Abstract
Background
Melanoma is known to be radioresistant and traditional treatments have been intractable. Therefore, novel approaches are required to improve the therapeutic efficacy of melanoma treatment. In our study, gold nanorods conjugated with Arg-Gly-Asp peptides (RGD-GNRs) were used as a sensitizer to enhance the response of melanoma cells to 6 mV radiation.
Methods and materials
A375 melanoma cells were treated by gold nanorods or RGD-GNRs with or without irradiation. The antiproliferative impact of the treatments was measured by MTT assay. Radiosensitizing effects were determined by colony formation assay. Apoptosis and cell cycle data were measured by flow cytometry. Integrin αvβ3 expression was also investigated by flow cytometry.
Results
Addition of RGD-GNRs enhanced the radiosensitivity of A375 cells with a dose-modifying factor of 1.35, and enhanced radiation-induced apoptosis. DNA flow cytometric analysis indicated that RGD-GNRs plus irradiation induced significant G2/M phase arrest in A375 cells. Both spontaneous and radiation-induced expressions of integrin αvβ3 were downregulated by RGD-GNRs.
Conclusion
Our study indicated that RGD-GNRs could sensitize melanoma A375 cells to irradiation. It was hypothesized that this was mainly through downregulation of radiation-induced αvβ3, in addition to induction of a higher proportion of cells within the G2/M phase. The combination of RGD-GNRs and radiation needs further investigation.
Introduction
Radiation therapy is one of the commonly utilized treatments for cancer. However, local failure after irradiation remains a challenge due to intrinsic and acquired resistance of tumor cells to radiation treatment. Radiation not only eradicates tumor cells through DNA damage and apoptosis, but also influences cell adhesion to the extracellular matrix by regulating receptors such as integrins.Citation1
Integrins are composed of a single alpha and a single beta chain that have activated or nonactivated conformations. There are eight known beta subunits and 18 alpha subunits that can be combined to form over 24 known mammalian integrins.Citation2 A substantial body of data support the view that integrins, such as αvβ3, play a critical role in regulating tumor growth and metastasis as well as tumor angiogenesis.Citation3,Citation4 αvβ3 is expressed on the surface of a variety of cells, including endothelial cells and certain tumor cells in human malignant melanoma, breast cancer, and advanced glioblastoma. Citation4 Integrin αvβ3 expression not only affects tumor radiosensitivity,Citation5 but is also associated with acquired radioresistance through the upregulation of integrin expression by radiation.Citation6,Citation7 Therefore, targeting integrin αvβ3 has received relatively more attention due to its role as a potential target for inhibition of angiogenesis and tumor growth.Citation3 Arginine (R)-glycine (G)-aspartate (D) (RGD) is the most effective and widely used peptide sequence for stimulating cell adhesion on synthetic material surfaces. The RGD sequence is present in many extracellular matrix proteins and can interact with integrin receptors at focal adhesion points. Once the RGD sequence is recognized and bound to integrins, it initiates an integrin-mediated cell adhesion process and activates signal transduction between the cell and extracellular matrix, thus influencing cell behavior on the substrate (eg, proliferation, differentiation, apoptosis, survival, and migration).Citation8 Cyclic RGD-containing pentapeptides, such as cilengitide, are the most commonly used integrin αvβ3 inhibitors and enhance the response of different cancer cells to irradiation.Citation9,Citation10
Although it is anticipated that RGD peptides will be very promising as radiosensitizers, success in enhancing the radiotherapeutic ratio for cancer depends on increasing tumor cytotoxicity while simultaneously reducing harmful effects on normal tissues. An ideal strategy would be to identify a radiosensitizer that preferentially and effectively enhances the process of radiation-induced cell death in tumor cells which can be coupled with a delivery system that will increase inhibition of tumor tissues in a targeted way without affecting normal tissue. Recently, there have been efforts to develop a nanomedicine that actively targets cancer cells and contains effective anticancer drugs to exploit cancer-specific molecular targets.Citation11 Nanotechnology is an emerging technique for improved cellular targeting and radiosensitization. Gold nanorods are widely used as delivery vehicles to carry chemotherapeutic agents or radiosensitizers to malignant cells in the treatment of cancer.Citation12 Gold nanorods have low cytotoxicity, robust stability, biocompatibility, and suitable physiochemical parameters, and some studies have reported that gold nanorods functionalized with drug molecules show substantially expanded performance as efficient therapeutic drug carriers. The use of structurally modified gold nanorods is less toxic to normal tissue during delivery, and at the molecular level, could traverse biologic barriers and preferentially accumulate in cancer cells.Citation13,Citation14 Achieving the development of a competent cancer nanomedicine may bring new hope to cancer patients.
The present study investigated the benefit of combining RGD-conjugated gold nanorods (RDG-GNRs) that target integrin αvβ3 with irradiation in melanoma cancer cells, and also considered some possible mechanisms for the radiosensitizing effects of RGD-GNRs.
Materials and methods
Preparation of RGD-GNRs
The gold nanorods were synthesized using the seed-mediated template-assisted protocol,Citation15,Citation16 by reducing gold salt in the presence of surfactant-directed synthesis. First, a 20 mL aliquot of gold nanorod stock solution (Sinopharm Chemical Reagent Co, Shanghai, China) was centrifuged and redispersed in 20 mL deionized water (Millipore, Shanghai, China). Then, 1.1 mL of 10 mM tetraethyl orthosilicate ethanol solution (J & K Chemical Ltd, Shanghai, China) was added to the 20 mL of aqueous gold nanorods (pH adjusted to 10–11 by NH4OH). After vigorous stirring for 10 hours at room temperature, an approximately 31 nm thick silica layer formed on the surface of the gold nanorods through hydrolysis and condensation of tetraethyl orthosilicate.Citation17 The silica-coated nanoparticles were isolated by centrifugation, washed with deionized water and ethanol several times, and then dispersed in deionized water for later use. To create molecularly targeted particles, the gold nanorod surfaces were modified by cycle RGD peptides (Shanghai, China) using the standard EDC-NHS reaction as described by Johnsson et al.Citation18 A solution of RGD in deionized water was added to a volume of silica-coated gold nanorods to react for 48 hours and excess RGD peptides were removed by centrifugation at 6000 rpm for 15 minutes. The RGD-GNRs were dispersed in Dulbecco’s Modified Eagle Medium (Hyclone, Carlsbad, CA) and stored at 4°C for later cell experiments.
Cell lines and culture
Human melanoma A375 cells were purchased from the Shanghai Institute of Cell Biology and Chinese Academy of Sciences (Shanghai, China) and grown in flasks containing Dulbecco’s Modified Eagle Medium with 10% heat- inactivated fetal bovine serum (Hyclone). Cells were incubated at 37°C in a humidified atmosphere with 50 mL/L CO2.
Transmission electron microscopic analysis of cells with internalized gold nanorods
A375 cells previously incubated with RGD-GNRs were washed three times with phosphate-buffered saline and fixed with 2.5% glutaraldehyde for six hours. The cells were postfixed in 1% osmium tetroxide for 2 hours, dehydrated in ethanol, and embedded in agar resin (Agar Scientific, Stansted, Essex, UK). Thin sections (60–70 nm) were collected on copper grids, stained with methanol and lead citrate, and visualized using transmission electron microscopy (Philips CM120).
Cellular proliferation assay
Exponentially growing cells were incubated in six-well culture dishes in the presence of RGD-GNRs or gold nanorods alone at different concentrations for a series of times. Then, 15 μL of MTT solution (5 mg/mL; Sigma-Aldrich, Shanghai, China) was added to each well and the cells were incubated for 4 hours. The reaction was stopped by removal of MTT, and 100 μL of dimethylsulfoxide (Sigma-Aldrich) was added to each well to dissolve the formazan crystals, then plates were read at 570 nm. All experiments were repeated three times and measurements were done in triplicate.
Irradiation
Cells in a monolayer were irradiated using 6 mV x-rays from linear accelerators (Siemens, Münich, Germany) at a dose rate of 3 Gy/minute at room temperature. A 1.5 cm bolus was used as a compensator.
Clonogenic assay
A total of about 5 × 105 cells were first seeded and grown in six-well culture plates for 24 hours, and then incubated with RGD-GNRs or gold nanorods alone (50 μg/mL) for one hour prior to irradiation. Next, the cells were detached, seeded, and grown in six-well culture plates for 2 weeks to form colonies. The colonies were stained with 0.4% crystal violet and the count of colonies containing >50 cells was used for the calculation of the surviving fraction (SF). Values were expressed as the mean ± standard deviation. All experiments were repeated three times. Survival curves were fitted to a LQ model using Graphpad Prism software (version 5.0). Survival fraction at 2Gy (SF2) and dose-modifying factor values were used to quantify the radiosensitizing effect of the cells.
Cell cycle and apoptosis assays by flow cytometry
A375 cells (106 cells/mL) fixed in 95% ethanol at −20°C for 24 hours were washed with cold phosphate-buffered saline, resuspended, and stained with propidium iodide (50 μg/mL phosphate-buffered saline; Invitrogen, Shanghai, China) for 15 minutes at 4°C. Analysis was performed using a FACSCalibur flow cytometer (Becton-Dickinson, Franklin Lakes, NJ). Cellular DNA content and cell cycle data were analyzed by flow cytometry using multicycle system 2.0 software. For the apoptosis assay, the cells were stained with Annexin-V-fluorescein isothiocyanate/propidium iodide (Invitrogen) and measured by flow cytometry. All tests were repeated three times.
Integrin αvβ3 analysis
A375 cells were collected and suspended in phosphate-buffered saline/0.2% bovine serum albumin at a concentration of 106 cells/mL. A 200 μL sample of the suspension was incubated with a mouse antihuman integrin αvβ3 mAb LM609 (Millipore, Shanghai, China) or isotype-matched control antibody DD7 (Millipore) for 45 minutes at 4°C. The cells were washed three times with phosphate-buffered saline and analyzed using a FACSCalibur flow cytometer. Specific fluorescence index values were calculated by dividing mean fluorescence obtained with specific Ab by mean fluorescence obtained with isotype control Ab.Citation19
Statistical analysis
SPSS statistical software (v13.0, SPSS Inc, Chicago, IL) was used for the statistical analyses. The results were expressed as the mean ± standard deviation. One-way analysis of variance was performed to compare the means between two test groups, with a P value ≤0.05 considered to be statistically significant.
Results
Cellular toxicity and uptake of RGD-GNRs by A375 cells
Gold nanoparticles were stabilized and grown to linear gold nanorods with the assistance of a reversible adsorption- desorption process of high concentrations of cetyltrimethylammonium bromide (CTAB) surfactant in a reaction medium. However, CTAB-coated gold nanorods (length 44.44 ± 4.7 nm; width 15.10 ± 1.7 nm) do not open any available sites for surface modification and CTAB showed high cell toxicity. For silica coating (thickness about 31 nm, ), surface-occupying CTAB was washed away via multiple centrifugeprecipitation processes.Citation20 The structures of the gold nanorods and the cellular uptake of RGD-GNRs were visualized using transmission electron microscopy. After the A375 cells were incubated with RGD-GNRs for one hour, RGD-GNRs could be located both on the surface of the cell membranes and internalized into A375 cells via integrin αvβ3-receptor-mediated endocytosisCitation21,Citation22 (). MTT assays showed that both the gold nanorods and RGD-GNRs had antiproliferative effects in a dose-dependent manner and that RGD- GNRs were significantly (P < 0.05) more toxic to the cells than the gold nanorods (). To evaluate the ability of gold nanorods and RGD-GNRs to sensitize the cells to radiation, 50 μg/mL of gold nanorods and RGD-GNRs that were slightly toxic after 24 hours in culture () were used for the radiosensitization experiments.
Figure 1 Gold nanorods and internalization by human melanoma A375 cells. (A and B) Nanoparticles shown at different magnifications as viewed by transmission electron microscopy. The gold nanorods were coated with an approximately 31 nm silica layer. (C and D) A375 melanoma cell shows evidence of internalized RGD-GNRs. Gold nanorods were internalized into cells by endocytosis at the cell membrane, and mainly distributed in cytoplasm.
Abbreviations: GNR, gold nanorod; RGD-GNRs, arginine-glycine-aspartate-conjugated gold nanorods.
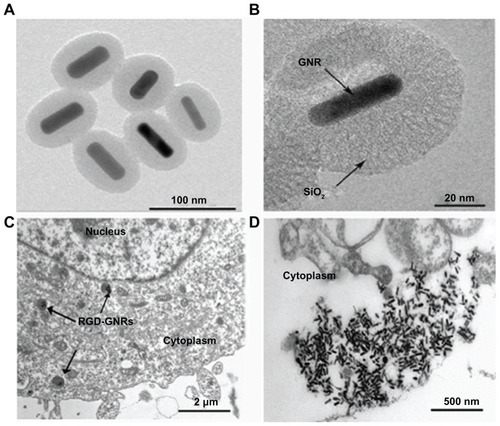
Figure 2 Effects of RGD-GNRs and gold nanorods on cell viability. A375 cells were seeded in 96-well culture plates and incubated in the absence (control) or the presence of the indicated concentrations of gold nanorods or RGD-GNRs for 48 hours (A), or A375 cells were treated with RGD-GNRs or gold nanorods at concentrations of 50 μg/mL for variable times (B). Cell viability was measured by MTT assay and analyzed using an enzyme-labeling analyzer.
Notes: Data were compared with the control group (normalized 1.0) and data points represent the mean survival fraction of three independent experiments done in triplicate; error bars represent the standard deviation.
Abbreviations: GNR, gold nanorod; RGD-GNRs, arginine-glycine-aspartate-conjugated gold nanorods.
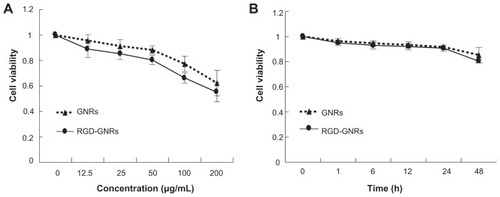
Radiosensitization of melanoma cells by RGD-GNRs
A colony formation assay was used to compare the radiosensitizing effect of gold nanorods and RGD-GNRs in melanoma cells. The dose-response curves of the cells are shown in . After irradiation treatment in combination with either gold nanorods or RGD-GNRs, the radiosensitizing effect was quantified by a dose-modifying factor based on the survival fraction at 2 Gy. Both gold nanorods alone and RGD- GNRs enhanced the radiosensitivity of A375 cells to 6 mV x-rays with a dose-modifying factor (SF2) of 1.14 and 1.35, respectively. These differences were statistically significant compared with radiation alone (P < 0.05). One- way analysis of variance shows that more radiosensitization was observed for cells containing RGD-GNRs compared with cells containing gold nanorods alone (P = 0.001).
Figure 3 Radiosensitizing effect of gold nanorods or RGD-conjugated gold nanorods. A375 cells were treated with either gold nanorods (50 μg/mL) or RGD-GNRs (50 μg/mL) for one hour prior to indicated irradiation. Cells were trypsinized, counted, and seeded at different dilutions. Colonies of >50 cells were counted approximately 2 weeks after treatment.
Notes: The clonogenic surviving fractions were generated with each point representing the mean surviving fraction calculated from three independent experiments done in triplicate for each treatment condition; error bars represent the standard deviation.
Abbreviations: GNR, gold nanorod; RGD-GNRs, arginine-glycine-aspartate-conjugated gold nanorods; RT, radiotherapy.
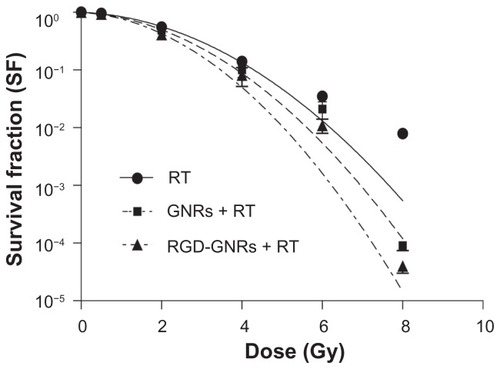
Enhanced radiation-induced apoptosis by RGD-GNRs in melanoma cells
The percentages of A375 cells in apoptosis after the various treatments are shown in . Cellular incorporation of gold nanorods alone or RGD-GNRs did not significantly increase the amount of cell apoptosis compared with controls. Radiation alone or combined with gold nanorods slightly enhanced apoptosis (4.97% ± 0.83% and 7.67% ±0.31%, respectively); there was a statistically significant difference between treated and untreated cells (2.23% ± 0.42%, P < 0.05). However, cells treated with RGD-GNRs plus radiation underwent significantly (P < 0.05) more apoptosis (15.10% ± 0.96%) compared with other cells.
Figure 4 Enhancement of radiation-induced apoptosis by RGD-GNRs. A375 cells were treated with either gold nanorods or RGD-GNRs for one hour prior to irradiation. The cells were stained with Annexin V and propidium iodide, and apoptosis was analyzed by flow cytometry after 24 hours of treatment. (A–C) Apoptosis in control cells and the cells containing gold nanorods (50 μg/mL for 24 hours) or RGD-GNRs (50 μg/mL for 24 hours) without radiation. (D–F) Apoptosis in radiation alone cells and cells containing gold nanorods or RGD-GNRs plus radiation (6 mV x-rays at a dose of 4 Gy). (G) Data from (A–F) were quantified and are presented as the mean ± standard deviation (n = 3 experiments).
Notes: *P < 0.05 compared with control group; **P < 0.05 compared with other treated groups.
Abbreviations: Ctr, Control Group; GNR, gold nanorod; PI, propidium iodide; RGD-GNRs, arginine-glycine-aspartate-conjugated gold nanorods; RT, radiotherapy.
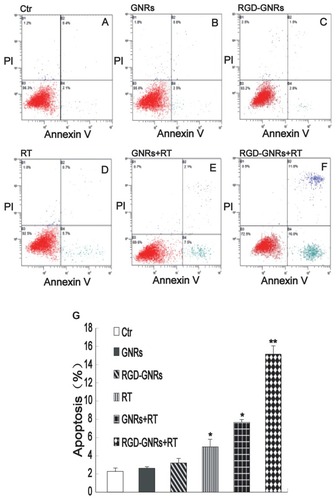
Enhancement of radiation-induced G2 cell cycle arrest by RGD-GNRs
Because the radiosensitivity of tumor cells is cell cycle-oriented, more radiosensitivity was observed in the G2/M phase and less in the G0/G1 or S phase. In , treatment of A375 cells with GNRs, RGD-GNRs, or radiation alone (6 mV x-rays with a dose of 4 Gy) significantly induced cell cycle arrest at the G2/M phase (35% ± 2.65%, 36.14% ± 0.35%, and 40.9% ± 0.35%, respectively). There were significant differences compared with the control cells (25.84% ± 0.49%; P < 0.05), but not among the single treatment groups. The cells treated with a combination of RGD-GNRs plus radiation further enhanced arrest of the cells at the G2/M phase (46.5% ± 1.2%). One-way analysis of variance has also shown that there were significant differences between the RGD- GNRs plus radiation group and both untreated and single treatment groups (P < 0.05).
Figure 5 Enhancement of radiation-induced G2/M cell cycle arrest by RGD-GNRs in A375 cells. A375 cells were incubated with either Dulbecco’s Modified Eagle Medium (controls), gold nanorods (50 μg/mL) for one hour, or RGD-GNRs (50 μg/mL) for one hour without or with irradiation (6 mV x-rays with a dose of 4 Gy), and then the cells were stained with propidium iodide, and analyzed by flow cytometry 24 hours after radiation. (A) Percentage in different cell phases. Histograms represent the mean ± standard deviation (n = 3 experiments). (B) Flow cytometry analysis for the different treatment groups.
Notes: *P < 0.05 compared with the control group; **P < 0.05 compared with radiotherapy alone group.
Abbreviations: Ctr, Control Group; GNR, gold nanorod; RGD-GNRs, arginine-glycine-aspartate-conjugated gold nanorods; RT, radiotherapy.
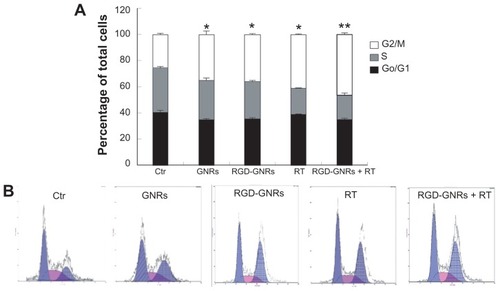
Integrin αvβ3 expression levels and effect of irradiation and RGD-GNRs
Radiation induced a significant expression of integrin αvβ3 in a dose-dependent manner (). Incubating the cells with GNRs did not impact the expression of integrin αvβ3, but RGD-GNRs could effectively reduce the spontaneous expression of integrin αvβ3 compared with the results of the untreated cells (P < 0.05). While the cells incubated with RGD-GNRs one hour prior to radiation at 4 Gy, RGD-GNRs could inhibit both spontaneous and radiation-induced αvβ3 expression, while untreated or radiation alone could not (P = 0.001).
Figure 6 Changes in integrin αvβ3 expression level. (A) A375 cells were treated with either radiation, gold nanorods (50 μg/mL) for one hour, or RGD-GNRs (50 μg/mL) for one hour without or with irradiation, and then the cells were stained with anti-integrin αvβ3 antibody LM609 or isotype-matched control antibody DD7 24 hours after treatment and αvβ3 expression was measured by flow cytometry. (B) αvβ3 expression was quantified with specific fluorescence intensity; experiments were done in triplicates. Columns, mean from three repeated experiments; bars, standard deviation.
Notes: *P < 0.05 compared with control group; **P < 0.05 compared with radiotherapy alone group.
Abbreviations: GNR, gold nanorod; RGD-GNRs, arginine-glycine-aspartate-conjugated gold nanorods; RT, radiotherapy.
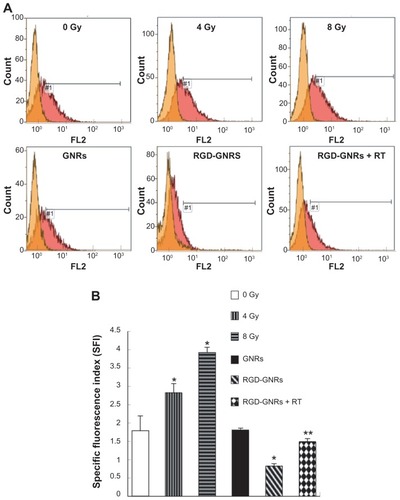
Discussion
The incidence of melanoma is on the rise worldwide, with an estimated age-standardized incidence rate of 40.2 cases per 100,000 population in 2008.Citation23 Melanoma cells are well known to be resistant to radiation, so any new strategies that overcome the relative radioresistance of melanoma tumors could prove to be useful and beneficial to many patients. In the present study, we used gold nanorods as a delivery system and conjugated these to cyclic RGD peptides, an antagonist of integrin αvβ3, to target tumor cells. Since CTAB is a necessary structure-directing agent in the gold nanorod synthesis method, severe cytotoxicity and low stability are still two problems which CTAB-coated gold nanorods need to solve. One of the solutions to these problems is depositing an additional coating on the surface of CTAB-coated gold nanorods. Because silica has been reported to be stable and have low toxicity, it is very suitable for use as a coating material for gold nanorods.Citation24,Citation25 Another feature of a silica system is that it is biocompatible and easily modified, so a gold nanorod coating with silica can easily be covalently conjugated with RGD. In this case, RGD-GNRs will recognize αvβ3 expression-positive tumor cells, be internalized into cells, trapped in endosomes, and degraded within endosome compartments. Therefore, the toxic molecules released by RGD-GNRs will be harmful to the cancer cells that bind to RGD-GNRs. Most normal cells, except for endothelial cells, due to the lack of the RGD receptor on the surface of the cell membrane, could take up few RGD-GNRs,Citation26 so these molecules may be nontoxic to normal cells.
Our results show that RGD-GNRs were readily incorporated into the cells through integrin αvβ3-receptor-mediated endocytosis. RGD-GNRs as a sensitizer could enhance the response of radioresistant melanoma cells with a 1.35 dose modification factor. The mechanisms whereby RGD-GNRs enhanced the response to radiation were associated with multiple factors.
In the present study, RGD-GNRs or radiation alone resulted in an increase in the percentage of cells in the G2/M phase of the cell cycle, while combining these two treatments had an additional effect of inducing the cells in the G2/M phase. Because tumor cells are more radiosensitive in the G2/M phase and less so in the G0/G1 or S phase,Citation27 increased radiation-induced cytotoxicity was seen following treatment of the cells with a combination of RGD-GNRs and radiation. Likewise, increased cell numbers in the G2/M phase could also explain some of the increase in susceptibility to radiation- induced DNA damage reflected by increased expression of the DNA repair protein, γH2AX, compared with cells treated with radiation alone.Citation28 Our results demonstrate that disruption of the cell cycle phases by RGD-GNRs might determine the relative radiosensitivity of A375 cells.
The radiosensitizing effect by RGD-GNRs might also be explained by inhibition of integrin αvβ3 expression. A375 melanoma cells that overexpress the integrin αvβ3, show elevated levels of the protein following irradiation. RGD-GNRs, which with the cells were preincubated, inhibited the expression of spontaneous and radiation-induced expression of integrin αvβ3 and presumably resulted in the sensitization of the cells to radiation (). There are a number of pathways in melanoma cells that contribute to radiation resistance, one of which may involve integrin-stimulated pathways. It has been reported that the impact on cell survival and cell cycle progression in mammalian cells by irradiation is strictly controlled by integrin-mediated adhesion to extracellular matrix proteins and the binding of growth factors to their cognate receptors.Citation29 Integrin-stimulated pathways are similar to and intimately coupled with those pathways triggered by growth factors, eg, vascular endothelial growth factor receptor 2 (VEGFR2).Citation30 Some studies indicate that a direct interplay between αvβ3 and VEGFR-2 occurs at the biochemical level.Citation30,Citation31 High VEGFR2 induces a highly specific radioprotective effect toward αvβ3 integrin-positive endothelial cells in the tumor;Citation32 this results in promoting intrinsic radioresistance. On the other hand, radiation upregulates αvβ3 expression and activates Akt, the key antiapoptic protein kinase in endothelial cells, thus forming a defense mechanism and survival signal against radiation damage.Citation9 This may account for the acquired radioresistance of tumor cells after radiotherapy. Therefore, inhibition of integrin αvβ3 survival signaling by RGD-GNRs may lead to the reversal of tumor cell resistance to radiotherapy, which is a way to enhance the antitumor effects of radiotherapy.
Gold nanorods not only serve as an RGD delivery system to improve selective binding to tumor cells and minimize damage to surrounding normal tissue, but also have other interesting properties, such as increasing the absorption of radiation energy and their preferential accumulation in cancer cells.Citation28,Citation33 It has long been realized that the effect of a defined dosage is increased when a high-Z material is in the targeted zone through a photoelectric effect,Citation34 of which the enhancement is proportional to the amount of the high-Z material. Nevertheless, most studies have focused on low-energy radiation because materials with a high atomic number, such as gold, preferentially absorb kilovoltage x-rays. Megavoltage x-rays, used in the present studies, are more commonly used in the clinic, particularly for deep-seated tumors. Therefore, the radiosensitizing effect of mV x-rays cannot be attributed to high-Z materials alone. Roa et al reportedCitation35 that one possibility, in agreement with present day data, is an interaction between gold nanorods and the cell cycle (). Another possible mechanism is that gold nanorods, when irradiated in the cell, produce a high level of intracellular reactive oxygen species, which leads to elevated levels of oxidative stress and is manifested as an increased level of apoptosis compared with irradiation alone.Citation36 An additional hypothesis proposed as a result of work in DNA plasmid modelsCitation28 is that sensitization occurs due to short-range electrons produced by the interaction between gold nanorods and mV x-rays. The increased production of low-energy electrons close to the DNA causes more damage than radiation alone. Our data confirm that gold nanorods plus radiation induced more apoptosis and mitotic death in A375 cells than radiation alone, and also showed that this effect could be significantly enhanced by conjugating the gold nanorods to RGD.
In summary, we demonstrated that RGD-GNRs have remarkable potential to enhance radiotherapy of melanoma cancer cells. RGD-GNRs, in combination with 6 MV irradiation, enhance the radiosensitivity of the cells. This radiosensitizing effect may be attributable to alterations in the capacity of the cell to resist apoptosis caused by RGD-GNRs, mainly through downregulation of radiation-induced αvβ3 and arresting the cell cycle at the G2/M phase. Because the success of radiotherapy for patients with melanoma largely depends on tumor radiosensitivity, the combination of RGD-GNRs and radiation needs further investigation.
Acknowledgments
This article was funded by research grants (09JC411900, 09411951100, 08411960600) from the Science and Technology Commission of Shanghai and the National Natural Science Foundation of China (81171377), National Basic Research Program of China (2011CB707504, 2010CB933903). The authors thank Drs T FitzGibbon and E Mi for comments on the manuscript.
Disclosure
The authors report no conflicts of interest in this work.
References
- NamJMChungYHsuHCParkCCBeta1 integrin targeting to enhance radiation therapyInt J Radiat Biol2009851192392819895268
- HynesROIntegrins: bidirectional, allosteric signaling machinesCell2002110667368712297042
- Hodivala-DilkeKAlphavbeta3 integrin and angiogenesis: a moody integrin in a changing environmentCurr Opin Cell Biol200820551451918638550
- SeftorRERole of the β3 integrin subunit in human primary melanoma progression: multifunctional activities associated with α(v)β3 integrin expressionAm J Pathol19981535134713519811323
- MonferranSSkuliNDelmasCAlphavbeta3 and alphavbeta5 integrins control glioma cell response to ionising radiation through ILK and RhoBInt J Cancer2008123235736418464290
- WickWWickASchulzJBPrevention of irradiation-induced glioma cell invasion by temozolomide involves caspase 3 activity and cleavage of focal adhesion kinaseCancer Res20026261915191911912174
- HallahanDEGengLCmelakAJTargeting drug delivery to radiation-induced neoantigens in tumor microvasculatureJ Control Release2001741–318319111489494
- MakriliaNKolliasAManolopoulosLSyrigosKCell adhesion molecules: role and clinical significance in cancerCancer Invest200927101023103719909018
- AbdollahiAGriggsDWZieherHInhibition of alpha(v) beta3 integrin survival signaling enhances antiangiogenic and antitumor effects of radiotherapyClin Cancer Res200511176270627916144931
- AlbertJMCaoCGengLLeavittLHallahanDELuBIntegrin alpha v beta 3 antagonist cilengitide enhances efficacy of radiotherapy in endothelial cell and non-small-cell lung cancer modelsInt J Radiat Oncol Biol Phys20066551536154316863930
- PeerDKarpJMHongSNanocarriers as an emerging platform for cancer therapyNat Nanotechnol200721275176018654426
- WangTZhangXPanYFabrication of doxorubicin functionalized gold nanorod probes for combined cancer imaging and drug deliveryDalton Trans201140389789979421879078
- ChenSHJiYXLianQGold nanorods coated with multilayer polyelectrolyte as intracellular delivery vector of antisense oligonucleotidesNano Biomed Eng2010211523
- ZhangXQPanBFWangKElectrochemical property and cell toxicity of gold electrode modified by monolayer PAMAM encapsulated gold nanorodsNano Biomed Eng201023182188
- PanBAoLGaoFEnd-to-end self-assembly and colorimetric characterization of gold nanorods and nanospheres via oligonucleotide hybridizationNanotechnology200516917761780
- MurphyCJanaNControlling the aspect ratio of inorganic nanorods and nanowiresAdv Mater20021418082
- LiXKaoFJChuangCCHeSEnhancing fluorescence of quantum dots by silica-coated gold nanorods under one- and two-photon excitationOpt Express20101811113351134620588995
- JohnssonBLöfåsSLindquistGImmobilization of proteins to a carboxymethyldextran-modified gold surface for biospecific interaction analysis in surface plasmon resonance sensorsAnal Biochem199119822682771724720
- WiendlHMitsdoerfferMHofmeisterVA functional role of hla-g expression in human gliomas: an alternative strategy of immune escapeJ Immunol200216894772478011971028
- YiDKA study of optothermal and cytotoxic properties of silica coated Au nanorodsMater Lett2011156523192321
- LiZMHuangPZhangXJRGD-conjugated dendrimer-modified gold nanorods for in vivo tumor targeting and photothermal therapyMol Pharm20091794104
- GormleyAJMaluginARayABiological evaluation of RGDfK-gold nanorod conjugates for prostate cancer treatmentJ Drug Target2011191091592422082105
- FerlayJAge-standardised incidence rates from the GLOBOCAN 2008 database (http://www-dep.iarc.fr/) using World 2000 population as standard provided on request2010
- TalluryPPaytonKSantraSSilica-based multimodal/multifunctional nanoparticles for bioimaging and biosensing applicationsNanomedicine20083457959218694319
- ZhanQQianJLiXiHeSA study of mesoporous silica-encapsulated gold nanorods as enhanced light scattering probes for cancer cell imagingNanotechnology201052105570420023304
- YunGEBinKSurface plasmon resonance scattering and absorption of biofunctionalized gold nanoparticles for targeted cancer imaging and laser therapySci China Tech Sci201195423582362
- SinclairWKCyclic x-ray responses in mammalian cells in vitroRadiat Res19683336206434867897
- ZhengYHuntingDJAyottePSancheLRadiosensitization of DNA by gold nanoparticles irradiated with high-energy electronsRadiat Res20081691192718159957
- CordesNMeinekeVIntegrin signalling and the cellular response to ionizing radiationJ Mol Histol200435332733715339052
- MahabeleshwarGHFengWReddyKMechanisms of integrin-vascular endothelial growth factor receptor cross-activation in angiogenesisCirc Res2007101657058017641225
- MahabeleshwarGHFengWPhillipsDRByzovaTVIntegrin signaling is critical for pathological angiogenesisJ Exp Med2006203112495250717030947
- GorskiDHBeckettMAJaskowiakNTBlockage of the vascular endothelial growth factor stress response increases the antitumor effects of ionizing radiationCancer Res199959143374337810416597
- ChenWZhangJUsing nanoparticles to enable simultaneous radiation and photodynamic therapies for cancer treatmentJ Nanosci Nanotechnol2006641159116616736782
- SpiersFWThe influence of energy absorption and electron range on dosage in irradiated zoneBr J Radiol19492226152153318147720
- RoaWZhangXGuoLGold nanoparticle sensitize radiotherapy of prostate cancer cells by regulation of the cell cycleNanotechnology2009203737510119706948
- GengFSongKXingJZThio-glucose bound gold nanoparticles enhance radio-cytotoxic targeting of ovarian cancerNanotechnology2011222828510121654036