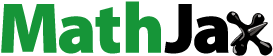
Abstract
Background
Liposomes can be modified with different ligands to control their biological properties, such as longevity, targeting ability, and intracellular penetration, in a desired fashion. The aim of this study was to modify liposomes with a novel mannosylated polyethylene glycol-phosphatidylethanolamine (M-PEG-PE) ligand to achieve active targeted gene delivery.
Methods
Rat Kupffer cells were isolated and used as model cells for in vitro evaluation of cytotoxicity and transfection efficiency. The modified liposomes were intravenously injected into the rats, and Kupffer cells were isolated and analyzed by flow cytometry for in vivo gene delivery and expression.
Results
The M-PEG-PE-modified liposome-enhanced green fluorescence protein plasmid (M-PEG-PE-Lipo-pEGFP) complexes had a particle size of 237 nm and a loading efficiency of 90%. The M-PEG-PE-Lipo-pEGFP complexes displayed remarkably higher transfection efficiency than unmodified Lipo-pEGFP, both in vitro (51%–30%) and in vivo (43%–27%).
Conclusion
M-PEG-PE could function as an excellent active targeting ligand, and M-PEG-PE-modified liposomes could be promising active targeted drug delivery vectors.
Introduction
Gene delivery using nonviral pharmaceutical vectors has long been considered, because these vectors are less toxic, less immunogenic, and easily modified.Citation1–Citation3 Nonviral carriers, such as polymeric nanoparticles,Citation4 liposomes,Citation5 micelles,Citation6 nanocapsules,Citation7 solid lipid nanoparticles,Citation8 niosomes,Citation9 and other vectors have been widely used as gene delivery systems. It has been shown that liposomes can act as biocompatible, biodegradable, and nonimmunogenic drug/gene carriers, and the advantages of liposomal-encapsulated drugs/genes are a prolonged duration of exposure, selective delivery to the intended site of action, and improved therapeutic index.Citation10,Citation11 Among the liposomes, the Lipofectamine ™ 2000 transfection reagent is a proprietary formulation for transfection of nucleic acids (DNA and RNA) into cells, and has been widely used by many researchers.Citation12–Citation14
Liposomes can be modified with different kinds of ligands to control their biological properties such as longevity, targeting ability, and intracellular penetration, in a desired fashion.Citation15 Modification of liposomes using polyethylene glycol (PEG) has emerged as a common strategy to ensure stealth shielding and long circulation of liposomal drug/ gene delivery systems. PEG modification could also provide liposomes with active targeting properties by enabling covalent attachment of a wide assortment of targeting ligands by amide bonding or disulfide bridge formation.Citation16
A series of PEG-containing ligands, commonly known as PEG-phosphatidylethanolamine (PEG-PE) conjugates, was described by Torchilin et al who demonstrated that PEG-PE conjugates with various PEG lengths and terminal-targeted moieties can provide extremely stable, long-circulating, and actively targeted nanocarriers which spontaneously accumulate at specific sites.Citation4,Citation6,Citation17 PEG-PE was subsequently used widely in liposomal formulations,Citation18,Citation19 and more recently, several such ligands (eg, PEG-PE and TAT-PEG-PE) have also been used for targeted delivery of drugs/genes.Citation20–Citation22
Saccharide ligands, such as galactose, mannose, lactose, and maltose, have been extensively investigated for modification of liposomes to achieve site-specific targeting.Citation23,Citation24 In this study, a novel conjugated ligand, ie, mannose-PEG-PE (M-PEG-PE), was synthesized, and M-PEG-PE-modified Lipofectamine™ 2000 (Lipo) liposomes were investigated as an active targeting gene delivery system. Enhanced green fluorescence protein plasmid (pEGFP) was used as the model gene. The novel modified vectors were delivered intravenously into rats and the in vivo targeting behavior of M-PEG-PE-modified liposome-pEGFP (M-PEG-PE-Lipo-pEGFP) complexes was investigated in liver macrophages and compared with that of unmodified Lipo-pEGFP complexes.
Materials and methods
Materials
Butyl carbonyl (Boc)-NH-PEG2000-COOH was purchased from Shanghai Yarebio Co Ltd (Shanghai, China). Mannose, L-α-phosphatidylethanolamine (PE), MTT (3-[4,5-dimethyl-2-thiazolyl]-2,5-diphenyl-2H-tetrazolium bromide), and concanavalin A were purchased from Sigma-Aldrich Co, Ltd, (St, Louis, MO). pEGFP-N1 was provided by Shandong University (Shandong, China). Quant-iT™ PicoGreen® dsDNA quantitation reagent and Lipofectamine 2000 were obtained from Invitrogen Life Technologies (Carlsbad, CA). All other chemicals were of analytical grade or higher.
Animals
Adult male Sprague-Dawley rats (10–11 weeks old) were purchased from the Medical Animal Testing Center of Shandong Province and housed under standard laboratory conditions. All animal experiments complied with the requirements of the National Act on the Use of Experimental Animals (People’s Republic of China).
Synthesis of M-PEG-PE ligands
M-PEG-PE ligands were synthesized as described in . Boc-NH-PEG-COOH 100 mg was dissolved in dimethyl sulfoxide and stirred with 36 mg of PE to form a mixture. 1-[3-(dimethylamino)propyl]-3-ethylcarbodiimide (EDC·HCl) 72 mg) and triethylamine (1 equivalent of EDC·HCl) were dissolved in dimethyl sulfoxide and added dropwise into the mixture in an ice bath, stirred for 36 hours, and concentrated hydrochloric acid was then used to detach the Boc group and produce NH2-PEG-CO-NH-PE. Mannose 50 mg was dissolved with 1 M sodium hydroxide of 1 mL and stirred for 30 minutes for alkalinization, then 1 mL of chloroacetic acid (20%) was added to the solution and stirred in an oil bath at 60°C for 6 hours. Following this, hydrochloric acid 1 M was added until pH was 2–3 to complete the carboxymethylation of mannose.Citation8 Carboxymethylated mannose was then stirred with NH2-PEG-CO-NH-PE in dimethyl sulfoxide solution, and EDC·HCl mixed with triethylamine (one equivalent of EDC·HCl in dimethyl sulfoxide) was added dropwise into the solution in an ice bath and stirred for 24 hours. Dimethyl sulfoxide was moved by rotary evaporation, and the product was dialyzed against Milli-Q (Billerica, MA) water for 24 hours to form M-PEG-PE.
Fabrication of Lipo-pEGFP complexes
Lipo-pEGFP complexes were prepared according to the manufacturer’s instructions for Lipofectamine 2000. Briefly, 100 μL of pEGFP 1 μg/μL was mixed with 200 μL of Lipofectamine 2000 by vortexing for 30 seconds. Incubation of the mixture for 30 minutes at room temperature-facilitated formation of the DNA-loaded liposomes ().
Modification of Lipo-pEGFP with M-PEG-PE
M-PEG-PE-Lipo-pEGFP complexes were produced by the solvent diffusion method ().Citation24,Citation25 Briefly, M-PEG-PE ligands were dissolved in 2 mL of phosphate-buffered saline (pH 7.4). The solution was then added dropwise into 10 mL of Lipo-pEGFP complexes and stirred at 600 rpm and room temperature, with immediate surface modification. Subsequently, free M-PEG-PE was removed from the modified Lipo-pEGFP by gel chromatography using a Sephadex® G-50 column (GE Healthcare, Uppsala, Sweden). The complexes obtained were resuspended in Milli-Q water, washed three times, and filtered through a membrane with a 0.80 μm pore size to obtain M-PEG-PE-Lipo-pEGFP. To optimize the modification ratio, M-PEG-PE ligands dissolved in phosphate-buffered saline were designed at different weight ratios for the Lipo-pEGFP (w/w). The particle size and zeta potential of the complexes were determined using a Zetasizer Nano ZS (Malvern Instruments, Worcestershire, UK). The shape of the M-PEG-PE-Lipo-pEGFP was examined by transmission electron microscopy. Samples were prepared by placing one drop of suspension onto a copper grid and dried, followed by negative staining with one drop of a 3% aqueous solution of sodium phosphotungstate for contrast enhancement. The air-dried samples were then examined directly under the transmission electron microscope.
M-PEG-PE modification and pEGFP-loading capacity of M-PEG-PE-Lipo-pEGFP
To confirm the success of modification and determine the optimum ratio of M-PEG-PE to Lipo-pEGFP, several experiments were carried out as follows.
PicoGreen fluorometry assay
During the modification procedure, M-PEG-PE ligands were coated onto Lipo-pEGFP carriers due to the electric charge and lipophilic interaction. A PicoGreen fluorometry assay was used to determine the appropriate modification rate of M-PEG-PE ligands and also to quantitate the amount of pEGFP carried by the optimally modified liposomes.Citation26 Different ratios of M-PEG-PE to Lipo-pEGFP (from 2% to 30%, w/w) were used to prepare the M-PEG-PE-Lipo-pEGFP complexes, and the pEGFP was isolated from M-PEG-PE-Lipo-pEGFP by centrifugation at 1000 g and 4°C for 30 minutes. The concentration of pEGFP was determined by fluorescence and comparing the sample with the blank liposome supernatant. The amount of pEGFP loaded into the liposomes was calculated according to the linear calibration curve of pEGFP:
Concanavalin A agglutination study
A concanavalin A agglutination assay was performed to confirm that the M-PEG-PE ligands were successfully modified onto the Lipo-pEGFP surface. Binding of the terminal α-mannose residues to concanavalin A causes agglutination of the complexes in solution, resulting in an increase in turbidity. Citation27–Citation29 Therefore, 100 μL of M-PEG-PE-Lipo-pEGFP complexes was added into 500 μL of concanavalin A 1 mg/mL in phosphate-buffered saline (pH 7.4) with 5 mM of calcium chloride (CaCl2) and 5 mM of magnesium chloride (MgCl2) at room temperature. The increase in turbidity at 360 nm (OD360) was monitored at room temperature for 3 minutes.
Isolation and culture of Kupffer cells
Kupffer cells were isolated from Sprague-Dawley rats under pentobarbitone anesthesia using a method described elsewhere. Citation30–Citation32 In brief, the rat portal vein was cannulated and perfused with Hank’s buffered salt solution for 10 minutes, the liver was excised, and the perfusate was discarded. The liver was then perfused with 0.2% pronase, then with a recirculating solution of 0.05% pronase and 0.05% collagenase until the liver was digested. The liver was thereafter cut into small pieces, suspended in a solution containing 0.02% pronase, 0.05% collagenase, and 0.005% DNase, and then agitated. Following digestion, the liver homogenate was filtered through sterile gauze and centrifuged at 1000 g and 4°C for 10 minutes. The supernatant was removed and the pellet then resuspended in Percoll™ gradient. Aliquots of this cell suspension were added to aliquots of Percoll gradient. These were carefully overlaid with Hank’s balanced salt solution and centrifuged. The nonparenchymal cell-enriched layer observed at the interface between the two layers was carefully harvested and diluted with Hank’s balanced salt solution. The suspension was then centrifuged to precipitate the Kupffer cells, which were then seeded into a 96-well microtiter plate at a density of 2 × 104 cells/well in RPMI 1640 supplemented with 10% fetal bovine serum and antibiotics. After incubation at 37°C for 2 hours under 5% CO2 atmosphere, the culture medium was replaced by 200 μL of fresh RPMI 1640 to yield the purified Kupffer cells.
The isolated Kupffer cells were defined on the basis of phagocytosis of latex beads and positive immunostaining with the antibody to the ED2 epitope. Using the abovementioned method, about 9.35 × 107/rat liver Kupffer cells were obtained, the purity of which was about 92.6% (1.01 × 108 nonparenchymal rat liver cells). The isolated and purified rat Kupffer cells retained their in vivo morphological, biological, and immunological characteristics.
In vitro cytotoxicity evaluation and transfection analysis
The in vitro cytotoxicity of M-PEG-PE-Lipo-pEGFP in Kupffer cells was evaluated by MTT assay.Citation30 The Kupffer cells were incubated with M-PEG-PE-Lipo-pEGFP and unmodified Lipo-pEGFP at various concentrations (10, 20, 50, 100, and 200 μg/mL) for 48 hours at 37°C and 5% CO2. The cell viability was then assessed by MTT assay; 5 mg/mL of MTT in phosphate-buffered saline was then added to each well and the plate was incubated for an additional 4 hours at 37°C and a 5% CO2 atmosphere. The medium containing MTT was then removed, and the crystals formed by living cells were dissolved in 100 μL of dimethyl sulfoxide. The absorbance at 570 nm was determined by microplate reader. Untreated cells were taken as a control with 100% viability, and cells without the addition of MTT were used as a blank to calibrate the spectrophotometer to zero absorbance. The relative cell viability (%) compared with control cells was calculated as (Abssample/Abscontrol) × 100.
For analysis of transfection efficiency, the Kupffer cells were seeded into 24-well plates at a density of 1 × 105 cells/ well in 1 mL of RPMI 1640 with 10% fetal bovine serum. After about 24 hours, when the cells were at about 80%–90% confluence, the media were replaced with 400 μL of transfection media containing M-PEG-PE-Lipo-pEGFP. Naked DNA was used as a negative control and unmodified Lipo-pEGFP was used as a positive control. The original incubation medium was replaced with 1 mL of complete medium after incubation at 37°C for 4 hours under a 5% CO2 atmosphere. The cells were incubated and studied at 72 hours following transfection. The fluorescent cells were observed using an inverted fluorescence microscope, at which time photographs were taken. To quantitate transfection efficiency further, the cells were washed with 1 mL of phosphate-buffered saline (100 g, 4°C for 5 minutes) and detached with trypsin-EDTA. The supernatant was discarded and resuspended in 300 μL of phosphate-buffered saline, and the fluorescence intensity of the cells was determined using a flow cytometer equipped with a 488 nm argon laser for excitation. For each sample, about 10,000 events were collected and fluorescence was detected. Signals were amplified in logarithmic mode for fluorescence to determine the positive events by a standard gating technique. The percentage of positive events was calculated as the events within the gate divided by the total number of events, excluding cell debris.
In vivo gene delivery
Adult male Sprague-Dawley rats were divided into three groups (six rats in each group) and injected intravenously with 1 mL of M-PEG-PE-Lipo-pEGFP, Lipo-pEGFP, and naked pEGFP (300 μL per rat, containing 30 μg of pEGFP). At predetermined time intervals, rat Kupffer cells were isolated as described earlier. The Kupffer cells were then seeded into 24-well plates, and incubated until 48 hours after transfection. The cells were then washed with 1 mL of phosphate-buffered saline (100 g and 4°C for 5 minutes) and detached with trypsin-EDTA. The supernatant was discarded and resuspended in 300 μL of phosphate-buffered saline, mixed well, and flow cytometry was performed to determine the amount of Kupffer cells that had been successfully transfected.
Statistical analysis
All studies were repeated three times and measurements were carried out in triplicate. Results are reported as the mean ± standard deviation. Statistical significance was determined using the Student’s t-test. Differences between experimental groups were considered significant when the P value was <0.05.
Results
Structural confirmation of M-PEG-PE ligands
The structure of M-PEG-PE was confirmed by infrared and 1H nuclear magnetic resonance (NMR) spectroscopy. Infrared ν/cm−1: 3521.5 (–NH–, –OH); 1818.3 (−C=O); 1665.4 (–HN–CO–); 1631.9 (–HN–CO–). 1H NMR (dimethyl sulfoxide-d6, 300 mHz) δs 2.45 (CH2CO), 3.28 (CH2 N), and 6.08 (NH). The production rate was around 65%.
Characterization of DNA-loaded liposomes
Transmission electron microscopic images of the vectors are shown in . The mean particle size, polydispersity index, and zeta potential of Lipofectamine 2000, Lipo- pEGFP, and M-PEG-PE-Lipo-pEGFP are characterized and summarized in .
Figure 3 Transmission electron microscopic images of Lipofectamine-green fluorescence protein plasmid (A), mannosylated polyethylene glycol-phosphatidylethanolamine- Lipofectamine-green fluorescence protein plasmid (B and C).

Table 1 Particle size and zeta potential of liposomes (mean ± standard deviation, n = 3)
Optimization of M-PEG-PE modification rate
The quantity of pEGFP loaded into the variously modified liposomes was calculated and is displayed in . Unmodified liposomes (0%) reached a gene loading quantity of 90%. With the increasing modification rate (from 2% to 10%), the pEGFP loading amount showed no obvious change (all around 90%). When the ratio of the ligands was above 10%, the loading capacity decreased, and only 15% gene carrying was observed at the ratio of 30%. The optimum rate was 10%, and this ratio was used for the investigations described below.
Determination of M-PEG-PE modification
A concanavalin A agglutination study was used to identify further that the M-PEG-PE ligands were successfully modified onto the Lipo-pEGFP surface. As shown in , the turbidity of M-PEG-PE-Lipo-pEGFP appeared to increase, while the Lipofectamine 2000 and Lipo-pEGFP formulations appeared not to show any significant increase in turbidity.
In vitro cytotoxicity evaluation
The in vitro cytotoxicity of M-PEG-PE-Lipo-pEGFP and unmodified Lipo-pEGFP at various concentrations was evaluated by MTT assay. The cell viability of the liposomes over the studied concentration range (10–200 μg/mL) was between 80% and 100% compared with the controls (). M-PEG-PE-Lipo-pEGFP showed no greater cytotoxicity than Lipo-pEGFP at any concentration (P > 0.05).
In vitro transfection analysis
The in vitro transfection efficiency of M-PEG-PE-Lipop-EGFP and Lipo-pEGFP in Kupffer cells was analyzed after 72 hours of transfection. Naked DNA was used as a negative control. As shown in the fluorescent images in , when compared with naked DNA and Lipo-pEGFP, M-PEG-PE-Lipo-pEGFP showed higher transfection efficiency at the different time intervals. The cells exhibited highest efficiency at 48 hours. Flow cytometry results showed that the highest in vitro efficiency of M-PEG-PE-Lipo-pEGFP at 48 hours was over 50%. Compared with around 30% efficiency for Lipo-pEGFP, the advantage of the modified vectors was significant.
Figure 7 Fluorescent images and flow cytometry analysis of Kupffer cells transfected with naked pEGFP; (A), Lipo/pEGFP (B), and M-PEG-PE-Lipo/pEGFP (C) at 24, 48, and 72 hours following transfection, respectively.
Abbreviations: M-PEG-PE, mannosylated polyethylene glycol-phosphatidylethanolamine; Lipo, Lipofectamine™; pEGFP, green fluorescence protein plasmid.
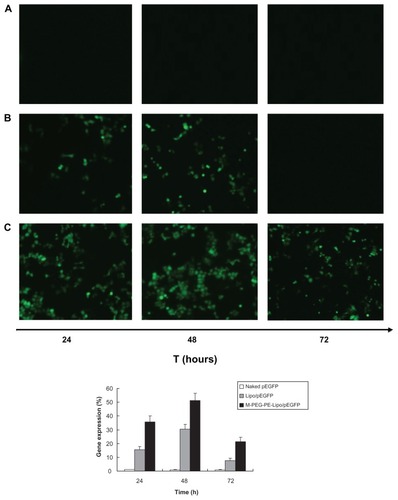
In vivo gene delivery
After intravenous injection of 1 mL of M-PEG-PE-Lipo-pEGFP, Lipo-pEGFP, and naked pEGFP, the rats were euthanized and the Kupffer cells were isolated at 48 hours. Flow cytometry was applied to quantitate the amount of cells that had been successfully transfected. As shown in , M-PEG-PE-Lipo-pEGFP showed a remarkably higher transfection efficiency than unmodified Lipo-pEGFP (P < 0.05) and naked pEGFP (P < 0.05).
Figure 8 Flow cytometry analysis of Kupffer cells transfected by naked pEGFP (A), Lipo-pEGFP (B), and M-PEG-PE-Lipo-pEGFP (C) in vivo. Gene expression was examined 48 hours following injection.
Abbreviations: M-PEG-PE, mannosylated polyethylene glycol-phosphatidylethanolamine; Lipo, Lipofectamine™; pEGFP, green fluorescence protein plasmid.

Discussion
Liposome-based delivery systems have long been identified as safe and effective drug carriers that are amenable to modification with cell-specific targeting ligands.Citation21 In regards to gene delivery, one of the most popular liposomes is Lipofectamine 2000 which is a proprietary formulation for the transfection of nucleic acids into cells.Citation33 In the present study, novel conjugated M-PEG-PE was synthesized and M-PEG-PE-modified Lipofectamine 2000 liposomes were investigated as a active targeting gene delivery system.
The investigation began with the synthesis of M-PEG-PE, in which mannose was the target moiety which could bind to the mannose receptor in the macrophage, and PEG-PE was the spacer linked into the surface of the liposomes. It is known that the method of connection between the carbohydrate and carrier surface through a flexible spacer influences the targeting ability and cellular uptake of the vectors.Citation15 For example, the length and flexibility of the spacer between the carbohydrate head group and liposome surface mainly influence the target specificity and uptake of liposome by macrophages. PEG-PE conjugates have been reported and TATp-PEG2000-PE conjugates have been used for the modification of liposomes to form active targeting vectors.Citation20 Therefore, PEG2000-PE was used as the anchor.
It is also known that the saccharide group density is an important factor for targeting efficiency.Citation34,Citation35 Generally, vectors modified with a higher content of sugar residues exhibit more efficient cellular recognition and internalization compared with those having a lower sugar density. Therefore, theoretically, the more M-PEG-PE ligands modified onto the carriers, the better the targeted delivery achieved. However, excessive modification could trigger the disintegration of liposomes;Citation36 pEGFP could leak out of the vector and cause a notable decrease of the gene loading capacity. A PicoGreen fluorometry assay was used to determine the appropriate modification rate of M-PEG-PE ligands and also to quantitate the amount of pEGFP carried by the optimally modified liposomes.
Nonmodified liposomes reached a gene loading quantity of 90.34% (). With an increase in the modification rate, the loading amount of pEGFP showed no obvious change until the ratio of the ligands was above 10%. The loading capacity was decreased to 10%–30%, indicating that the optimal modification rate would be 10% (ligands/ carrier, w/w). Therefore, 10% was finally determined as the ligand to carrier ratio and the gene loading amount of the modified liposomes was 90%. There was no significant difference in loading capacity between the modified and unmodified liposomes, indicating that binding of a mannose-containing ligand onto the liposome surface did not release DNA from the complexes.
Concanavalin A was the first legume lectin recognized as being mannose-specific, and is believed to play a role in recognition of mannose-containing vectors.Citation27,Citation37 It has been used extensively for the evaluation of glycoconjugates.Citation38–Citation40 In our research, a concanavalin A agglutination study was used to identify further that the M-PEG-PE ligands were successfully modified onto the Lipo-pEGFP surface. M-PEG-PE-Lipo-pEGFP showed an apparent increase in turbidity (), whereas the Lipofectamine 2000 and Lipo-pEGFP formulations appeared to show no significant increase in turbidity. This could be taken as evidence of the success of the mannose-containing ligands modified onto the liposome surface.
Macrophages play a major role in the immune response to foreign antigens, and targeting macrophages is one of the important therapeutic methods used to treat genetic metabolic diseases such as Gaucher’s disease and human immunodeficiency virus infection.Citation41 Kupffer cells are liver-specific resident macrophages that play an integral part in physiological homeostasis of the liver. They have significant roles in acute and chronic responses of the liver to bacterial and viral infections, toxic or carcinogenic attack, as well as mediating hepatotoxicity.Citation42 In this study, rat Kupffer cells were isolated and used as model cells for in vitro cytotoxicity evaluation and transfection analysis. The cell viabilities of the liposomes over the studied concentration range were between 80% and 100% compared with controls (). M-PEG-PE-Lipo-pEGFP showed no greater cytotoxicity than Lipo-pEGFP at all concentrations. When compared with naked DNA and Lipo-pEGFP, M-PEG-PE-Lipo-pEGFP had greater transfection efficiency at all time points (). This may be explained by the sugar-lectin-mediated active targeting mechanism. The M-PEG-PE-modified pEGFP-loaded liposomes were more likely to bind to Kupffer cells via the mannose receptor on the cells and deliver DNA more easily into the cells to express EGFP.
The gene delivery ability of the modified gene vector could be tested further in animal models. In vivo gene delivery and expression studies were used in rats. After intravenous injection, the rats were euthanized and the Kupffer cells were isolated at 48 hours and analyzed by flow cytometry. M-PEGPE-Lipo-pEGFP displayed remarkably higher transfection efficiency (43%) than unmodified Lipo-pEGFP (26%) and naked pEGFP (1.5%), as shown in . This could only be explained by the mannosylated PEG-PE-modified liposomes having the ability to target liver Kupffer cells and to deliver more genes into cells, displaying higher transfection efficiency. These observations strongly support the active targeting ability of M-PEG-PE-modified liposomes, and the resulting vectors would be very useful for in vivo gene delivery.
Conclusion
This study showed that mannose-mediated targeting can successfully transfer genes into cells expressing the mannose receptor. It could also demonstrate that having a targeting ligand, such as M-PEG-PE, in liposome formulations can significantly improve the transfection efficiency of a liposomal formulation. M-PEG-PE-Lipo-pEGFP had remarkably higher transfection efficiency both in vitro and in vivo than unmodified Lipo-pEGFP. It could be concluded that mannosylated PEG-PE could function as an excellent active targeting ligand, and M-PEG-PE-modified Lipofectamine 2000 could be a promising active targeting drug delivery vector.
Disclosure
The authors report no conflicts of interest in this work.
Supplementary in vivo gene delivery data
Details of the experiments and data analysis are as follows. Cells were washed in cold phosphate-buffered saline three times and the fluorescent intensity of the cells was determined using a flow cytometer equipped with a 488 nm argon laser for excitation. For each sample, about 10,000 events were collected and fluorescence was detected. Signals were amplified in the logarithmic mode for fluorescence to determine the positive events by a standard gating technique. The percentage of positive events was calculated as the events within the gate divided by the total number of events, excluding cell debris.
The pictures of flow cytometry analysis are shown in two ways, ie, as in and as a quadrantal rule diagram. The percentage of cells transfected with pEGFP appear at the upper right and lower right quadrants of the diagram.
The data in the quadrantal rule diagram for the in vivo study are as follows
Data on dose optimization are as follows (gene expression was analyzed at 48 hours after intravenous injection)
References
- BoulaizHMarchalJAPradosJMelguizoCAránegaANon-viral and viral vectors for gene therapyCell Mol Biol (Noisy-le-grand)200551132216171561
- ZhaoXBLeeRJTumor-selective targeted delivery of genes and anti-sense oligodeoxyribonucleotides via the folate receptorAdv Drug Deliv Rev20045681193120415094215
- AtkinsonHChalmersRDelivering the goods: viral and non-viral gene therapy systems and the inherent limits on cargo DNA and internal sequencesGenetica2010138548549820084428
- TorchilinVPMultifunctional nanocarriersAdv Drug Deliv Rev200658141532155517092599
- LiSDHuangLSurface-modified LPD nanoparticles for tumor targetingAnn N Y Acad Sci200610821817145918
- TorchilinVPMicellar nanocarriers: pharmaceutical perspectivesPharm Res200724111617109211
- BéduneauASaulnierPHindréFClavreulALerouxJCBenoitJPDesign of targeted lipid nanocapsules by conjugation of whole antibodies and antibody Fab’ fragmentsBiomaterials200728334978499017716725
- YuWLiuCLiuYZhangNXuWMannan-modified solid lipid nanoparticles for targeted gene delivery to alveolar macrophagesPharm Res20102781584159620422265
- YuWZhangNSurface modification of nanocarriers for cancer therapyCurr Nanosci200952123134
- ZamboniWCLiposomal, nanoparticle, and conjugated formulations of anticancer agentsClin Cancer Res200511238230823416322279
- SunWZhangNLiAZouWXuWPreparation and evaluation of N(3)-O-toluyl-fluorouracil-loaded liposomesInt J Pharm20083531–224325018155370
- ZhangQChengSXZhangXZZhuoRXWater soluble polymer protected Lipofectamine 2000/DNA complexes for solid-phase transfectionMacromol Biosci20099121262127119904725
- LiuJLMaQPHuangQDCationic lipids containing protonated cyclen and different hydrophobic groups linked by uracil-PNA monomer: synthesis and application for gene deliveryEur J Med Chem20114694133414121757268
- OpanasopitPPaecharoenchaiORojanarataTNgawhirunpatTRuktanonchaiUType and composition of surfactants mediating gene transfection of polyethylenimine-coated liposomesInt J Nanomedicine2011697598321720509
- YuWZhangNLiCSaccharide modified pharmaceutical nanocarriers for targeted drug and gene deliveryCurr Pharm Des200915323826383619925431
- Van VlerkenLEVyasTKAmijiMMPoly(ethylene glycol)-modified nanocarriers for tumor-targeted and intracellular deliveryPharm Res20072481405141417393074
- LukyanovANGaoZMazzolaLTorchilinVPPolyethylene glycol-diacyllipid micelles demonstrate increased accumulation in subcutaneous tumors in micePharm Res200219101424142912425458
- TorchilinVPRecent advances with liposomes as pharmaceutical carriersNat Rev Drug Discov20054214516015688077
- YangLWangLSuXQSuppression of ovarian cancer growth via systemic administration with liposome-encapsulated adenovirus-encoding endostatinCancer Gene Ther2010171495719609295
- SawantRMHurleyJPSalmasoS“SMART” drug delivery systems: double-targeted pH-responsive pharmaceutical nanocarriersBioconjug Chem200617494394916848401
- ReddyJAAbburiCHoflandHFolate-targeted, cationic liposome-mediated gene transfer into disseminated peritoneal tumorsGene Ther20029221542155012407426
- Penate MedinaOHaikolaMTahtinenMLiposomal tumor targeting in drug delivery utilizing MMP-2- and MMP-9-binding ligandsJ Drug Deliv201116051521490745
- WangSNDengYHXuHSynthesis of a novel galactosylated lipid and its application to the hepatocyte-selective targeting of liposomal doxorubicinEur J Pharm Biopharm2006621323816226883
- WijagkanalanWKawakamiSTakenagaMEfficient targeting to alveolar macrophages by intratracheal administration of mannosylated liposomes in ratsJ Control Release2008125212113018037185
- LiuCChenZYuWZhangNNovel cationic 6-lauroxyhexyl lysinate modified poly (lactic acid)-poly (ethylene glycol) nanoparticles enhance gene transfectionJ Colloid Interface Sci2011354252853521094495
- AdjimateraNKralTHofMBlagbroughISLipopolyamine-mediated single nanoparticle formation of calf thymus DNA analyzed by fluorescence correlation spectroscopyPharm Res20062371564157316783476
- BarreABourneYVan DammeEJPeumansWJRougePMannose-binding plant lectins: different structural scaffolds for a common sugar-recognition processBiochimie200183764565111522393
- CuiZGHanSJHuangLCoating of mannan on LPD particles containing HPV E7 peptide significantly enhances immunity against HPV-positive tumorPharm Res20042161018102515212168
- CuiZMumperRJTopical immunization using nanoengineered genetic vaccinesJ Control Release2002811–217318411992690
- KongFGeLLiuXHuangNZhouFMannan-modified PLGA nanoparticles for targeted gene deliveryInt J Photoenergy20112012926754
- OlynykJKClarkeSLIsolation and primary culture of rat Kupffer cellsJ Gastroenterol Hepatol19981388428459736181
- LiuHCaoHWuZYIsolation of Kupffer cells and their suppressive effects on T lymphocyte growth in rat orthotopic liver transplantationWorld J Gastroenterol200713223133313617589933
- GaoYGuWChenLXuZLiYA multifunctional nano device as non-viral vector for gene delivery: in vitro characteristics and transfectionJ Control Release2007118338138817321625
- ManagitCKawakamiSYamashitaFHashidaMEffect of galactose density on asialoglycoprotein receptor-mediated uptake of galactosylated liposomesJ Pharm Sci200594102266227516136555
- YeepraeWKawakamiSYamashitaFHashidaMEffect of mannose density on mannose receptor-mediated cellular uptake of mannosylated O/W emulsions by macrophagesJ Control Release2006114219320116876282
- BalazsDAGodbeyWTLiposomes for use in gene deliveryJ Drug Deliv2011201132649721490748
- SummerJBHowellSFThe identification of the hemagglutinin of the jack bean with concanavalin AJ Bacteriol193632222723716559945
- KizhakkedathuJNCreaghALShenoiRAHigh molecular weight polyglycerol-based multivalent mannose conjugatesBiomacromolecules201011102567257520804173
- MangoldSLCloningerMJBinding of monomeric and dimeric concanavalin A to mannose-functionalized dendrimersOrg Biomol Chem20064122458246516763692
- WolfendenMLCloningerMJMannose/glucose-functionalized dendrimers to investigate the predictable tunability of multivalent interactionsJ Am Chem Soc200512735121681216916131163
- YamadaMNishikawaMKawakamiSTissue and intrahepatic distribution and subcellular localization of a mannosylated lipoplex after intravenous administration in miceJ Control Release200498115716715245897
- KitaniHTakenouchiTSatoMYoshiokaMYamanakaNA novel isolation method for macrophage-like cells from mixed primary cultures of adult rat liver cellsJ Immunol Methods20103601–2475520600081