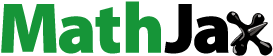
Abstract
Infection and epithelial downgrowth are major problems associated with maxillofacial percutaneous implants. These complications are mainly due to the improper closure of the implant–skin interface. Therefore, designing a percutaneous implant that better promotes the formation of a stable soft tissue biologic seal around percutaneous sites is highly desirable. Additionally, the fibroblast has been proven to play an important role in the formation of biologic seals. In this study, titania nanotubes were filled with 11.2 kDa C-terminal CCN2 (connective tissue growth factor) fragment, which could exert full CCN2 activity to increase the biological functionality of fibroblasts. This drug delivery system was fabricated on a titanium implant surface. CCN2 was loaded into anodized titania nanotubes using a simplified lyophilization method and the loading efficiency was approximately 80%. Then, the release kinetics of CCN2 from these nanotubes was investigated. Furthermore, the influence of CCN2-loaded titania nanotubes on fibroblast functionality was examined. The results revealed increased fibroblast adhesion at 0.25, 0.5, 1, 2, 4, and 24 hours, increased fibroblast viability over the course of 5 days, as well as enhanced actin cytoskeleton organization on CCN2-loaded titania nanotubes surfaces compared to uncoated, unmodified counterparts. Therefore, the results from this in vitro study demonstrate that CCN2-loaded titania nanotubes have the ability to increase fibroblast functionality and should be further studied as a method of promoting the formation of a stable soft tissue biologic seal around percutaneous sites.
Introduction
The long-term success of percutaneous implants relies on the formation of a stable soft tissue biologic seal around the percutaneous site.Citation1–Citation3 Infection and epithelial growth are two of the principal causes of the failure of percutaneous implants.Citation1,Citation3–Citation9 A biosynthetic soft tissue seal can act as a barrier against epithelial downgrowth and bacterial invasion on subepithelial connective tissues and the implant interface.Citation1,Citation10,Citation11
The fibroblast plays an important role in the formation of a biologic seal. The biologic seal of skin is provided by both the epidermis and the dermis, which mainly consist of fibroblasts that produce extracellular matrix (ECM) and various essential components of connective tissues, including glycosaminoglycans and collagen in fibrous tissue.Citation12,Citation13 Previous studies have demonstrated that the quality of the connective tissue at the percutaneous area is more important than the quality of the epithelial attachment for the long-term success of maxillofacial implants.Citation11,Citation14 Therefore, expediting the attachment and growth of fibroblasts could promote the formation of a permanent biologic seal at the percutaneous area and prevent contamination of the implant.Citation15
Currently, the percutaneous surfaces of implants are often made smooth to prevent the adhesion of bacteria.Citation11 Upon healing, the wound closure is likely to be generated by the contraction of fibrous connective tissues during the healing process. However, such smooth surfaces have been shown to lead to detrimental capsule.Citation9,Citation11 Current methods to improve the formation of a biologic seal are aimed at preventing bacterial infection rather than promoting skin growth around the percutaneous site. These methods include altering surgical techniques, modifying the implant design, and coating its surface.Citation16 Coating approaches have been shown to increase the antibacterial ability of implants by using fibroblast growth factor 2-embedded apatite compositesCitation17 and silver nanoparticles.Citation18,Citation19 Although these coatings reduced the presence of bacteria compared to their uncoated counterparts, the infection rate was still high and there was no reported effect on biologic seal formation. Therefore, there is a need for alternate ways to improve the formation of a biologic seal and decrease infection rates. An alternative strategy is to create a growth factor coating that can promote fibroblast growth around the percutaneous site, which would not only provide a stable biological seal but would also provide a permanent barrier against bacterial infection. By optimizing the surface structure, as well as the growth factor loading capacity and release rate, biosynthetic coating materials can achieve both of these goals.
A more suitable drug carrier for creating a growth factor-eluting implant coating is titania nanotubes, which have already been shown to exhibit very good biomechanical compatibility.Citation16,Citation20–Citation22 This material has served as a carrier for several agents such as proteins, antibacterial compounds, and other drugs.Citation18,Citation22–Citation25 In addition, titania nanotubes are highly ordered with different diameters and lengths that can be easily fabricated to specification.Citation26 CCN2 (connective tissue growth factor) is an excellent candidate for loading drugs. CCN2 has been shown to be sufficient to promote fibroblast adhesion, and it also promotes fibroblast proliferation.Citation27–Citation33 Recombinant human CNN2, a lower molecular weight isoform containing the 11.2 kDa C-terminal portion of the full length CNN2 protein, exerts full heparin binding, cell adhesion, and mitogenic CNN2 activity. CNN2 is noncytotoxic at suitable doses and has satisfactory stability. Because the required dose of CNN2 for biological activity is extremely low, it is possible to fabricate coatings with extended delivery times by controlling CNN2 release.
In this study, the purpose was to investigate whether CNN2 could be incorporated into the nanotubes controllably and to evaluate the effect of CNN2-loaded titania nanotubes on the biological responses of fibroblasts.
Materials and methods
Substrate preparation and anodization
Circular pure titanium sheets 1 mm thick and 10 mm in diameter were used as the substrates. The substrates were ultrasonically cleaned in acetone, followed by ethanol, and deionized water for 10 minutes, respectively. They were anodized for 120 minutes in an electrolyte containing 0.3 weight percent hydrofluoric acid and 1 M/L phosphoric acid using a direct current power supply with a platinum electrode as the cathode. The anodization process took place at a constant voltage of 20 V and 25 V. The titanium substrates were rinsed with large amounts of deionized water immediately after anodization, air dried, and sterilized under ultraviolet light for 3 hours per substrate side prior to cell culture experiments.
Surface characterization
Topography and composition of the surface
Structural characterization of the titania nanotube surfaces was performed using a field emission scanning electron microscope (S-4800; Hitachi High Technologies, Tokyo, Japan). Images with a range of scan sizes were acquired from the top and cross-sections.
Roughness
For quantitative surface roughness analysis, atomic force microscopy (Veeco Germany, Mannheim, Germany) was performed on both nanotubular and unmodified surfaces. Measurements were conducted in ambient air with a scan rate of 2 Hz. The scan area was 0.5 × 0.5 μm2. Image analysis software was used to generate micrographs and to quantitatively compare the root mean square roughness and relative surface area of the nanotubular (20 V voltage) and unmodified titanium substrates.
Contact angle and surface free energy
Contact angle measurements were carried out by the sessile drop method on an EasyDrop Standard instrument (KRUSS GmbH, Hamburg, Germany) at room temperature. Three different liquids, ultrapure water, and diiodomethane were in the contact angle measurements. The surface free energy and its components were calculated using the van Oss acid–base method. The contact angle was measured by analyzing the drop shape surface and the calculations of free energy using DSA1 software (KRUSS).
Fabrication of the titania nanotubular CNN2-loaded coating and release kinetics
Filling of the nanotubes
A simplified lyophilization method was chosen to load recombinant human CNN2 (PeproTech, Rocky Hill, NJ) into the nanotubes. This recombinant human CCN2, an 11.2 kDa protein of 98 amino acid residues, is Escherichia coli-derived. A total of 5 μL of protein solution was pipetted onto the surface and spread to ensure even coverage. The surfaces were then allowed to dry under vacuum at room temperature in a freeze dryer for 2 hours. After drying, the loading step was repeated until the appropriate amount of protein (25 ng or 50 ng) was loaded. After the final drying step, excess drug was rinsed from the surface by pipetting 500 mL of phosphate buffered saline (PBS) to analyze the loading efficiency.
Release of CCN2
To measure the release of the protein from the nanotubes, the surfaces were immersed in 500 μL of PBS in a 48-well plate at room temperature with orbital shaking at 70 rpm. A total of 200 μL of samples was taken (replaced with 200 mL of fresh PBS) after specific intervals of time (15 minutes) for up to 120 minutes to determine the release kinetics. The concentration of protein was monitored using a CCN2 enzyme-linked immunosorbent assay (PeproTech). The loading efficiency and the release kinetics for different doses of drug coatings were determined.
Cell culture
Primary human skin fibroblasts were isolated from newborn foreskin as previously described.Citation34 Cells were used at passages three to eight. Human skin fibroblasts were cultured separately in HyClone Dulbecco’s Modified Eagle Medium (Thermo Fisher Scientific Inc, Pittsburg, PA) with HyClone 10% fetal bovine serum (Thermo Fisher) and 1% penicillin/streptomycin (Sigma-Aldrich Corporation, St Louis, MO) under standard culture conditions at 37°C in humidified 5% carbon dioxide/95% air. When the density of fibroblasts reached a suitable population, they were seeded onto the experimental substrates (50 ng CCN2-loaded 20 V voltage nanotubular coated surface, 20 V voltage nanotubular surface, and unmodified surface were chosen as the experimental substrates), which were placed in a 48-well polystyrene plate and stored in a carbon dioxide incubator. The cell media was replaced every 2 days.
Cell viability assay
The concentration of cells seeded onto the specimen substrate was 1000 cells cm−2 per substrate for cell viability experiments in a standard 48-well culture plate under standard cell conditions. Cell viability was assayed with a Cell Counting Kit-8 (Dojindo Molecular Technologies Inc, Kumamoto, Japan). Briefly, after 1, 3, and 5 days of culture, culture medium was moved, and 200 mL of fresh culture medium mixed with 20 μL of Cell Counting Kit-8 reagent was added into each well. Then, the samples were incubated for 2 hours according to the manufacturer’s instructions. In addition, the same volume of culture medium and Cell Counting Kit-8 reagent without cells was also incubated as the background control. An aliquot (150 mL) of incubated medium was pipetted into a 96-well plate and the absorbance at 450 nm was measured for each well as above. All experiments were performed with a sample size of n = 6.
Cell adhesion assay
Cell adhesion was assayed with a live cell labeling kit (Cellstain-CFSE; Dojindo). The fibroblasts (107 cells/mL) were labeled with carboxyfluorescein diacetate succinimyl ester (20 μM/mL) at 37°C for 15 minutes. We pelleted cells by centrifugation and washed the cells by resuspending the pellet in fresh media. This process was repeated for a total of three washes. Then the fibroblasts were seeded at a density of 25,000 cells per substrate for adhesion experiments in a standard 48-well culture plate under standard cell conditions. At the indicated time points (0, 0.25, 0.5, 1, 2, 4, and 24 hours), substrates were rinsed in PBS to remove any nonadherent cells and 200 mL of sodium dodecyl sulfate/Tris (hydroxymethyl) aminomethane hydrochloride solution was added into each well to dissolve the fluorescent dye. An aliquot (150 mL) from each well was transferred to a new 96-well plate. Fluorescence was measured using a fluorometer plate reader (Synergy HT; BioTek, Winooski, VT). All experiments were performed with a sample size of n = 6.
Immunofluorescence of cytoskeletal actin
Epifluorescence microscopy was used to compare the morphological alterations both in regard to the organization of the actin cytoskeleton and cellular shape. The distribution of actin was observed at 4 hours, 1 day, and 3 days of culture. After this period of time, the cells were washed with PBS and fixed in a 4% paraformaldehyde solution for 15 minutes. The samples were then washed in PBS and treated with 0.1% Triton X-100 (5 minutes at room temperature). The samples were then incubated at 37°C for 5 minutes in 1% bovine serum albumin/PBS, followed by washing with PBS and incubation with phalloidin-fluorescein isothiocyanate (Sigma-Aldrich) for 1 hour at 37°C. Actin staining was visualized and analyzed under a laser scanning confocal microscope (FV1000; Olympus Corporation, Tokyo, Japan).
Statistical analyses
All experiments were run in triplicate (at least) and repeated a minimum of three separate times. Experimental data were analyzed statistically using analysis of variance followed by Student’s t-tests. Statistical significance was considered at P < 0.05.
Results
Surface characterization
Scanning electron micrographs () showed that the nanotubes were highly ordered and vertically aligned with a diameter of approximately 100 nm (20 V voltage) and 120 nm (25 V voltage). It was estimated that the nanotubes were between 400 nm and 500 nm deep from the cross-sectional image (). Representative atomic force microscope images of unmodified and 20 V voltage nanotubular anodized titanium were characterized by root mean square roughness and relative surface area (; ). The results showed that the unmodified titanium surface was relatively smooth compared with the 100 nm diameter nanotubular anodized titanium surface.
Table 1 Surface roughness of unmodified and nanotubular titanium (20 V voltage) surfaces
Figure 1 Scanning electron microscope images of (A) 20 V voltage nanotubular anodized titanium under low magnification and high magnification, and nanotubular anodized titanium cross-sections; (B) 25 V voltage nanotubular anodized titanium under low magnification and high magnification, and nanotubular anodized titanium cross-sections; and (C) unmodified titanium.
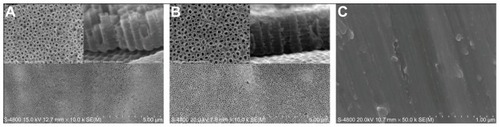
Figure 2 Atomic force microscope images of (A) nanotubular anodized titanium (20 V voltage) and (B) unmodified titanium.

Surface energy calculations from contact angle measurements indicated that the greater surface roughness at the nanoscale level correlated with an increased surface energy. Specifically, the 100 nm diameter nanotubular titanium had a surface energy significantly higher than that of unmodified titanium ().
Table 2 Contact angle (in degrees) and surface energy components of unmodified and nanotubular titanium (20 V voltage) surfaces (in mJ/m2)
Analysis of the CCN2 loading efficiency and release curve
The loading efficiency was expressed as the percentage of loaded drug after washing according to the following formula:
where η is loading efficiency, D0 is drug dose used to fill the nanotubes, and Dr is the drug dose in the rinse solution.
Loading efficiencies for 100 nm diameter nanotubes loaded with 25 ng and 50 ng of CCN2 and 120 nm diameter nanotubes loaded with 50 ng of CCN2 are shown in . These results indicated that 77%, 81%, and 76% of the drug, respectively, was retained in the nanotubes after an initial wash.
Figure 3 (A) Loading efficiency of CNN2 (connective tissue growth factor) in nanotubes; fraction of total CNN2 released from 100 nm diameter nanotubes filled with (B) 25 ng and (C) 50 ng of CNN2; (D) fraction of total CNN2 released from 120 nm diameter nanotubes filled with 50 ng of CNN2.
Notes: The marked time point on each graph indicates when maximum protein has been released, after which the amount of additional drug eluted was negligible. Concentrations at these time points are significantly different from those at time points before (P < 0.05), but were not significantly different from the time points after; n = 3.
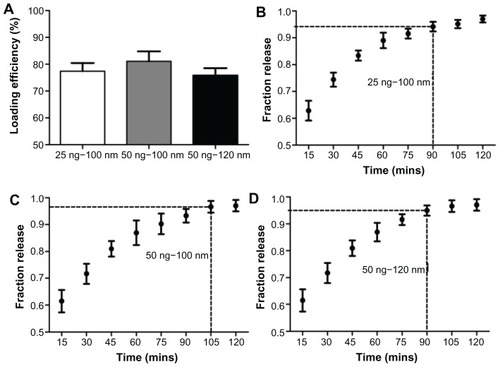
show the CCN2 release kinetics from the coating in PBS with orbital shaking. The amount of released CCN2 diminished gradually with immersion time. As expected, there was a slower and more sustained release from the 100 nm diameter nanotubes loaded with 50 ng CCN2 compared to 100 nm diameter nanotubes loaded with 25 ng CCN2 and 120 nm diameter nanotubes loaded with 50 ng. Kinetic analysis of the nanotubes loaded with 50 ng of CCN2 indicated that the maximum drug had eluted within 105 minutes. Alternately, kinetic analysis of the 100 nm diameter nanotubes loaded with 25 ng of CCN2 and the 120 nm diameter nanotubes loaded with 50 ng indicated that the maximum drug in both had eluted within 90 minutes.
Cell viability
The cell viability of fibroblasts grown on the above substrates and the corresponding incubation times are summarized in . As shown, cell growth on the CCN2-loaded titania nanotubular surface was significantly higher than the other two kinds of surfaces at days 1, 3, and 5 after incubation (P < 0.05). However, the difference between the nanotubular surface and the unmodified surface was not obvious from statistical analysis. This finding indicates that titania nanotubes with a CCN2 coating release growth factor in a sustainable manner that promotes cell mitosis continuously.
Figure 4 Cell viability on CCN2 (connective tissue growth factor)-loaded nanotubular titanium, nanotubular titanium, and unmodified titanium surfaces.
Notes: n = 6; *P < 0.05 compared to nanotubular titanium; **P < 0.05 compared to unmodified titanium.
Abbreviations: CNN2, connective tissue growth factor; Ti, titanium.

Fibroblast adhesion
Results of the present study demonstrate increased fibroblast adhesion to the CCN2-loaded titania nanotubular surface compared to nanotubular titanium and unmodified titanium surfaces (). There was not a statistically significant (P < 0.05) difference in fibroblast adhesion between the nanotube anodized titanium compared to unmodified titanium. As expected, the results also showed increased (P < 0.05) adhesion of fibroblasts to the CCN2-loaded titania nanotubular surface.
Morphological alterations and cytoskeletal actin
These results showed a strong initial interaction between the cells and the modified surface (). Notably, the morphology of cells grown on these surfaces was different after different times during the culturing. At 4 hours, many stress fibers were apparent around the periphery of the cells on the CCN2-loaded titania nanotubular surface. However, the cells grown on the other two kinds of surfaces at this point, whilst showing lamella formation, had fewer stress fibers. Within 1 day, cells on the CCN2-loaded titania nanotubular surface displayed thick, contractile stress fibers. In contrast, the cells on the other two surfaces had noticeably fewer contractile stress fibers. Within 3 days, cells on the CCN2-loaded titania nanotubular surface exhibited thick stress fibers and a more voluminous cell body and began to become confluent, whereas fewer cells on the other two surfaces were confluent.
Figure 6 Fluorescent images of actin cytoskeleton among fibroblasts reacting to (A, D, and G) CCN2 (connective tissue growth factor)-loaded nanotubular titanium, (B, E, and H) nanotubular titanium, and (C, F, and I) unmodified titanium surfaces after (A–C) 4 hours, (D–F) 1 day, and (G–I) 3 days.
Note: Bar = 10 μm.
Abbreviations: CNN2, connective tissue growth factor; d, days; h, hours; Ti, titanium.
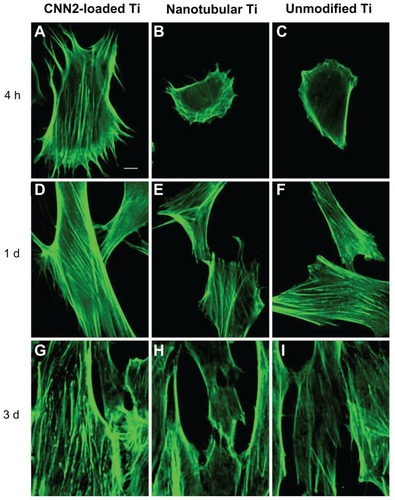
Discussion
CCN2-loaded titania nanotubular surfaces promoted excellent cellular responses throughout the 5-day study period. At 0.25, 0.5, 1, 2, and 4 hours, the number of cells initially adhered to the CCN2 coating were higher than the other two surfaces. Cells adhered to the implant surfaces by attaching to the ECM, and cytoskeletal organization is an important criterion of cell–ECM interactions. Cytoskeletal organization is also extremely important in modulating cell morphology, motility, and adhesion. In the present study, when the cell initially responded to the CCN2-coated nanotubular surface, the cytoskeleton was formed faster for these cells than for those reacting to control surfaces. Additionally, with time, denser and thicker parallel-oriented stress fibers were noticed on the surface. Therefore, there were most likely more stable cell substrate interactions on the CCN2-coated surface.
Infection and epithelial downgrowth are major problems with percutaneous implants and both are mainly due to the improper closure of the implant–soft tissue interface.Citation16 Most current methods are aimed at preventing bacterial infection rather than repairing the lack of connective tissue growth around the percutaneous site.Citation18,Citation24 A better strategy is to create an implant surface with a growth factor coating that can promote connective tissue growth around the surgical site, which would not only prevent epithelial downgrowth but also provide a permanent barrier for bacteria invasion. Delivery of drugs locally from an implant surface coating rather than systemically can reduce unnecessary side effects and the amount of drug required to reach the same or better therapeutic efficacy.Citation35 This implant surface with a growth factor coating not only offers the advantage of a high localized drug concentration but is also an effective way of delivering growth factors directly to the percutaneous site. Several previous studies have tried to fabricate implant surfaces with growth factor coatings by adsorbing antibiotics or growth factors directly to the implant surface with bone cementsCitation36,Citation37 or by loading agents in collagen sponges, porous coatings, or polymer-based matrices.Citation38,Citation39 However, all of these approaches have shortcomings, which include chemical instability and local inflammatory reactions due to material composition.
The titanium nanotube was chosen as the drug carrier for making the growth factor coating because it has been shown to have good biocompatibility. In addition, previous research has clearly proven that nanotube surfaces promote bone, skin, and other tissue growth more than current conventional surfaces.Citation16,Citation20–Citation22 Several papers have been published that have focused on the applications of titanium nanotube drug coatings, but none of them has examined the use of this delivery system at the percutaneous site.Citation18,Citation23,Citation24,Citation35 Titanium nanotube drug-eluting coatings on the implant surface can have a variety of applications. However, the present study just focused on their use for percutaneous implants. In the present study, these nanotube surfaces showed increased hydrophilicity compared to unmodified surfaces. Therefore, these surfaces can be easily modified by organic molecules.
Recombinant human CCN2 is an 11.2 kDa C-terminal protein that has a positive effect on fibroblasts and is a model drug. It has been shown to be sufficient to promote fibroblast adhesion via integrins, and it also promotes fibroblast proliferation.Citation27–Citation30 CCN2 has also been shown to play a key role in mediating the formation of attachments between fibroblasts and the ECM at focal adhesions.Citation40 CCN2 gene knockout mice also display decreased expression of ECM components and matrix metalloproteinases.Citation12 It is envisioned that other drugs with fewer detrimental side effects but of a similar size could also be loaded into nanotubular titanium to improve the interface between the implant and the soft tissue. The results of the present study indicate that CCN2 released from titania nanotubes improved the functionality of fibroblasts on the titanium surface.
In the present study, the nanotubes were 100 nm in diameter. Although a diameter wider than 100 nm may significantly allow more solvent and more drug to enter the nanotubes, more drug would be lost during the rinse step as a result of the larger diameter and the lower packing density. Results showed that the loading efficiency and release time of 120 nm diameter nanotube were both lower than those of the 100 nm diameter nanotube. Another paper also demonstrated that larger diameter nanotubes (300 nm) can elute less drug than smaller nanotubes (100 nm) of the same length.Citation23 Using a simplified lyophilization step to fill the nanotubes with CCN2, the loading efficiencies of the drug coating groups were both higher than 75%. This means that there was little waste in the drug filling process. Other studies have also shown that the nanotube surface is hydrophilic enough for solvents to reach the maximum depth attainable by using only a capillary force-dependent filling method. Thus, any further improvements in drug loading would require the application of another filling method.Citation23
To release protein from the nanotubes, the surfaces were immersed in PBS with orbital shaking. The release kinetics showed that the time of release for CCN2 was about 105 minutes. Some reports have shown elution times over weeks for loading with paclitaxel, sirolimus, bovine serum albumin, or silver nanoparticles that were seemingly based on different sampling without shaking.Citation18,Citation23 Other reports have shown similar release times.Citation22,Citation24 The 105 minute-released CNN2 mainly enhanced initial adhesion and cytoskeletal development of the fibroblast. Rapid and strong adhesion and rapid growth of the fibroblast on the implant surface would promote the formation of a permanent liquid bacterial seal in the percutaneous area and would prevent early contamination of the implant site,Citation15 which is very important for the successful formation of a permanent biologic seal. Initial adhesion and cytoskeletal development of the fibroblast means rapid growth in the subsequent period, which were proven in the results.
In the present study, although the nanotubular titanium had greater nanoscale surface roughness and higher surface energy than that of unmodified titanium, there were no statistically significant differences between the unmodified surface and the nanotubular surface in regard to fibroblast response. Recent reports have shown better cell attachment to nanotubular surfaces that are more hydrophobic than unmodified surfaces.Citation20,Citation41,Citation42 But according to some results in the literature, fibroblasts prefer a smooth surface to a rough surface.Citation43
To prevent any side effect of CNN2 in the bone healing process, a two-stage solution may be a good choice for the further clinic application of the CNN2-loaded titania nanotube surface on percutaneous implant. This would involve initially attaching a conventional component to the bone and subsequently, when the wound has already healed, inserting the percutaneous component with CNN2-loaded titanium nanotube surface.
Conclusion
This study showed the effect of a CCN2-loaded nanotubular titanium coating on fibroblast functionality. By using a simplified lyophilization method, CCN2 was loaded into nanotubes. These titania nanotubes coated with CCN2 underwent the elution process at a regular release rate with a release time of approximately 105 minutes. Moreover, the present study provides evidence of enhanced fibroblast adhesion and viability due to this CCN2-loaded titania nanotubular coated substrate under in vitro conditions, which also promoted actin cytoskeleton organization. The results suggest that a CCN2-loaded nanotubular titanium coating may be useful for percutaneous implants due to the beneficial effects on the formation of a soft tissue biological seal around the percutaneous site of the implant surface.
Acknowledgments
Financial support was provided by the National Natural Science Foundation of China (No. 50805141).
Disclosure
The authors report no conflicts of interest in this work.
References
- von RecumAFApplications and failure modes of percutaneous devices: a reviewJ Biomed Mater Res19841843233366234317
- GittoCAPlataWGSchaafNGEvaluation of the peri-implant epithelial tissue of percutaneous implant abutments supporting maxillofacial prosthesesInt J Oral Maxillofac Implants1994921972068206556
- JansenJAPaquayYGvan der WaerdenJPTissue reaction to soft-tissue anchored percutaneous implants in rabbitsJ Biomed Mater Res1994289104710547814432
- KimJWKikkawaDOAboyAGlasgowBJChronic exposure of hydroxyapatite orbital implants: cilia implantation and epithelial downgrowthOphthal Plast Reconstr Surg2000163216222
- KnabeCGrosse-SiestrupCGrossUHistologic evaluation of a natural permanent percutaneous structure and clinical percutaneous devicesBiomaterials199920650351010213352
- DasseKAInfection of percutaneous devices: prevention, monitoring, and treatmentJ Biomed Mater Res19841844034116736075
- MooneyVSchwartzSARothAMGorniowskyMJPercutaneous implant devicesAnn Biomed Eng1977513446851262
- GangjeeTColaizzoRvon RecumAFSpecies-related differences in percutaneous wound healingAnn Biomed Eng19851354514674073629
- ChehroudiBBrunetteDMSubcutaneous microfabricated surfaces inhibit epithelial recession and promote long-term survival of percutaneous implantsBiomaterials200223122923711762842
- HamdanMBlancoLKhraisatATresguerresIFInfluence of titanium surface charge on fibroblast adhesionClin Implant Dent Relat Res200681323816681491
- YoshinariMMatsuzakaKInoueTOdaYShimonoMEffects of multigrooved surfaces on fibroblast behaviorJ Biomed Mater Res A200365335936812746883
- ChenYAbrahamDJShi-WenXCCN2 (connective tissue growth factor) promotes fibroblast adhesion to fibronectinMol Biol Cell200415125635564615371538
- KieltyCMShuttleworthCAMicrofibrillar elements of the dermal matrixMicrosc Res Tech19973844134279297691
- JansenJAvan der WaerdenJPde GrootKFibroblast and epithelial cell interactions with surface-treated implant materialsBiomaterials199112125312009341
- JansenJAvan der WaerdenJPde GrootKEffect of surface treatments on attachment and growth of epithelial cellsBiomaterials19891096046082611309
- PuckettSDLeePPCiomborDMAaronRKWebsterTJNanotextured titanium surfaces for enhancing skin growth on transcutaneous osseointegrated devicesActa Biomater2010662352236220005310
- MutsuzakiHItoASakaneMSogoYOyaneAOchiaiNFibroblast growth factor-2-apatite composite layers on titanium screw to reduce pin tract infection rateJ Biomed Mater Res B Appl Biomater200886236537418286592
- ZhaoLWangHHuoKAntibacterial nano-structured titania coating incorporated with silver nanoparticlesBiomaterials201132245706571621565401
- CaoHLiuXMengFChuPKBiological actions of silver nanoparticles embedded in titanium controlled by micro-galvanic effectsBiomaterials201132369370520970183
- BurnsKYaoCWebsterTJIncreased chondrocyte adhesion on nanotubular anodized titaniumJ Biomed Mater Res A200988356156818306319
- YaoCSlamovichEBWebsterTJEnhanced osteoblast functions on anodized titanium with nanotube-like structuresJ Biomed Mater Res A200885115716617688267
- ParkJBauerSSchlegelKANeukamFWvon der MarkKSchmukiPTiO2 nanotube surfaces: 15 nm – an optimal length scale of surface topography for cell adhesion and differentiationSmall20095666667119235196
- PengLMendelsohnADLaTempaTJYoriyaSGrimesCADesaiTALong-term small molecule and protein elution from TiO2 nanotubesNano Lett2009951932193619323554
- PopatKCEltgrothMLatempaTJGrimesCADesaiTADecreased Staphylococcus epidermis adhesion and increased osteoblast functionality on antibiotic-loaded titania nanotubesBiomaterials200728324880488817697708
- EaninweneG2ndYaoCWebsterTJEnhanced osteoblast adhesion to drug-coated anodized nanotubular titanium surfacesInt J Nanomedicine20083225726418686785
- RoyPBergerSSchmukiPTiO2 nanotubes: synthesis and applicationsAngew Chem Int Ed Engl201150132904293921394857
- BrigstockDRSteffenCLKimGYVeguntaRKDiehlJRHardingPAPurification and characterization of novel heparin-binding growth factors in uterine secretory fluids. Identification as heparin-regulated Mr 10,000 forms of connective tissue growth factorJ Biol Chem19972723220275202829242708
- ChenCCChenNLauLFThe angiogenic factors Cyr61 and connective tissue growth factor induce adhesive signaling in primary human skin fibroblastsJ Biol Chem200127613104431045211120741
- LeaskAAbrahamDJThe role of connective tissue growth factor, a multifunctional matricellular protein, in fibroblast biologyBiochem Cell Biol200381635536314663501
- BallDKRachfalAWKemperSABrigstockDRThe heparin-binding 10 kDa fragment of connective tissue growth factor (CTGF) containing module 4 alone stimulates cell adhesionJ Endocrinol20031762R1712553878
- PerbalBThe CCN family of genes: a brief historyMol Pathol200154210310411322165
- BrigstockDRThe CCN family: a new stimulus packageJ Endocrinol2003178216917512904165
- Van BeekJPKennedyLRockelJSBernierSMLeaskAThe induction of CCN2 by TGFβ1 involves Ets-1Arthritis Res Ther200682R3616469114
- El-GhalbzouriAVan Den BogaerdtAJKempenaarJPonecMHuman adipose tissue-derived cells delay re-epithelialization in comparison with skin fibroblasts in organotypic skin cultureBr J Dermatol2004150344445415030326
- PopatKCEltgrothMLaTempaTJGrimesCADesaiTATitania nanotubes: a novel platform for drug-eluting coatings for medical implants?Small20073111878188117935080
- PriceJSTencerAFArmDMBohachGAControlled release of antibiotics from coated orthopedic implantsJ Biomed Mater Res19963032812868698690
- SilvermanLDLukashovaLHermanOTLaneJMBoskeyALRelease of gentamicin from a tricalcium phosphate bone implantJ Orthop Res2007251232917034052
- FriedlaenderGEPerryCRColeJDOsteogenic protein-1 (bone morphogenetic protein-7) in the treatment of tibial nonunionsJ Bone Joint Surg200183-ASuppl 1(Pt 2)S151S15811314793
- LindMOvergaardSSoballeKNguyenTOngpipattanakulBBüngerCTransforming growth factor-β1 enhances bone healing to unloaded tricalcium phosphate coated implants: an experimental study in dogsJ Orthop Res19961433433508676246
- Shi-WenXStantonLAKennedyLCCN2 is necessary for adhesive responses to transforming growth factor-β1 in embryonic fibroblastsJ Biol Chem200628116107151072616484225
- BrammerKSOhSCobbCJBjurstenLMvan der HeydeHJinSImproved bone-forming functionality on diameter-controlled TiO(2) nanotube surfaceActa Biomater2009583215322319447210
- DemetrescuIPirvuCMitranVEffect of nano-topographical features of Ti/TiO(2) electrode surface on cell response and electrochemical stability in artificial salivaBioelectrochemistry201079112212920189888
- BrunetteDMPrinciples of cell behavior on titanium surfaces and their application to implanted devicesBrunetteDMTengvallPTextorMThomsenPTitanium in Medicine: Material Science, Surface Science, Engineering, Biological Responses, and Medical ApplicationsBerlinSpringer-Verlag2001485512