift of the ratio of [NO]/[ONOO−], as nanoparticle size decreased. Indeed, 10 μg/mL 10SiNP induced a greater decrease in [NO]/[ONOO−] than an equal concentration of 50SiNP (). Moreover, 50SiNP (100 μg/mL) induced a lower ratio than an equal concentration of larger nanoparticles ().
Figure 2 Amorphous SiNP induce NO and ONOO− production and unfavorably shift the [NO]/[ONOO−] balance in human platelets. (A) Representative recordings of NO and ONOO− measured in situ by specific nanosensors after the addition of 10 μg/mL 10SiNP to the platelet suspension (2.5 × 108 platelets per milliliter). (B and C) Maximal NO (B) and ONOO− (C) release from a single platelet following stimulation by SiNP: 10SiNP (■), 50SiNP (□), 150SiNP (●), 500SiNP (○). (D and E) The [NO]/[ONOO−] ratio of the maximal NO and ONOO− concentrations measured upon stimulation of platelets by SiNP at 10 μg/mL (D) and 100 μg/mL (E).
Notes: All values are mean ± standard error of the mean (SEM) of n = 4. One-way ANOVA and Tukey-Kramer multiple comparison tests were used for analysis (B and C). All values are mean ± SEM of n = 4. Two-tailed Student’s t-test: ***P < 0.001 compared with 10 μg/mL 10SiNP (D). One-way ANOVA and Tukey-Kramer multiple comparison test: ***P < 0.001 compared with 100 μg/mL 50SiNP (E).
Abbreviations: SEM, standard error of the mean; SiNP, silica nanoparticles.
![Figure 2 Amorphous SiNP induce NO and ONOO− production and unfavorably shift the [NO]/[ONOO−] balance in human platelets. (A) Representative recordings of NO and ONOO− measured in situ by specific nanosensors after the addition of 10 μg/mL 10SiNP to the platelet suspension (2.5 × 108 platelets per milliliter). (B and C) Maximal NO (B) and ONOO− (C) release from a single platelet following stimulation by SiNP: 10SiNP (■), 50SiNP (□), 150SiNP (●), 500SiNP (○). (D and E) The [NO]/[ONOO−] ratio of the maximal NO and ONOO− concentrations measured upon stimulation of platelets by SiNP at 10 μg/mL (D) and 100 μg/mL (E).Notes: All values are mean ± standard error of the mean (SEM) of n = 4. One-way ANOVA and Tukey-Kramer multiple comparison tests were used for analysis (B and C). All values are mean ± SEM of n = 4. Two-tailed Student’s t-test: ***P < 0.001 compared with 10 μg/mL 10SiNP (D). One-way ANOVA and Tukey-Kramer multiple comparison test: ***P < 0.001 compared with 100 μg/mL 50SiNP (E).Abbreviations: SEM, standard error of the mean; SiNP, silica nanoparticles.](/cms/asset/4fe376c1-9031-498f-8bbe-2217275fcf2d/dijn_a_28293_f0002_b.jpg)
Silica nanoparticles induce platelet aggregation
We studied the ability of SiNP to induce platelet aggregation. Our results demonstrate that amorphous SiNP induce activation of GPIIb/IIIa as well as expression of SELP in the platelet surface membrane (). Importantly, platelet receptor activation and expression was inversely proportional to nanoparticle size and directly proportional to particle concentration. Indeed, the smallest nanoparticles (10SiNP) induced GPIIb/IIIa activation and SELP expression when used at a low concentration (10 μg/mL). In contrast, an equal concentration of larger nanoparticles (50SiNP, 150SiNP, and 500SiNP) did not influence platelet receptors. However, when used at a high concentration (100 μg/mL), 50SiNP induced both GPIIb/IIIa activation and SELP expression. Moreover, 200 μg/mL 150SiNP and 500SiNP induced SELP expression.
Figure 3 Amorphous SiNP upregulate surface receptors and induce platelet aggregation. Nanoparticles induce GPIIb/IIIa activation (A), SELP expression (B), and platelet aggregation (C). The use of phenanthroline (Phen) and apyrase (Apyr), but not acetylsalicylic acid (ASA), inhibits 10SiNP-induced platelet aggregation (D). A mixture of these three inhibitors also inhibited nanoparticle-induced platelet aggregation (D). Collagen (Coll) was used as a positive control for platelet aggregation. The GPIIb/IIIa activation (A) and SELP expression (B) induced by each nanoparticle treatment is expressed relative to unstimulated (resting) platelets.
Notes: All values are mean ± SEM of n = 4. One-way ANOVA, Tukey-Kramer post test: *P < 0.05; **P < 0.01; ***P < 0.001 compared with resting platelets (RP) (A–C). One-way ANOVA, Tukey-Kramer post test: ^^P < 0.01; ^^^P < 0.001 compared with 10 μg/mL 10SiNP (D).
Abbreviations: SEM, standard error of the mean; SiNP, silica nanoparticles.
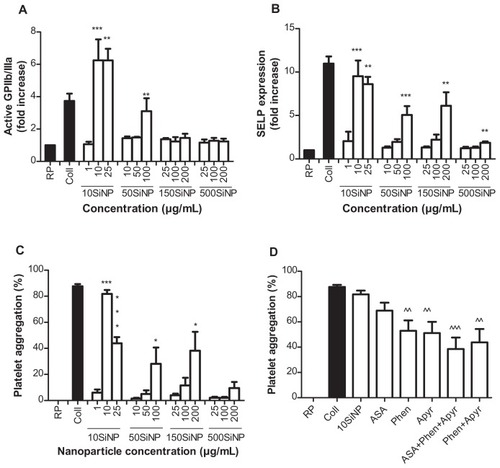
Furthermore, we demonstrated that amorphous SiNP directly induce platelet aggregation and that this effect is greater when nanoparticle size decreases and particle concentration increases (). Indeed, 10SiNP induced platelet aggregation when used at a concentration of 10 μg/mL or higher. Also, 50SiNP and 150SiNP induced platelet aggregation when used at a concentration of 100 μg/mL and 200 μg/mL, respectively. However, 500SiNP did not induce platelet aggregation even when used at a very high concentration of 200 μg/mL.
Knowing that 10SiNP at 10 μg/mL were able to induce platelet aggregation, further experiments with selective inhibitors of the pathways leading to platelet aggregation mediated by TXA2 (ASA), ADP (apyrase), and MMP2 (phenanthroline) were carried out to characterize the mechanism of SiNP-induced platelet aggregation (). Our results show that incubation of platelets with both phenanthroline and apyrase, but not ASA, prior to their exposure to nanoparticles, inhibited nanoparticle-induced platelet aggregation ( and ).
Figure 4 Amorphous SiNP induce platelet aggregation. Platelets were exposed to 10 μg/mL 10-nm SiNP in the absence (C) or present of inhibitors of platelet aggregation (D–G). Unstimulated (resting) platelets (A) and platelet aggregation induced by collagen (B) are also shown. Previous to the exposure to 10SiNP, platelets were incubated with acetylsalicylic acid (D), phenanthroline (E), apyrase (F) and a mixture of these three inhibitors (G).
Note: Scale bars represent 100 μm.
Abbreviation: SiNP, silica nanoparticles.
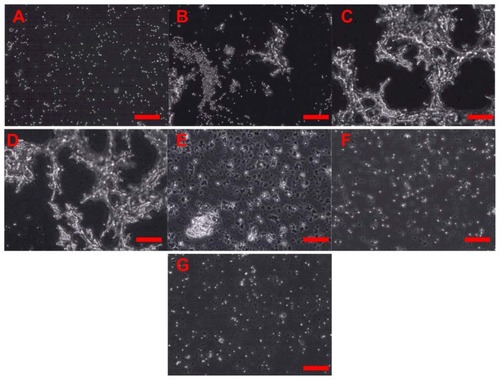
Discussion
Platelets and endothelial cells are crucial for the correct maintenance of vascular homeostasis. To exert their function, platelets survey the vascular system and adhere where alterations of the endothelial cell lining, accompanied or not by exposure of subendothelial matrix components, are detected.Citation24 On the other hand, by the secretion or surface expression of a series of specific molecules (NO, prostacyclin), endothelial cells ensure that under normal conditions blood flow is appropriately regulated and that intravascular platelet activation and blood coagulation are avoided.
The deleterious effect of SiNP on endothelial cells found in our previous workCitation1 prompted us therefore to study the effect of SiNP on human platelets. In this current study, we found that amorphous SiNP when interacting with human blood platelets have the ability to stimulate the release of NO and to a greater extent ONOO− leading to a low [NO]/[ONOO−] ratio that correlated well with the SiNP-induced platelet aggregation. Indeed, we observed that SiNP stimulated GPIIb/IIIa activation and SELP expression and led to platelet aggregation via ADP- and MMP2-dependent mechanisms. All of these effects augmented with increased nanoparticle concentration, but were inversely proportional to the size of SiNP. In fact, the smallest SiNP (10 nm) induced the most severe effects on platelets. Recently, our studies on the effects of amorphous SiNP on human endothelial cells also reported the role of the [NO]/[ONOO−] ratio in the nanoparticle-induced endothelial inflammatory and cytotoxic responses.Citation1
Amorphous SiNP have a noncrystalline structure, a high surface-area-to-volume ratio and a large negative surface charge. A high surface-area-to-volume ratio, which decreases in the opposite direction to size, favors the formation of the “protein corona” with fundamental significance for bionanointeractions. The “protein corona” along with fluid characteristics and particle surface area and zeta potentials can affect the formation of nanoparticle agglomerates in the biological environment and may influence nanoparticle toxicity.Citation25,Citation26
Our TEM study shows that SiNP interacted with the platelet surface membrane, internalized and distributed within the platelet cytoplasm. The interaction of SiNP with platelet surface membranes raised the possibility that nanoparticles can affect calcium channels as well as the endothelial nitric oxide synthase (eNOS) that is associated with caveolae.Citation27 Indeed, there is convincing evidence that modulation of mechanosensitive ion channels involves NO and ONOO−.Citation28 Therefore, we hypothesized that amorphous SiNP collide with the platelet surface membrane leading to opening of calcium channels, and eventually, uncoupling of eNOS. The kinetics of the penetration of the platelet membrane by SiNP most likely involved a flux of calcium ions into the cytoplasm.Citation29 Therefore, SiNP are likely to stimulate a calcium-dependent NO release in human platelets. The process of increased and prolonged NO production leads to depletion of enzymatic substrates and cofactors, and eventually, uncoupling of eNOS.Citation30 The uncoupled eNOS can generate superoxideCitation10 which can rapidly react with NO to produce ONOO− and change critically the balance of [NO]/[ONOO−]. At high level of cytoprotective NO and/or low level of cytotoxic ONOO−, this ratio is high (>2.0) indicating coupled eNOS and normal endothelial and platelet function. A low ratio (<1.0) is associated with uncoupled eNOS, dysfunctional cardiovascular system and high nitroxidative/oxidative stress.Citation1,Citation9,Citation10 Our current experimental results support this hypothesis and show that amorphous SiNP unfavorably shift the [NO]/[ONOO−] balance to low levels. There is convincing evidence that platelet aggregation is favored by nitroxidative/oxidative stress induced by ONOO−.Citation11,Citation12 Indeed, platelet-derived and exogenous ONOO− induce TXA2 formation,Citation31 nitration of tyrosine-containing proteins,Citation32 SELP expression and GPIIb/IIIa activation.Citation12 The activation of GPIIb/IIIa (a change from the low- to high-affinity conformation) and translocation of SELP to the platelet surface membrane is crucial for platelet aggregation to occur.Citation33,Citation34 Indeed, GPIIb/IIIa is the major platelet surface transmembrane receptor and it plays an important role in platelet aggregation. Citation35 In addition, platelets contain a pool of this glycoprotein stored in α-granules.Citation36 Furthermore, SELP, that is also stored in platelet α-granules, mediates platelet-leukocyte aggregation since it is rapidly translocated to the surface upon platelet activation.Citation37 Our studies demonstrate that SiNP have the ability to induce GPIIb/IIIa activation and SELP expression on the platelet surface membrane leading to platelet aggregation. Interestingly, carbon nanoparticles, including nanotubes, have a similar ability.Citation38,Citation39 The activation of GPIIb/IIIa is triggered off by the release of major platelet activators that mediate pathways of aggregation such as TXA2,Citation13 ADP,Citation14 and MMP2Citation15 which amplify aggregation. In fact, we have previously shown that MMP2 translocates to the platelet surface during platelet aggregation.Citation40 Indeed, it has been also demonstrated the interaction of MMP2 with the integrin αIIβIII on the platelet membrane. Citation41 We demonstrate here that SiNP can stimulate platelet aggregation via ADP- and MMP2-dependent mechanisms. However, in contrast to agonist-induced aggregation which can be abolished by combination of ASA, apyrase, and phenanthroline,Citation15 SiNP-induced aggregation is not completely dependent on the release of TXA2, ADP, and MMP2. Interestingly, nanoparticles can directly interact with proteins and ion channels and these effects can also contribute to the profile of SiNP actions on platelets.Citation42,Citation43
In conclusion, engineered amorphous SiNP penetrated the platelet plasma membrane and stimulated a rapid and prolonged NO release leading to eNOS uncoupling, ONOO− over- production and eventually, a low [NO]/[ONOO−] ratio and high nitroxidative/oxidative stress. The unfavorable situation, thus produced, favored receptors activation (GPIIb/IIIa) and expression (SELP) on the platelet surface membrane leading to platelet activation and aggregation. Furthermore, SiNP-induced platelet aggregation is mediated via the MMP2 and ADP pathways. Importantly, these effects were all inversely proportional to the amorphous SiNP size and directly proportional to nanoparticle concentration. These findings, along with our study on endothelial cells,Citation1 conclusively highlight the importance of NO/peroxynitrite imbalance in the pathomechanism of nanotoxicological effects of amorphous SiNP with profound implications in the disruption of vascular homeostasis.
Acknowledgments
This project was funded by a Science Foundation Ireland grant to MWR and a Trinity College Dublin award to JJC. Authors would like to thank Dr Lorraine O’Driscoll, Dr Lidia Tajber, Dr Maria Jose Santos-Martinez, Dr Alan Gaffney, Mr Neal Leddy, Mr Dino Muravec and Ms Neasa Chambers for advice and technical assistance. CM is Science Foundation Ireland Stokes lecturer.
Disclosure
The authors report no conflicts of interest in this work.
References
- CorbalanJJMedinaCJacobyAMalinskiTRadomskiMWAmorphous silica nanoparticles trigger nitric oxide/peroxynitrite imbalance in human endothelial cells: inflammatory and cytotoxic effectsInt J Nanomedicine201162821283522131828
- RadomskiMWPalmerRMMoncadaSThe anti-aggregating properties of vascular endothelium: interactions between prostacyclin and nitric oxideBr J Pharmacol19879236396463322462
- RadomskiMWPalmerRMMoncadaSAn L-arginine/nitric oxide pathway present in human platelets regulates aggregationProc Natl Acad Sci U S A19908713519351971695013
- PalmerRMFerrigeAGMoncadaSNitric oxide release accounts for the biological activity of endothelium-derived relaxing factorNature198732761225245263495737
- MalinskiTRadomskiMWTahaZMoncadaSDirect electrochemical measurement of nitric oxide released from human plateletsBiochem Biophys Res Commun199319429609658343175
- PacherPBeckmanJSLiaudetLNitric oxide and peroxynitrite in health and diseasePhysiol Rev200787131542417237348
- AlonsoDRadomskiMWNitric oxide, platelet function, myocardial infarction and reperfusion therapiesHeart Fail Rev200381475412652159
- PoytonROBallKACastelloPRMitochondrial generation of free radicals and hypoxic signalingTrends Endocrinol Metab200920733234019733481
- HeebaGHassanMKKhalifaMMalinskiTAdverse balance of nitric oxide/peroxynitrite in the dysfunctional endothelium can be reversed by statinsJ Cardiovasc Pharmacol200750439139818049306
- KalinowskiLDobruckiITMalinskiTRace-specific differences in endothelial function: predisposition of African Americans to vascular diseasesCirculation2004109212511251715159296
- VillaLMSalasEDarley-UsmarVMRadomskiMWMoncadaSPeroxynitrite induces both vasodilatation and impaired vascular relaxation in the isolated perfused rat heartProc Natl Acad Sci U S A1994912612383123877809045
- MoroMADarley-UsmarVMGoodwinDAParadoxical fate and biological action of peroxynitrite on human plateletsProc Natl Acad Sci U S A19949114670267067517561
- NeedlemanPMoncadaSBuntingSVaneJRHambergMSamuelssonBIdentification of an enzyme in platelet microsomes which generates thromboxane A2 from prostaglandin endoperoxidesNature19762615561558560934294
- BornGVEffects of adenosine diphosphate (ADP) and related substances on the adhesiveness of platelets in vitro and in vivoBr J Haematol196612137385906266
- SawickiGSalasEMuratJMiszta-LaneHRadomskiMWRelease of gelatinase A during platelet activation mediates aggregationNature199738666256166199121586
- RadomskiMMoncadaSAn improved method for washing of human platelets with prostacyclinThromb Res19833043833896351341
- RadomskiAStewartMWJuraszPRadomskiMWPharmacological characteristics of solid-phase von Willebrand factor in human plateletsBr J Pharmacol200113451013102011682449
- RadomskiAJuraszPSandersEJIdentification, regulation and role of tissue inhibitor of metalloproteinases-4 (TIMP-4) in human plateletsBr J Pharmacol200213781330133812466243
- JuraszPAlonsoDCastro-BlancoSMuradFRadomskiMWGeneration and role of angiostatin in human plateletsBlood200310293217322312855585
- MalinskiTTahaZNitric oxide release from a single cell measured in situ by a porphyrinic-based microsensorNature199235863886766781495562
- BrovkovychVPattonSBrovkovychSKiechleFHukIMalinskiTIn situ measurement of nitric oxide, superoxide and peroxynitrite during endotoxemiaJ Physiol Pharmacol19974846336449444612
- KalinowskiLMalinskiTEndothelial NADH/NADPH- dependent enzymatic sources of superoxide production: relationship to endothelial dysfunctionActa Biochim Pol200451245946915218542
- JuraszPStewartMWRadomskiAKhadourFDuszykMRadomskiMWRole of von Willebrand factor in tumour cell-induced platelet aggregation: differential regulation by NO and prostacyclinBr J Pharmacol200113451104111211682459
- RuggeriZMMendolicchioGLAdhesion mechanisms in platelet functionCirc Res2007100121673168517585075
- LundqvistMStiglerJEliaGLynchICedervallTDawsonKANanoparticle size and surface properties determine the protein corona with possible implications for biological impactsProc Natl Acad Sci U S A200810538142651427018809927
- ThomassenLCAertsARabolliVSynthesis and characterization of stable monodisperse silica nanoparticle sols for in vitro cytotoxicity testingLangmuir201026132833519697952
- NishikawaTIwakiriNKanekoYNitric oxide release in human aortic endothelial cells mediated by delivery of amphiphilic polysiloxane nanoparticles to caveolaeBiomacromolecules20091082074208519583242
- DyachenkoVRueckschlossUIsenbergGModulation of cardiac mechanosensitive ion channels involves superoxide, nitric oxide and peroxynitriteCell Calcium2009451556418639930
- GeldermanMPSimakovaOClogstonJDAdverse effects of fullerenes on endothelial cells: fullerenol C60(OH)24 induced tissue factor and ICAM-I membrane expression and apoptosis in vitroInt J Nanomedicine200831596818488416
- HukINanobashviliJNeumayerCL-arginine treatment alters the kinetics of nitric oxide and superoxide release and reduces ischemia/reperfusion injury in skeletal muscleCirculation19979626676759244241
- SchildknechtSvan der LooBWeberKTiefenthalerKDaiberABachschmidMMEndogenous peroxynitrite modulates PGHS-1- dependent thromboxane A2 formation and aggregation in human plateletsFree Radic Biol Med200845451252018514074
- NaseemKMKhanJJacobsMBruckdorferKRNitration of platelet cytosolic proteins by peroxynitriteBiochem Soc Trans1997253397S9388627
- CollerBSBlockade of platelet GPIIb/IIIa receptors as an antithrombotic strategyCirculation1995929237323807586333
- RadomskiMWRadomskiASRegulation of blood cell function by the endothelial cellsVallancePJTWallanceDJVascular Endothelium in Human Physiology and PathophysiologyLondon, UKHarwood Academic Publishers200095106
- ShattilSJKashiwagiHPamporiNIntegrin signaling: the platelet paradigmBlood1998918264526579531572
- WagnerCLMascelliMANeblockDSWeismanHFCollerBSJordanREAnalysis of GPIIb/IIIa receptor number by quantification of 7E3 binding to human plateletsBlood19968839079148704248
- AndrewsRKBerndtMCPlatelet physiology and thrombosisThromb Res20041145–644745315507277
- RadomskiAJuraszPAlonso-EscolanoDNanoparticle-induced platelet aggregation and vascular thrombosisBr J Pharmacol2005146688289316158070
- BihariPHolzerMPraetnerMSingle-walled carbon nanotubes activate platelets and accelerate thrombus formation in the microcirculationToxicology20102692–314815419698757
- SawickiGSandersEJSalasEWozniakMRodrigoJRadomskiMWLocalization and translocation of MMP-2 during aggregation of human plateletsThromb Haemost19988058368399843180
- ChoiWSJeonOHKimHHKimDSMMP-2 regulates human platelet activation by interacting with integrin alphaIIbbeta3J Thromb Haemost20086351752318088350
- McCarthyJGongXNahirneyDDuszykMRadomskiMPolystyrene nanoparticles activate ion transport in human airway epithelial cellsInt J Nanomedicine201161343135621760729
- CedervallTLynchILindmanSUnderstanding the nanoparticle-protein corona using methods to quantify exchange rates and affinities of proteins for nanoparticlesProc Natl Acad Sci U S A200710472050205517267609