Abstract
Background
High-dose tissue-specific delivery of therapeutic agents would be a valuable clinical strategy. We have previously shown that repeated transcranial focused ultrasound is able to increase the delivery of Evans blue significantly into brain tissue. The present study shows that repeated pulsed high-intensity focused ultrasound (HIFU) can be used to deliver high-dose atherosclerotic plaque-specific peptide-1 (AP-1)-conjugated liposomes selectively to brain tumors.
Methods
Firefly luciferase (Fluc)-labeled human GBM8401 glioma cells were implanted into NOD-scid mice. AP-1-conjugated liposomal doxorubicin or liposomal doxorubicin alone was administered followed by pulsed HIFU and the doxorubicin concentration in the treated brains quantified by fluorometer. Growth of the labeled glioma cells was monitored through noninvasive bioluminescence imaging and finally the brain tissue was histologically examined after sacrifice.
Results
Compared with the control group, the animals treated with 5 mg/kg injections of AP-1 liposomal doxorubicin or untargeted liposomal doxorubicin followed by repeated pulsed HIFU not only showed significantly enhanced accumulation of drug at the sonicated tumor site but also a significantly elevated tumor-to-normal brain drug ratio (P < 0.001). Combining repeated pulsed HIFU with AP-1 liposomal doxorubicin or untargeted liposomal doxorubicin has similar antitumor effects.
Conclusion
This study demonstrates that targeted or untargeted liposomal doxorubicin, followed by repeated pulsed HIFU, is a promising high-dose chemotherapy method that allows the desired brain tumor region to be targeted specifically.
Introduction
Malignant glioma remains one of the most deadly types of tumor in humans, and glioblastoma multiforme is one of the most common forms of glioma. It is difficult to treat gliomas completely by surgical resection, and therefore radiotherapy and chemotherapy are used to remove residual microscopic tumor material.Citation1 Radiotherapy can induce developmental delays, so chemotherapy is recommended as the first line of treatment for children. Presently, chemotherapies are ineffective because many drugs do not reach therapeutic levels in the tumor tissue, either due to limited lipid solubility or to an inability to penetrate an intact blood-brain barrier.Citation2 Over the last decade, high-dose chemotherapy has been extensively investigated as first-line therapy in patients having brain tumors with a poor prognosis. Previous studies have demonstrated that first-line, high-dose chemotherapy may have a potential survival benefit compared with historical controls treated with standard-dose therapy.Citation3,Citation4
It has been reported that human brain tumor cell lines express high levels of plasma membrane interleukin-4 receptors.Citation5 Moreover, human brain tumors in situ overexpress interleukin-4 receptors compared with normal brain tissue.Citation6 In an immunodeficient xenograft model of human glioblastoma multiforme, an interleukin-4 receptor-targeted cytotoxin has been shown to have a remarkable antitumor effect.Citation7 These findings show that therapeutic agents that bind to interleukin-4 receptors may be a useful approach to tumor treatment.Citation8 Our previous work has demonstrated that the concentration of Evans blue in tumors and the tumor-to- normal brain ratio of Evans blue in the brain is elevated after blood-brain barrier disruption induced by pulsed-high intensity focused ultrasound (HIFU) in the presence of microbubbles. In these circumstances, repeated pulsed HIFU exposure is able to increase further the efficiency of Evans blue delivery into the brain.Citation9–Citation12 Another study has shown that magnetic resonance imaging (MRI)-guided focused ultrasound is able to achieve therapeutic levels of liposomal doxorubicin in the brain.Citation13 It has been pointed out in other studies that doxorubicin has potential when used clinically against both primary and metastatic brain tumors and that there is improved survival of glioma patients treated by direct intratumoral infusion of doxorubicin; furthermore, doxorubicin is useful against multiple tumor types.Citation14,Citation15 However, the concentration of chemotherapeutics required to achieve clinically effective cytotoxicity in tumors is limited by tissue toxicity and by the physiological barriers that prevent the delivery of drugs to the tumor.Citation16
Glioblastoma multiforme can be highly vascularized with a leaky vasculature, and thus may be amenable to liposome-based drug delivery systems that lead to enhanced drug deposition while limiting systemic drug exposure.Citation17 Receptor-targeted liposomal doxorubicin has been found to be effective in targeting glioma tumors in a brain tumor model.Citation18,Citation19 Here, we designed a ligand from atherosclerotic plaque-specific peptide-1 (AP-1) selected from phage display libraries and can locate atherosclerotic plaque tissue and bind to the interleukin-4 receptor because it has the same binding motif to the interleukin-4 protein.Citation8 Specifically, in order to allow more specific and efficient delivery of liposomal doxorubicin to brain tumors, an AP-1 actively targeted liposomal antitumor drug specific for interleukin-4 receptors, which is present on the cell membrane of malignant tumors, has been developed. Previous work has demonstrated that AP-1-labeled nanoparticles can be used for targeted drug delivery to tumor tissue.Citation20,Citation21 In this study, the integration of AP-1 liposomal doxorubicin and repeated pulsed HIFU was used to deliver and concentrate this high-dose chemotherapeutic agent in brain tumors by the usual systemic dosage. Our aim was to investigate the efficacy of this combined technology using an intracranial brain tumor model.
Materials and methods
Preparation of AP-1-labeled liposomal doxorubicin
Liposomal doxorubicin was prepared using a solvent injection method plus remote loading procedure. Briefly, hydrogenated soybean L-α-phosphatidylcholine (95.8 mg, Avanti Polar Lipids Inc, Alabaster, AL), cholesterol (31.9 mg, Sigma-Aldrich, St Louis, MO) and 1,2-distearoyl-sn-glycero- 3-phosphoethanolamine-N-[methoxy(polyethylene glycol)- 2000] (DSPE-PEG 2000, 31.9 mg, Avanti Polar Lipids) were dissolved and well mixed in 1 mL absolute ethanol at 60°C. The lipid containing ethanol was then injected into a 9 mL solution of 250 mM ammonium sulfate and stirred for one hour at 60°C. The mixture was then extruded five times through polycarbonate membranes, each of different pore size (Isopore Membrane Filter, Millipore, Billerica, MA; 0.4, 0.2, 0.1, and 0.05 μm) consecutively in that order at 60°C using high-pressure extrusion equipment (Lipex Biomembranes, Vancouver, BC); this produced smaller and smaller sized liposomes.
The liposome suspension was then dialyzed five times against large amounts of 10% sucrose containing 5 mM NaCl to remove free ammonium sulfate and ethanol. After dialysis, the liposome suspension was placed in a 50 mL glass bottle in 60°C water bath and was mixed with doxorubicin in a drug to lipid ratio of 1/9 (w/w), with a final doxorubicin concentration of 2 mg/mL in a 10% sucrose solution. The bottle was intermittently shaken in the water bath for one hour at 60°C and then cooled down to 4°C immediately. The final product was the liposomal doxorubicin.
Due to the presence of a thiol group on each cysteine of the AP-1 peptide (CRKRLDRNC), it is possible to couple AP-1 to liposomes by the thiol-maleimide reaction. Briefly, AP-1 peptide was conjugated to 1,2-distearoyl-sn-glycero- 3-phosphoethanolamine- N-[maleimide(polyethylene glycol)-2000] (DSPE-PEG2000-MAL, Avanti Polar Lipids) by adding AP-1 to a DSPE-PEG2000-MAL micelle solution at a 2:1 molar ratio, while still mixing at 4°C overnight. The free thiol groups were measured with 5,5′-dithiobis-(2-nitrobenzoic acid, Ellman’s reagent, Sigma- Aldrich) at an ultraviolet wavelength of 420 nm, which confirmed that most of the AP-1 was conjugated with the DSPE-PEG2000-MAL. The AP-1-conjugated DSPE-PEG2000 was transferred into the preformed liposomal doxorubicin at a 1.5% molar ratio of total lipid components and incubated at 60°C for one hour to obtain the AP-1 liposomal doxorubicin (). The composition and properties of each preparation are given in .
Table 1 Composition and properties of prepared liposomal doxorubicin and AP-1 liposomal doxorubicin
Figure 1 (A) Schematic representation of the AP-1-conjugated liposome. Liposomes were prepared containing maleimide functional polyethylene glycol chains. Maleimide was used to attach the AP-1 peptide through the thiol group on a cysteine. (B) Flow cytometric detection of the cell-surface interleukin-4 receptor on cloned human GBM8401-luc cells. (C) Linearity of measured bioluminescence versus GBM8401-luc cell number. GBM8401-luc cells were plated into 96-well dishes in triplicate in various numbers, and were then imaged by the in vivo imaging system. A strong correlation (R2 = 0.996) was observed between luciferase activity and cell numbers. (D) A luciferase image of the plated GBM8401-luc cells.
Abbreviations: IL-4R, interleukin-4 receptor; Lipo-Dox, liposomal doxorubicin.
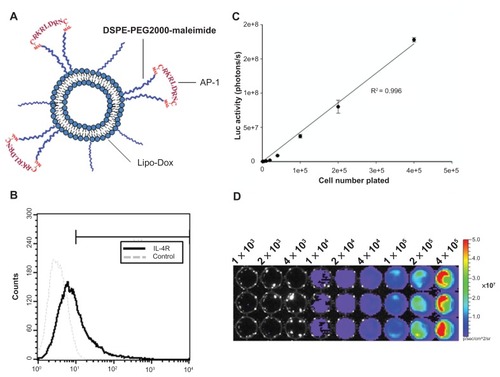
Intracranial glioma xenograft model
Male 6–8-week-old NOD-scid mice were anesthetized by intraperitoneal administration of pentobarbital at a dose of 40 mg/kg bodyweight. All procedures were performed according to ethical guidelines approved by our Animal Care and Use Committee. The mice were shaved on the head above the nape of the neck, scrubbed with betadine/alcohol, and immobilized in a Cunningham mouse/neonatal rat adaptor stereotactic apparatus (Stoelting, Wood Dale, IL). A 5 mm skin incision was made along the sagittal suture line and a burr hole drilled into the skull. Malignant human brain glioma cells (GBM8401) were transformed with the luciferase gene (GBM8401-luc), and 2 × 105 GBM8401-luc cells in 2 μL culture medium were then injected into the brains of the mice. The glioma cells were stereotactically injected into a single location in the left hemisphere (0.14 mm anterior and 2.0 mm lateral to the bregma) of each mouse at a depth of 3.5 mm from the brain surface. Next, the burr holes in the skull were sealed with bone wax and the wound was flushed with iodinated alcohol. Bioluminescence quantification of the tumor cells and the established tumor was determined by bioluminescence imaging.
Flow cytometric analysis
For detection of cell surface interleukin-4 receptor expression on GBM8401-luc cells, the cells were fixed by 1.5% para-formaldehyde and then stained with anti-interleukin-4 receptor monoclonal antibody (Sf 21-derived rhIL-4R; R&D Systems, Minneapolis, MN) and DyLight488 fluorescent-labeled rabbit antimouse antibody (Rockland Immunochemicals, Gilbertsville, PA). The expression level of the interleukin-4 receptor chain was analyzed by FACSCalibur (Becton Dickinson, San Jose, CA, ).
Enzyme-linked immunosorbent assay
Proteins for enzyme-linked immunosorbent assay (ELISA) analysis were isolated from fresh tissue using T-Per extraction reagent (Pierce Biotechnology Inc, Rockford, IL) according to the manufacturer’s recommendations, with the addition of the Halt protease inhibitor cocktail (Pierce Biotechnology Inc). Protein concentrations were determined using the Pierce 660 nm protein assay reagent (Pierce Biotechnology Inc). Protein levels in the tissue supernatant were measured by ELISA using either a mVEGF Quantikine (R&D Systems) kit or an ELISA kit for the human interleukin-4 receptor (Uscn Life Science Inc), following the manufacturer’s instructions. The results were corrected for total protein concentration and are reported as picograms of vascular endothelial growth factor/interleukin-4 receptor per microgram of protein.
Pulsed HIFU system and treatment
The pulsed HIFU sonications were generated by a 1.0 mHz single-element focused transducer (A392S, Panametrics, Waltham, MA). The focal zone of the therapeutic transducer was in the shape of an elongated ellipsoid, with a radial diameter (−6dB) of 3 mm and an axial length (−6dB) of 26 mm. The ultrasound-driving system and equipment setup were the same as used in our previous studies.Citation22 Ultrasound contrast agent (SonoVue, Bracco International, Amsterdam, Netherlands) was injected into the tail vein of the mice about 10 seconds before each sonication. The agent contains phospholipid-coated microbubbles at a concentration of 1 to 5 × 108 bubbles/mL, with the bubbles having a mean diameter of 2.5 μm. The ultrasound beam was delivered to one location in the left brain hemisphere centered on the tumor injection site. The following sonication parameters were used: an acoustic power of 2.86 W (corresponding to peak-negative pressure of 0.7 MPa) with an injection of 300 μL/kg ultrasound contrast agent; a pulse repetition frequency of 1 Hz; and a duty cycle of 5%. Each sonication time was 60 seconds. In order to increase drug accumulation in the tumor region effectively, all mice were sonicated a second time 20 minutes after the first sonication and injected intravenously with ultrasound contrast agent before each sonication.
Five days after tumor cell implantation, the glioma-bearing mice were divided into two groups. One group (n = 8) received liposomal doxorubicin followed by repeated pulsed HIFU. Another group (n = 8) were treated with AP-1 liposomal doxorubicin followed by repeated pulsed HIFU. The concentration of liposomes that was administered to the mice via tail vein injection corresponded to 5 mg/kg. A control group of six mice were injected with GBM8401 glioma cells but received no treatment.
Quantitative measurement of doxorubicin
Animals were put into a state of deep anesthesia with an overdose of pentobarbital. The brain was perfused by the transcardiac method with normal saline for 3.5 hours after doxorubicin administration in order to flush out unabsorbed doxorubicin from the cerebral vessels. The site of tumor tissue was harvested along with its contralateral counterpart as a control. Doxorubicin was extracted from the tumor and control tissues by homogenization and refrigeration for 24 hours in 20 volumes of acidified ethanol at 4°C. Tissues were centrifuged at 16,000 g for 25 minutes at 4°C and the supernatant stored at −20°C until the fluorometric assay.Citation23 The concentration of doxorubicin present was measured using a spectrophotometer (PowerWave 340, BioTek, Winooski, VT; excitation 480 nm and emission 590 nm) and the value was determined by taking the average of at least three fluorometric readings. The doxorubicin present in the tissue samples was quantified using a linear regression standard curve derived from seven concentrations of doxorubicin; the amount of doxorubicin is denoted in absorbance per gram of tissue.
Bioluminescence imaging
To assess the cultured GBM8401-luc cells, we imaged the luciferase signal by adding phosphate buffer solution or colorless Opti-MEM® medium (Invitrogen) with luciferin at a concentration of 0.15 mg/mL. Cells were imaged 10 minutes after luciferin administration (). Tumor size was quantified by analysis of their biophotonic images obtained from 5 days to 16 days after tumor implantation. The mice received injections of 4.29 mg per mouse of freshly prepared luciferin substrate suspended in phosphate buffer solution. After induction of anesthesia with isoflurane (1.5 L oxygen + 4% isoflurane per minute), the mice were imaged using an in vivo imaging system (Xenogen, Palo Alto, CA); this occurred 10 minutes after the intraperitoneal injection of luciferin using a one-minute acquisition time in small binning mode. Luciferase activity was viewed and quantified using Living Image software from Xenogen for a region of interest that encompassed the head of the mouse after anesthesia and administration of luciferin substrate.
Magnetic resonance imaging
MRI was performed using a 3-T MRI system (TRIO 3-T MRI, Siemens Magnetom, Germany). The mice were anesthetized with isoflurane mixed with oxygen during the imaging procedure. A loop coil (Loop Flex Coil, approximately 4 cm in diameter) for RF reception was used. Tumor progression was evaluated by means of T2-weighted images obtained on day 12 after tumor implantation. The imaging plane was located across the center of the tumor injection site. Parameters for T2-weighted images were as follows: repetition time/echo time 3500/75 msec; matrix 125 × 256; field of view 25 × 43 mm; and section thickness 1.0 mm.
Histological examination
After MRI scanning, the brains of the mice were prepared for histological assessment. Two tumor-bearing mice each from the repeated pulsed HIFU plus AP-1 liposomal doxorubicin group, from the repeated pulsed HIFU plus the liposomal doxorubicin treatment group, and the control group were sacrificed 12 days after tumor implantation for histological assessment. The mouse was perfused with saline and 10% neutral buffered formalin. Tissue sections from the mouse brains were fixed in paraformaldehyde and the sections stained with hematoxylin and eosin (Thermo-Scientific, Waltham, MA). Photomicrographs of the hematoxylin and eosin-stained tissue sections were obtained using a Mirax Scan digital microscope slide scanner (Carl Zeiss, Mirax 3D Histech) with a Plan-Apochromatic 20/0.8 objective. The serial histology images were annotated using Panoramic Viewer software.
Statistical analysis
All values are shown as the mean ± standard error of the mean. Statistical analysis was performed using the unpaired Student t-test. Statistical significance was defined as a P value ≤0.05.
Results
Concentration of doxorubicin and intensity of pulsed HIFU
shows the growth of tumor cells in the control mice with no treatment (n = 3). Compared with cell numbers on day 5 after implantation, there was a significant increase in tumor cell numbers on days 12, 16, and 20. The number of tumor cells showed a significant decrease when the mice received a single dose of liposomal doxorubicin at 10 mg/kg compared with a dose of 5 mg/kg. In addition, there was no significant difference in the bodyweight change after treatment with these two doses of drug (). In the normal brains, pulsed HIFU exposure (2.86 W or 4.29 W) significantly increased the doxorubicin concentration in the brain (). To reduce the systemic toxicity of the drug and tissue damage induced by sonication, doxorubicin accumulation was therefore quantified in tumor-bearing brains after injection of 5 mg/kg doxorubicin followed by 2.86 W of repeated sonications. indicates that interleukin-4 receptor expression by the tumor tissue and vascular endothelial growth factor expression by the surrounding tissue did not significantly change after pulsed HIFU sonication of 2.86 W.
Figure 2 Influence of the concentration of liposomal doxorubicin on tumor progression and bodyweight. (A) Control mice are indicated by ●. Tumor-bearing mice were treated on day 5 with different doses of liposomal doxorubicin (▾, 5 mg/kg; ○, 10 mg/kg). Each point consisted of three mice. *Significant difference in control mice over the three days following cell numbers on day 5 after implantation. There was a significant difference between the tumors treated with liposomal doxorubicin at doses of 5 mg/kg or 10 mg/kg and control tumors on day 12 after implantation. (B) Body weight change (relative to day 1) of tumor-bearing mice treated with different doses of liposomal doxorubicin.
Notes: *P < 0.05; #P < 0.05; n = 3.
Abbreviation: Lipo-Dox, liposomal doxorubicin.
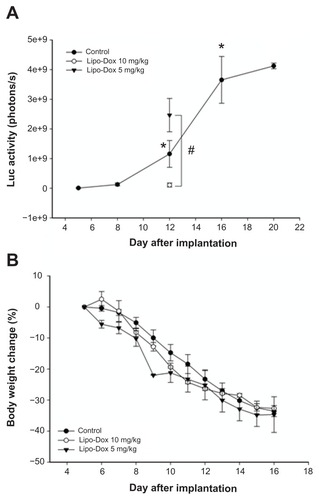
Figure 3 (A) Concentration of doxorubicin delivered to the brain as a function of acoustic power. Compared with the nonsonicated normal brain, there was a significant difference for sonicated brains at 2.86 W or 4.29 W. **,#/##Significant difference compared with the nonsonicated brain and the brain sonicated at 1.43 W, respectively. The concentrations of interleukin-4 receptor protein and vascular endothelial growth factor protein were evaluated in the brain tumor and the neighboring brain, respectively. There was no significant difference in protein expression of the interleukin-4 receptor (B) or vascular endothelial growth factor (C) after pulsed HIFU exposure.
Notes: #P < 0.05; **P < 0.01; ##P < 0.01; n = 3).
Abbreviations: Lipo-Dox, liposomal doxorubicin; HIFU, high-intensity focused ultrasound; VEGF, vascular endothelial growth factor.
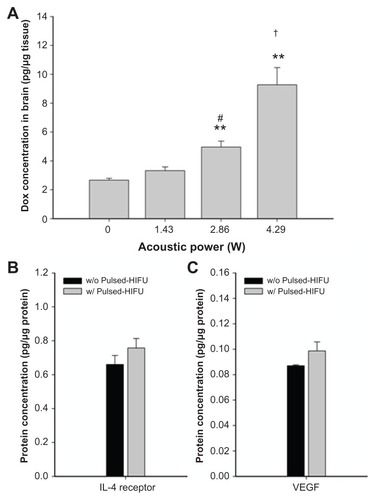
Doxorubicin deposition in brains and tumors
We used spectrophotometry to measure the average tumor doxorubicin concentration (in micrograms per gram of tissue) for three mice from each group. Doxorubicin was extracted from the tumor and contralateral control regions of the harvested brains treated with untargeted liposomal doxorubicin or AP-1 liposomal doxorubicin. shows the mean concentration of doxorubicin per unit mass for the brain tumors and the contralateral normal brain tissues with or without repeated sonication after untargeted liposomal doxorubicin or AP-1 liposomal doxorubicin administration. Not only was the concentration of doxorubicin in the nonsonicated tumor significantly greater than that in the contralateral normal brain region, but it was also found that the concentration of doxorubicin significantly increased at the tumor site after repeated sonication compared with the nonsonicated tumor for the two treatments. Repeated pulsed HIFU exposure administered after the drugs were introduced increased the doxorubicin concentration in the tumor by 441% and 374% for untargeted liposomal doxorubicin and AP-1 liposomal doxorubicin, respectively. Additionally, the concentration of doxorubicin was significantly greater at the tumor site with the untargeted liposomal doxorubicin followed by repeated sonication than for the nonsonicated tumor treated with targeted liposomal doxorubicin without sonication (P < 0.05). Compared with the control tumor, there was a significant increase in the derived tumor-to-contralateral brain ratios for the repeatedly sonicated tumor treated with either drug (). Importantly, however, the derived tumor-to-contralateral brain ratio was significantly greater after repeated sonication for the untargeted liposomal doxorubicin group than for the targeted liposomal doxorubicin group without sonication.
Figure 4 (A) Measurements of untargeted liposomal doxorubicin and AP-1-conjugated liposomal doxorubicin in the contralateral normal brain and the brain tumor without and with repeated sonication. Compared with the contralateral normal brain, there was a significant difference between the control tumors and the repeatedly sonicated tumors with the two drugs. The concentrations of doxorubicin in the brain tumors after repeated sonication were significantly higher than in brain tumors without sonication. *,***,###Significant difference compared with contralateral normal brain and the nonsonicated brain tumor, respectively. (B) Derived tumor-to-contralateral brain ratios without and with repeated sonication after drug administration. The values of the ratios are significantly elevated after application of repeated sonication.
Notes: *P < 0.05; ***P < 0.001; ###P < 0.001; n = 3.
Abbreviations: AP-1, atherosclerotic plaque-specific peptide-1; Lipo-Dox, liposomal doxorubicin; HIFU, high-intensity focused ultrasound.

Antitumor effect on tumors treated with untargeted or targeted liposomal doxorubicin followed by repeated sonication
The control tumors and the effect of tumors treated on day 5 by untargeted liposomal doxorubicin or targeted liposomal doxorubicin in combination with repeated pulsed HIFU on tumor progression were monitored by bioluminescence imaging over time (). Tumor cells spread rapidly in the untreated control mice (, top). When the intracranial brain tumors were treated with untargeted liposomal doxorubicin or targeted liposomal doxorubicin, in both cases followed by repeated pulsed HIFU, a similar pattern of tumor progression was followed. Tumor treatment by liposomal doxorubicin or AP-1 liposomal doxorubicin with repeated sonication significant slowed the growth of the tumors by day 12 after implantation (). Both treatment protocols were associated with no statistically significant decrease in body weight compared with the animals with untreated control tumors ().
Figure 5 Longitudinal bioluminescence imaging of the brain tumors was monitored from 5 to 16 days after implantation. Firefly luciferase-labeled GBM8401 cells had been implanted into the left forebrain of NOD-scid mice which received no treatment (control), post-treatment with repeated pulsed HIFU after liposomal doxorubicin on day 5, or post-treatment with repeated pulsed HIFU after AP-1 liposomal doxorubicin injection on day 5.
Abbreviations: AP-1, atherosclerotic plaque-specific peptide-1; Lipo-Dox, liposomal doxorubicin; HIFU, high-intensity focused ultrasound.
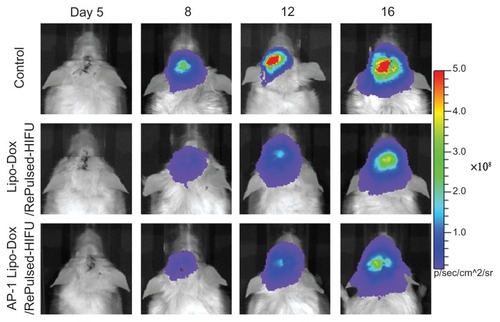
Figure 6 (A) Analysis of increases in tumor size (relative to day 5) is based on data obtained from bioluminescence images in . Compared with the control tumor mice, * and # denote a significant difference for untargeted liposomal doxorubicin with repeated sonication and AP-1 liposomal doxorubicin with repeated sonication, respectively. (B) Bodyweight change (relative to day 5) of tumor-bearing mice treated by untargeted liposomal doxorubicin with repeated sonication and AP-1 liposomal doxorubicin with repeated sonication.
Notes: *P < 0.05; #P < 0.05.
Abbreviations: AP-1, atherosclerotic plaque-specific peptide-1; Lipo-Dox, liposomal doxorubicin; HIFU, high-intensity focused ultrasound.
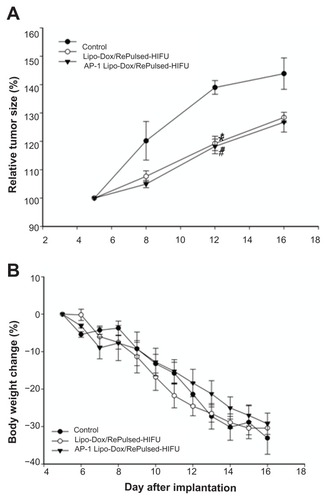
MRI and histological evaluation
The effects of the various treatment protocols on tumor progression were monitored by MRI and also evaluated by hematoxylin and eosin staining on day 12 after implantation (). Based on the MRI and histology, tumor progression was found to be consistent with the bioluminescence imaging () and no significant difference in the tumor size was found between the treatment groups.
Figure 7 Twelve days after tumor implantation, three mice each from the control group, the untargeted liposomal doxorubicin with repeated sonication group, and the AP-1 liposomal doxorubicin with repeated sonication group were imaged by T2-weighted magnetic resonance imaging (A, coronal view; B, axial view), and then sacrificed for hematoxylin and eosin (C) histological examination (scale bar, 2000 μm).
Abbreviations: AP-1, atherosclerotic plaque-specific peptide-1; Lipo-Dox, liposomal doxorubicin; HIFU, high-intensity focused ultrasound.
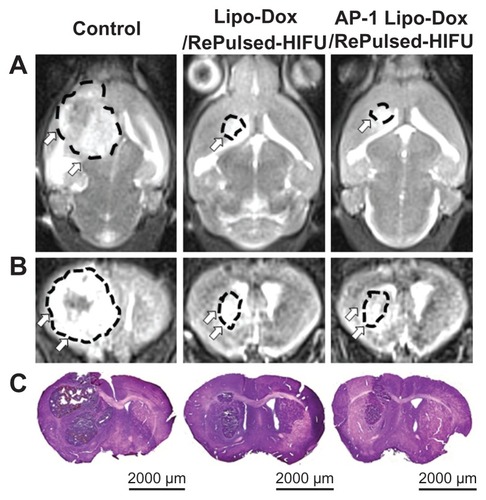
Discussion
This study was designed to investigate the applicability of repeated pulsed HIFU exposures when treating brain tumors with high-dose chemotherapeutic agents. This study shows that repeated sonications could significantly increase the concentration of drugs in the brain tumor. Combining the repeated sonications with either untargeted liposomal doxorubicin or targeted liposomal doxorubicin was found to have a similar and significant antitumor effect.
Several studies have indicated that poor distribution and limited penetration by doxorubicin into solid tumors are the main causes of its deficiency as a therapeutic agent.Citation24,Citation25 Long- circulating chemotherapies delivered through lipid nanoparticles have been approved to deliver drugs into brain tumors passively by the enhanced permeability and retention effect.Citation26 It has been demonstrated that focused ultrasound is able to improve the therapeutic efficacy of liposomal doxorubicin in a breast cancer tumor model.Citation27 Previous studies have demonstrated that repeated sonication can significantly increase the efficiency of drug delivery when microbubbles are present for sonication.Citation11,Citation28 Our strategy is to use repeated pulsed HIFU to deliver and concentrate the high-dose chemotherapeutic agent into the brain tumor at a minimal systemic dosage (). Moreover, repeated sonications not only significantly enhance the concentration of doxorubicin but also significantly elevate the tumor-to-normal brain ratio in the sonicated tumor tissue.
In our in vitro pilot study, colocalized expression of tumor cells was significantly greater for treatment with AP-1 liposomal doxorubicin than for treatment with untargeted liposomal doxorubicin. shows that repeated pulsed HIFU administered after AP-1 liposomal doxorubicin was introduced gave the highest doxorubicin concentration and tumor-to-normal brain ratio at the sonicated tumor. However, there were no significant differences between the group treated with AP-1 liposomal doxorubicin and a similar approach using untargeted liposomal doxorubicin. This is consistent with the similar antitumor effects seen in . One possible reason could be that drug delivery was limited by the blood-brain barrier in the in vivo study. Therefore, the targeting ability of AP-1 would not be obvious due to low drug concentration in the tumor tissue. Additionally, the concentration of doxorubicin was significantly greater at the tumor site with the untargeted liposomal doxorubicin followed by repeated sonication than for the nonsonicated tumor treated with targeted liposomal doxorubicin alone (P < 0.05). Thus, our results show that doxorubicin accumulation in the tumor occurring with the untargeted drug followed by repeated pulsed HIFU is significantly higher than with targeted doxorubicin alone. In parallel, the tumor-to-normal brain ratio is also significantly elevated by repeated sonication compared with targeted liposomal doxorubicin without sonication (). Therefore, liposomal doxorubicin delivery to the tumor by repeated sonication is much more efficient than that obtained using IL-4 receptor-targeted liposomal doxorubicin without sonication.
Chemical modification of drugs and biological agents are methods often used to help an agent cross through the blood-brain barrier into a brain tumor. However, by traditional means, in order that sufficient drugs can be delivered to the tumor tissue, the drug dose often needs to be at toxic levels. Pulsed HIFU is a physical assistance method that enhances delivery of chemotherapeutic agents to the targeted region of the brain. There are several mechanisms that may be involved in enhancing drug propagation through the blood-brain barrier, such as blood-brain barrier disruption or widening of the intercellular gaps, both of which can increase transport into the tumor site. Furthermore, pulsed HIFU can shorten peak tumor uptake times and increase peak tumor uptake values compared with unsonicated tumors.Citation29 Our previous study has demonstrated that the blood-brain barrier was transiently disrupted by pulsed HIFU.Citation9 In the present study, regardless of whether untargeted or targeted drugs are used, focused ultrasound offers a local and efficient method for delivering either drug (). High-dose chemotherapy has yielded favorable results when treating brain metastases or primary brain tumors.Citation30 Using pulsed HIFU to allow delivery of high-dose therapeutic agents to brain tumors using standard chemotherapeutic doses is an innovative approach to treatment.
This study demonstrates that repeated pulsed HIFU is able to deliver high-dose chemotherapeutic drugs to brain tumors and improve the antitumor effect of the drugs at minimal systemic dosage. Repeated pulsed HIFU seems to be a good method of achieving local high-dose chemotherapy for malignant glioma or other brain diseases without increasing systemic toxicity.
Acknowledgments
This study was supported by grants from the National Science Council of Taiwan (100-2321-B-010-010 and 99-- 2321-B-010-017), Cheng Hsin General Hospital Foundation (100F117CY25), Veterans General Hospital University System of Taiwan Joint Research Program (VGHUST100-G1-3-3 and V100E6-007), Yen Tjing Ling Medical Foundation (CI-100-17), and Department of Health of Taiwan (DOH101- TD-PB-111-TM012 and DOH101-TD-C-111-007).
Disclosure
The authors report no conflicts of interest in this work.
References
- DeenDFChiarodoAGrimmEABrain tumor working group report on the 9th international conference on brain tumor research and therapy Organ System Program, National Cancer InstituteJ Neurooncol1993162432727905510
- Cordon-CardoCO’BrienJPCasalsDMultidrug-resistance gene (P-glycoprotein) is expressed by endothelial cells at blood-brain barrier sitesProc Natl Acad Sci U S A1989866956982563168
- MotzerRJMazumdarMGulatiSCPhase II trial of high-dose carboplatin and etoposide with autologous bone marrow transplantation in first-line therapy for patients with poor-risk germ cell tumorsJ Natl Cancer Inst199385182818357693955
- MotzerRJMazumdarMBajorinDFBoslGJLynPVlamisVHigh-dose carboplatin, etoposide, and cyclophosphamide with autologous bone marrow transplantation in first-line therapy for patients with poor-risk germ cell tumorsJ Clin Oncol199715254625529215823
- PuriRKLelandPKreitmanRJPastanIHuman neurological cancer cells express interleukin-4 (IL-4) receptors which are targets for the toxic effects of IL4-pseudomonas exotoxin chimeric proteinInt J Cancer1994585745818056454
- JoshiBHLelandPAsherAPraysonRAVarricchioFPuriRKIn situ expression of interleukin-4 (IL-4) receptors in human brain tumors and cytotoxicity of a recombinant IL-4 cytotoxin in primary glioblastoma cell culturesCancer Res2001618058806111719427
- HusainSRBehariNKreitmanRJPastanIPuriRKComplete regression of established human glioblastoma tumor xenograft by interleukin-4 toxin therapyCancer Res199858364936539721874
- HongHYLeeHYKwakWPhage display selection of peptides that home to atherosclerotic plaques: Il-4 receptor as a candidate target in atherosclerosisJ Cell Mol Med2008122003201419012727
- YangFYWangHELinGLMicro-SPECT/CT-based pharmacokinetic analysis of 99 mtc-diethylenetriaminepentaacetic acid in rats with blood-brain barrier disruption induced by focused ultrasoundJ Nucl Med20115247848421321259
- YangFYHorngSCLinYSKaoYHAssociation between contrast-enhanced MR images and blood-brain barrier disruption following transcranial focused ultrasoundJ Magn Reson Imaging20103259359920815056
- YangFYLinYSKangKHChaoTKReversible blood-brain barrier disruption by repeated transcranial focused ultrasound allows enhanced extravasationJ Control Release201115011111621070825
- YangFYLiuSHHoFMChangCHEffect of ultrasound contrast agent dose on the duration of focused-ultrasound-induced blood-brain barrier disruptionJ Acoust Soc Am20091263344334920000948
- TreatLHMcDannoldNVykhodtsevaNZhangYTamKHynynenKTargeted delivery of doxorubicin to the rat brain at therapeutic levels using MRI-guided focused ultrasoundInt J Cancer200712190190717437269
- WalterKATamargoRJOliviABurgerPCBremHIntratumoral chemotherapyNeurosurgery199537112811458584154
- CummingsJMcArdleCSStudies on the in vivo disposition of adriamycin in human tumours which exhibit different responses to the drugBr J Cancer1986538358383718837
- FloydJDNguyenDTLobinsRLBashirQDollDCPerryMCCardiotoxicity of cancer therapyJ Clin Oncol2005237685769616234530
- AllenTMCullisPRDrug delivery systems: Entering the mainstreamScience20043031818182215031496
- MadhankumarABSlagle-WebbBWangXEfficacy of interleukin- 13 receptor-targeted liposomal doxorubicin in the intracranial brain tumor modelMol Cancer Ther2009864865419276162
- MadhankumarABSlagle-WebbBMintzASheehanJMConnorJRInterleukin-13 receptor-targeted nanovesicles are a potential therapy for glioblastoma multiformeMol Cancer Ther200653162316917172420
- KimJHBaeSMNaMHFacilitated intracellular delivery of peptide-guided nanoparticles in tumor tissuesJ Control Release September 162011 [Epub ahead of print.]
- WuXLKimJHKooHTumor-targeting peptide conjugated pH-responsive micelles as a potential drug carrier for cancer therapyBioconjug Chem20102120821320073455
- YangFYLinGLHorngSCPulsed high-intensity focused ultrasound enhances the relative permeability of the blood-tumor barrier in a glioma-bearing rat modelIEEE Trans Ultrason Ferroelectr Freq Control20115896497021622052
- BachurNRMooreALBernsteinJGLiuATissue distribution and disposition of daunomycin (ncs-82151) in mice: Fluorometric and isotopic methodsCancer Chemother Rep19705489945527015
- PrimeauAJRendonAHedleyDLilgeLTannockIFThe distribution of the anticancer drug doxorubicin in relation to blood vessels in solid tumorsClin Cancer Res2005118782878816361566
- LankelmaJDekkerHLuqueFRDoxorubicin gradients in human breast cancerClin Cancer Res199951703170710430072
- SharmaUSSharmaAChauRIStraubingerRMLiposome-mediated therapy of intracranial brain tumors in a rat modelPharm Res1997149929989279878
- FrenkelVEtheringtonAGreeneMDelivery of liposomal doxorubicin (Doxil) in a breast cancer tumor model: Investigation of potential enhancement by pulsed-high intensity focused ultrasound exposureAcad Radiol20061346947916554227
- BekeredjianRChenSFrenkelPAGrayburnPAShohetRVUltrasound-targeted microbubble destruction can repeatedly direct highly specific plasmid expression to the heartCirculation20031081022102612912823
- KhaibullinaAJangBSSunHPulsed high-intensity focused ultrasound enhances uptake of radiolabeled monoclonal antibody to human epidermoid tumor in nude miceJ Nucl Med20084929530218199622
- KollmannsbergerCNicholsCBambergMFirst-line high-dose chemotherapy +/− radiation therapy in patients with metastatic germ-cell cancer and brain metastasesAnn Oncol20001155355910907948