Abstract
Ultrasound (US) sonication with microbubbles (MBs) has the potential to disrupt blood vessels and enhance the delivery of drugs into the sonicated tissues. In this study, mouse ear tumors were employed to investigate the therapeutic effects of US, MBs, and pegylated liposomal doxorubicin (PLD) on tumors. Tumors started to receive treatments when they grew up to about 15 mm3 (early stage) with injection of PLD 10 mg/kg, or up to 50 mm3 (medium stage) with PLD 6 (or 4) mg/kg. Experiments included the control, PLD alone, PLD + MBs + US, US alone, and MBs + US groups. The procedure for the PLD + MBs + US group was that PLD was injected first, MB (SonoVue) injection followed, and then US was immediately sonicated on the tumor. The results showed that: (1) US sonication with MBs was always able to produce a further hindrance to tumor growth for both early and medium-stage tumors; (2) for the medium-stage tumors, 6 mg/kg PLD alone was able to inhibit their growth, while it did not work for 4 mg/kg PLD alone; (3) with the application of MBs + US, 4 mg/kg PLD was able to inhibit the growth of medium-stage tumors; (4) for early stage tumors after the first treatment with a high dose of PLD alone (10 mg/kg), the tumor size still increased for several days and then decreased (a biphasic pattern); (5) MBs + US alone was able to hinder the growth of early stage tumors, but unable to hinder that of medium stage tumors. The results of histological examinations and blood perfusion measurements indicated that the application of MBs + US disrupts the tumor blood vessels and enhances the delivery of PLD into tumors to significantly inhibit tumor growth.
Introduction
The aim of chemotherapy is to deliver anticancer drug(s) to the targeted tumors with sufficiently high doses and to produce minimum side effects on normal tissue.Citation1 Drug delivery with nanotechnology can result in preferential transport of drugs to tumors, owing to the enhanced permeability and retention effect.Citation2 Vasculature plays a crucial role in tumor growth, metastasis, and drug delivery. The therapeutic strategy of nanodrugs is based on tumor microenvironment and on morphological and functional characteristics of tumor vasculature.Citation3,Citation4 Tumors commonly secrete vascular endothelial growth factor, and their vessels usually have wider intercellular junctions than normal vessels do, and many tumor vessels have fenestrated structures.Citation5–Citation7 The abnormal organization and structure of the tumor vasculature results in tortuous and leaky vessels and rather heterogeneous blood flow.Citation8 Tumor vessels with different morphological characteristics coexist in the same tumor, and a previous studyCitation9 showed that the process of tumor growth from initiation to necrosis could be classified into four characteristic stages and that tumor vascular morphology drastically changed according to tumor growth.
An effective drug accumulation in tumors can lead to a significant therapeutic response for the outcome of treatment. Citation10,Citation11 Strategies that can further increase drug availability and therapeutic responses in tumor inhibition are needed. Ultrasound (US) sonication with microbubbles (MBs) could noninvasively enhance the transport of therapeutic agents to targeted tissues.Citation12,Citation13 With US sonication right after an injection of MBs, the MBs in the vasculature within the acoustic beam are interacting with the ultrasound waves. The interaction of ultrasound waves with MBs may cause oscillation and cavitation of the bubbles and lead to the rupture of vascular walls.Citation14–Citation16 For overcoming the difficulties of drug delivery posed by vascular walls, US sonication in the presence of MBs can produce ruptured openings to increase vascular permeability of sonicated tissues and permit a specific and effective cellular uptake.Citation17–Citation20 Studies have recently demonstrated that ultrasound-mediated chemotherapeutics with MBs showed promising potential for animal tumor treatment.Citation19,Citation20 The therapeutic drug concentration increased specifically in the sonicated region and significantly suppressed the tumor growth.
Pegylated liposomal doxorubicin (PLD) is able to avoid the recognition of the reticuloendothelial system and possesses long-circulating properties to improve the delivery of free drug.Citation21–Citation23 The average size of PLD is approximately 100 nm, which is small enough for the PLD to extravasate through the leaky vasculature to achieve passive accumulation in tumor tissues. Previously, we have shown that focused US (FUS) sonication with MBs can significantly enhance the delivery of nanoparticles into tumor tissues.Citation15,Citation16 The results indicated that an injection of MBs followed by pulsed-FUS sonication is promising for nanodrug delivery in the sonicated tumor tissues. There have been no reports relating to the influence of the changes of vascular permeability through ruptured openings on the tumor treatment at different tumor growth stages. In the present study, we employ a mouse-ear tumor model to investigate the influences of US sonication in the presence of MBs on the therapeutic responses of early and medium-stage tumors. Different PLD dosages were also considered, and histological examination and blood perfusion measurement were used to study the tumors’ physiological changes due to US sonication with MBs.
Materials and methods
Reagents
The PLD (Doxil) was obtained from Alza (Bedford, MA). The US contrast agent (UCA) (SonoVue) used as MBs was purchased from Bracco (Amsterdam, Netherlands). Other chemicals, if not specified, were reagent grade from Aldrich-Sigma (St, Louis, MO).
Cell culture and animal model
Mouse colorectal adenocarcinoma CT-26 cells were received from American Type Culture Collection (ATCC, MD). The CT-26 cells were cultured in the Roswell Park Memorial Institute medium supplemented with 10% fetal bovine serum in a 37°C, 5% CO2 incubator for 24 hours with complete cell attachment. Cell number and viability were counted by using a hemocytometer with trypan blue exclusion test.
Male, 6-week-old BALB/c mice weighing 20–25 g were used in this study. To produce ear tumors for experiments, CT-26 cells (106 cells/50 μL) were inoculated subcutaneously into both sides of the mouse ears. Before the experiments, tumor size was selected according to tumor volume (TV) by the measurement of length (L), width (W), and height (H) with a caliper and using the formula TV=π / 6L×W × H.Citation24 All tumors were measured three times per week. Seven or 10 days after tumor cell inoculation, mice bearing tumors with an initial size of about 15 mm3 (early stage) or 50 mm3 (medium stage) were selected for the experiments. The mice were anesthetized with a combined solution of ketamine (30 mg/kg) and acepromazine (0.75 mg/kg) by intraperitoneal injection. The experiment procedure met the criteria outlined by the Institution of Animal Care and Use Committee of National Taiwan University, and the mice were handled according to the guidelines in The Handbook of Laboratory Animal Center, National Taiwan University. All experiments were designed to minimize the animals’ suffering.
Ultrasound sonication system
The sonication was conducted either with a 1.0-MHz US transducer (Sonitron 2000; Rich Mar, Inola, OK; output power intensity 2 W/cm2, duty cycle 50%, pulse length 10 ms, pulse-repetition frequency 50 Hz, and sonication duration 60 seconds) for early stage tumors or with a 1.0- MHz FUS transducer (A392S; Panametrics, Waltham, MA; diameter 38 mm, curvature radius 63.5 mm) for medium-stage tumors, which was driven by a power amplifier (150A250A; Amplifier Research, Souderton, PA) connected to both a function generator (33220A; Agilent, Palo Alto, CA) and a power meter/sensor module (4421; Bird, Cleveland, OH). A removable cone (inner diameters for top and bottom 12 and 38 mm, respectively; cone length 58 mm) filled with distilled and degassed water was mounted on the bottom of the FUS transducer. The tip of the cone was firmly covered with a polyurethane membrane to ensure no air bubbles in the water, and the cone was used to guide the acoustic beam to the tumor region. The center of the focal zone was about 5.3 mm away from the cone tip. The acoustic pressure at the focal point was 0.6 MPa, measured by a needle hydrophone (HPM1/1; Precision Acoustics, Dorchester, UK), and the parameters of FUS sonication used were 60-second sonication duration, a 10-ms pulse length, a 50% duty cycle, and a 50-Hz pulse-repetition frequency. A 3-mm-thick acoustic transmission gel (Pharmaceutical Innovations, Newark, NJ) was mantled to the skin over each tumor, and then the cone tip was immersed in the gel and targeted on the tumor. The cone with US transducer was then circularly scanned above the tumor and sonicated, as shown in .
Figure 1 Experimental arrangement. The acoustic beam from the ultrasound transducer to sonicate mouse ear tumors was guided by a cone filled with degassed water. A 3-mm-thick ultrasound transmission gel was mantled over the ear skin and the acoustic beam was targeted on the tumor. The ultrasound beam was circularly scanned during sonication.

Experimental designs
We conducted the experiments to investigate the influence of US sonication with MBs on the tumor growth in mouse-ear tumors treated with anticancer nanodrug. In this study, a US contrast agent (SonoVue; phospholipid-coated MBs mean diameter 2.5 μm and concentration 2–5 × 108 bubbles/mL) was used as MBs. If not specified, the injected dose of MBs was 100 μL/kg.
Growth response for early stage tumors treated with 10 mg/kg of PLD
To study the effect of US sonication with MBs on the therapeutic response of early stage tumors, we selected a small US probe (3 mm diameter at the tip) and an initial size of treated tumors of about 15 mm3 (7 days after inoculation). Experimental groups included the control (n = 3), PLD (PLD injection only, n = 6), PLD + MBs + US (PLD injection, followed by MBs injection and then US sonication, n = 6), US (US sonication only, n = 3), and MBs + US (MBs injection, followed by US sonication, n = 3). We injected 10 mg/kg of PLD into the tail veins of the tumor-bearing mice for both PLD and PLD + MBs + US groups, and the other two groups were treated with either MBs + US or US alone, respectively, on days 7, 14, and 21 after tumor inoculation.
Growth response for medium-stage tumors treated with 6 mg/kg of PLD
To study the effect of US sonication with MBs on the therapeutic response of medium-stage tumors, we used an FUS transducer and an initial size of treated tumor of about 50 mm3 (10 days after inoculation). The mice were divided into five groups: the control (n = 6), PLD (n = 5), the PLD + MBs + US (n = 5), US (n = 3), and MBs + US (n = 3). Treatments were executed with an injection of 6 mg/kg PLD for both the PLD and PLD + MBs + US groups, and the other two groups were treated with either MBs + US or US alone, respectively, on days 10 and 17 after tumor inoculation.
Growth response for medium-stage tumors treated with 4 mg/kg of PLD
To further investigate the effect of MBs + US on the therapeutic response, a lower dose (4 mg/kg) of PLD was used to treat tumors with an initial size of about 50 mm3 for both the PLD and PLD + MBs + US groups. The treatments were executed on days 10 and 17 after tumor inoculation.
Measurements of tumor blood perfusion with a Laser Doppler
Red blood cell perfusion was measured using a laser Doppler (OxyLab LDF; Oxford Optronics, Oxford, UK). The mice bearing ear tumors were divided into three groups: the control group (n = 3), the PLD group (n = 3), and the PLD + MBs + US group (n = 5). Blood perfusion of the ear tumors was assessed just before both the first treatment (on day 10 after tumor inoculation) and the second treatment (on day 17). For each tumor, red blood cell perfusion was obtained from one central and two peripheral locations of the tumor using the Miniature Surface Probe (MSP310XP [diameter 1 mm]; AD Instruments, Bella Vista, NSW, Australia) with a self-adhesive disc. Estimates of perfusion were obtained at a frequency of 5 Hz over a period of 60 seconds. The measurements for each sample were repeated three times, and the mean value in blood perfusion units was calculated. The data were expressed as relative blood perfusion units with percentage values.
Microscopic system and histological morphology
Tumor development on both ears was observed every day after tumor inoculation, and the mice were anesthetized and photomicrographed every other day for both ears under transillumination using a Leica MZ16FA stereomicroscope (Leica Microsystems, Wetzlar, Germany).
The mice were killed at the end of the experiment, and were then infused with 0.9% saline via the left ventricle. After infusion, tumor tissues were harvested from both ears, and the tissues were immediately frozen in liquid nitrogen and kept at −80°C for later histological staining.
Mouse tumor tissues were moved from the −80°C freezer to a −20°C Leica CM3050 S Cryostat (Meyer, TX) and sectioned. The tissues were embedded into a Tissue-Tek OCT compound (Sakura Finetek, Torrance, CA) and were sectioned into 5-μm-thick slices on SuperFrost glass slides (Thermo Fisher Scientific, Braunschweig, Germany). Tissue slices were stained with hematoxylin and eosin (H&E) for histological analysis and evaluated using a Leica DMIRB microscope (Leica Microsystems) with image acquisition software to examine the slices of H&E staining.
Statistical analysis
Statistical analysis of variance was used to evaluate the data, and a nonparametric Mann–Whitney U test was used to determine the level of significance of differences in sample means with the Statistical Package for the Social Sciences version 16.0 software (SPSS, Chicago, IL). Values of P < 0.05 and P < 0.01 were considered significant.
Results
Influence of US sonication with MBs on tumor growth for early stage tumors treated with anticancer nanodrug
To investigate the therapeutic effects of US sonication with MBs on early stage tumors treated with anticancer nanodrug, we injected 10 mg/kg of PLD through the tail vein of the mice with an initial size of treated ear tumor of about 15 mm3 for both PLD and PLD + MBs + US groups, whereas the other two groups received US sonication with or without MBs injection. shows the tumor growth response during a sequence of treatments on days 7, 14, and 21 after tumor inoculation. The results show that the tumor growth is significantly inhibited in the PLD, PLD + MBs + US, and MBs + US groups, as compared with the control group (P < 0.05, P < 0.01). The results display that US sonication with MBs after an injection of 10 mg/kg PLD can further and earlier inhibit the tumor growth, as compared with PLD alone. It is also worth noting that tumor growth appears biphasic: the size increases after the first treatment and then decreases, for both PLD and PLD + MBs + US groups. Meanwhile, tumor growth for the MBs + US group was hindered, even though there was no PLD injection.
Figure 2 Effects of ultrasound (US) sonication with microbubbles (MBs) on tumor growth for early stage tumors treated with 10 mg/kg of anticancer nanodrug. The arrows indicate the schedule for treatments. The figure shows the tumor growth responses for an initial size of treated tumors of about 15 mm3 with different conditions: control, PLD alone, PLD + MBs + US, US alone, and MBs + US.
Notes: *P < 0.05; **P < 0.01 (Mann–Whitney U test); for each group, mean ± standard deviation.
Abbreviations: US, ultrasound; MBs, microbubbles; PLD, pegylated liposomal doxorubicin.
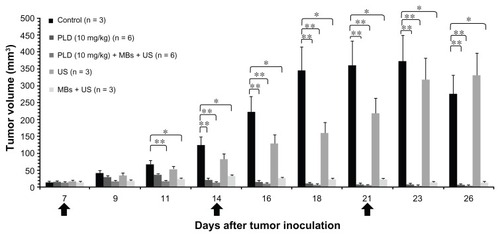
Influences of FUS sonication with MBs on tumor growth and blood perfusion for medium-stage tumors treated with anticancer nanodrug
To investigate the therapeutic effects of FUS sonication with MBs on medium-stage tumors treated with anticancer nanodrug, we injected 6 mg/kg of PLD into the mice with an initial size of treated ear tumor of about 50 mm3 for both PLD and PLD + MBs + US groups, whereas the other two groups received FUS sonication with or without MBs injection. shows the tumor growth responses for treatments on days 10 and 17 after tumor inoculation. This figure displays that the tumor growth was effectively inhibited after the first treatment for the PLD group (P < 0.05, as compared with the control group), and the tumor growth was further inhibited by FUS sonication with MBs (P < 0.01 between the PLD + MBs + US and control groups). Meanwhile, there was no significant difference among the control, US, and MBs + US groups.
Figure 3 Effects of focused US sonication with MBs on tumor growth for medium-stage tumors treated with 6 mg/kg. (A) Tumor growth responses for an initial size of treated tumors of about 50 mm3 with different conditions: control, PLD alone, PLD + MBs + US, US alone, and MBs + US. The arrows indicate the schedule for treatments. (B) Photomicrographs of mouse-ear tumors (control, PLD alone, and PLD + MBs + US) on days 10, 16, and 26 after tumor inoculation (magnification: 10×). (C) Blood perfusion measurements of tumors before treatment on days 10 and 17, for the control, PLD, and PLD + MBs + US groups.
Notes: Mean ± SD; *P < 0.05, **P < 0.01 (Mann–Whitney U test).
Abbreviations: US, ultrasound; MBs, microbubbles; PLD, pegylated liposomal doxorubicin.
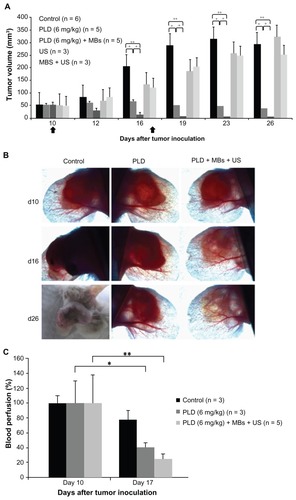
Photomicrograph was used to examine the tumor growth for the control, PLD, and PLD + MBs + US groups on days 10, 16, and 26 after tumor inoculation. , photomicrograph observation for the tumors, shows that the inhibition of tumor growth was more effective in the PLD + MBs + US group than the PLD group, and the tumor almost disappeared for the former.
Laser Doppler was used to examine the response of microvascular blood perfusion for the tumors after treatment. We measured the tumor blood perfusion for the control, PLD, and PLD + MBs + US groups just before both the first treatment (day 10 after tumor inoculation) and the second treatment (day 17 after inoculation). shows that blood perfusion significantly decreased 7 days after the first treatment for the PLD group (P < 0.05), and it was further decreased by FUS sonication with MBs (the PLD + MBs + US group) (P < 0.01).
Histological examinations for the influence of FUS sonication with MBs on tumor tissues
Staining with H&E was used to examine the change of histology induced in tumor tissues for the PLD, PLD + MBs + US, and MBs + US groups. The H&E staining of tumor tissues for these three groups is shown in , depicting the treatment results of . Hemorrhagic damage occurred rarely in the PLD and MBs + US groups, whereas for the PLD + MBs + US group, FUS sonication with MBs resulted in local suppression of neutrophil influx, interstitial edema, congestion, and disruption of tissue architecture.
Figure 4 H&E staining of tissue sections for the ear tumors after a sequence of treatments with MBs + US, PLD alone, and PLD + MBs + US.
Notes: Magnifications 20×, 100×, 200×, and 400× (yellow square).
Abbreviations: H&E, hematoxylin and eosin; MBs, microbubbles; US, ultrasound; PLD, pegylated liposomal doxorubicin.
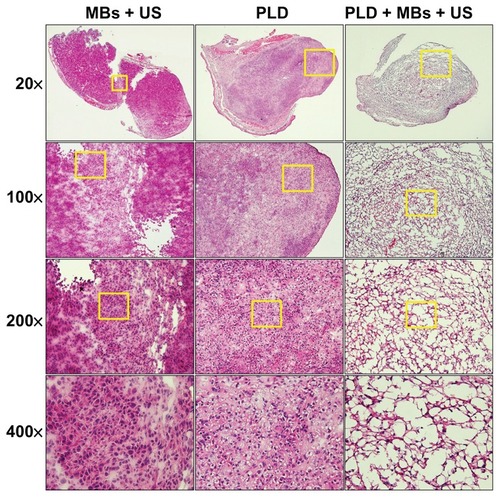
Influence of FUS sonication with MBs on tumor growth for medium-stage tumors with a low dose of anticancer nanodrug
To further study the effect of FUS sonication with MBs on the therapeutic response of anticancer nanodrug for medium-stage tumors, we injected a low dose (4 mg/kg) of PLD into the mice with an initial treated tumor of size about 50 mm3 for both PLD and PLD + MBs + US groups. shows the tumor growth response with treatments on days 10 and 17 after inoculation. The results show that the tumor growth was significantly different between the PLD and PLD + MBs + US groups. There was no hindrance to the tumor growth for the PLD group, while the application of FUS sonication with MBs after the injection of PLD was able to significantly inhibit tumor growth.
Figure 5 Tumor growth response of medium-staged tumors treated with 4 mg/kg of PLD with and without MBs + FUS. The arrows indicate the schedule for treatments.
Notes: Mean ± SD; *P < 0.05 (Mann–Whitney U test).
Abbreviations: PLD, pegylated liposomal doxorubicin; MBs, microbubbles; FUS, focused ultrasound.
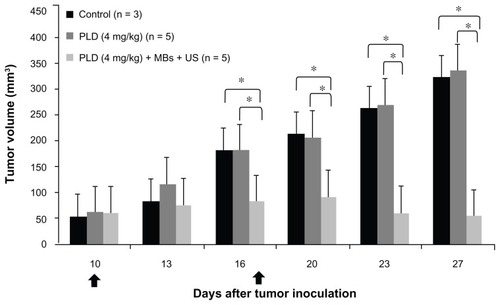
Discussion
In this study, we investigated the synergistic effect of US sonication with MBs on early and medium staged tumors located on mouse ears treated with anticancer nanodrug. The results showed that: (1) US sonication in the presence of MBs was always able to produce a further hindrance to tumor growth for both early and medium-stage tumors; (2) the dosage of anticancer nanodrug was one of the critical factors for the tumor growth inhibition: administration of 6 mg/kg PLD alone was able to inhibit the growth of medium-stage tumors, while this did not occur for injection of 4 mg/kg PLD alone; (3) with the application of MBs + US after nanodrug administration, a low dose of PLD (4 mg/ kg) was able to inhibit the growth of medium-stage tumors; (4) for early stage tumors after the first treatment with a high dose of nanodrug alone (PLD 10 mg/kg), the tumor size still increased for several days and then decreased (biphasic pattern); (5) the use of MBs + US alone was able to hinder the tumor growth of early stage tumors, but for medium-stage tumors MBs + US alone could not inhibit tumor growth, and an injection of PLD was required for effective hindrance. The experimental results indicated that the use of MBs + US can significantly affect the growth response of early stage tumors () and the application of MBs + US after PLD administration can enhance the anticancer treatment of PLD to further inhibit tumor growth for both early and medium-stage tumors (, , and ).
When US sonicates in the tumors right after an injection of MBs, the interaction of the US beam with MBs in blood vessels results in the bubbles’ oscillation, collapse, and even violent cavitation. These phenomena of MBs may disrupt blood vessel walls and produce vascular pores or even vascular rupture, which may be able to hinder the growth of early stage tumors () and improve the transport of nanodrug into tumor tissues to further inhibit tumor growth when PLD is injected before the use of US + MBs for both early and medium-stage tumors (, , and ). Meanwhile, the temperature rise in the sonicated tumor tissue during US sonication in the presence of MBs may have some thermal effects on the tumor to increase the blood flow for raising the delivery of nanodrug into tumor tissues.
The vascular transport of anticancer nanodrug into tumor tissues is related to both the stage of tumor growthCitation9,Citation25 and the use of MBs + US. For early stage tumors, the response of tumor growth displays a biphasic pattern (tumor size increases for several days and then decreases) after the first treatment with a high dose of PLD (10 mg/kg) alone. For early stage tumors, their vascular integrity is still rather intact for PLD and hence it impedes the transport of nanodrug into the tumor tissue,Citation21,Citation25 while the vascular permeability for PLD increases as the tumor enters the medium stage. This may be able to explain the phenomenon that early stage tumors still grew for several days after injection of a high dose of PLD (10 mg/kg), while medium-stage tumors were inhibited after a 6 mg/kg dose of PLD. It is also worth noting that the growth of early stage tumors after the first treatment was significantly hindered for the MBs + US group (). This indicates that the bubbles’ oscillation, collapse, and violent cavitation in microvessels during US sonication disrupt microvessels sufficiently to affect the growth of early stage tumors. Previous studies displayed that early stage tumors have a much higher microvascular density,Citation9,Citation25 and a prominent antivascular effect on tumors was produced after US sonication with MBs.Citation26,Citation27 Histology demonstrated disruption of vascular walls and tumor cell death in areas of vascular congestion and thrombosis.Citation28 For early stage tumors, their microvasculature is denser but fragile, and hence more thorough damage may appear to suppress tumor growth after US sonication with MBs. The damage level of blood vessels to hinder tumor growth due to US sonication depends on tumor growth stage, quantity of injected MBs, and sonication conditions, which may be able to explain the hindrance difference between early and medium-stage tumors for the MBs + US groups in and .
The transport of nanodrug and its concentration distribution in tumor tissues are related to (1) convection, in which fluid flows through the vascular walls into tumor tissues and from tumor tissues to the adjacent normal tissues, (2) diffusion, which is determined by concentration difference of nanodrug between intravascular and extravascular regions, and (3) injected dosage of nanodrug. Hence, vascular density, vascular permeability, and interstitial diffusivity of PLD nanodrug, interstitial pressure in tumors, and injected dosage are crucial factors affecting the anticancer treatment. Early stage tumors possess high vascular density, but with relatively intact vascular walls hampering the transport of PLD into tumor tissues. Hence the use of MBs + US to disrupt vascular integrity can effectively induce the transport of nanodrug into interstitial tumor tissues to hinder tumor growth. This may be able to explain the reason for the hindrance to tumor growth for the PLD + MBs + US group (P < 0.01 compared with the control group) appearing significantly earlier than the PLD group, as shown in . For medium-stage tumors (about 50 mm3), they have already developed pores on vascular walls sufficiently large for the transport of PLD nanodrug to the extravascular region, while their vascular density is relatively less. It means that there is a longer distance for drug to travel to reach a therapeutic level in some tumor region. An administration of 6 mg/kg PLD is able to inhibit tumor growth, while an injection of 4 mg/kg PLD cannot result in a sufficiently high dose of drug in some tumor regions, due to the long-distance transport of drug, to hinder medium-stage tumor growth. FUS sonication with MBs is able to disrupt blood vessels to enable greater transport of PLD (), making it possible to inhibit tumor growth with a dose as low as 4 mg/kg (). This suggests that the use of MBs + US may reduce the dosage of PLD down to 4 mg/kg, which may avoid toxicities while maintaining antitumor efficacy. In addition to vascular density and permeability, other tumor microenvironment conditions also need to be considered, such as high interstitial fluid pressure, hypoxia, and low pH, which may hinder drug delivery and treatment effectiveness for a low dose of PLD to inhibit the growth of medium-stage tumors.
Tumor perfusion measured by laser Doppler showed that the reduction of blood perfusion was statistically significant for both the PLD and PLD + MBs + US groups (P < 0.05, P < 0.01) after one treatment, as shown in . As compared with , the tumor growth was significantly inhibited for the PLD group, and the tumors even shrank significantly for the PLD + MBs + US group. This suggests that vasculature was effectively damaged by PLD treatment and further damaged with the application of MBs + US, while the reduction of blood perfusion in the control group can be regarded as due to the significant growth of tumor size. During US sonication in the presence of MBs, the oscillation, collapse, and cavitation of MBs in the acoustic beam produced vascular pores and disruption of vessel wall to increase vascular permeability significantly in the sonicated areas. This may have further led to the formation of smaller bubbles, which interacted with the US beam and caused the cellular bioeffects. The predominant acute effects of US sonication might have induced dilation of tumor vessels and hemorrhage, so that there would not have been the normal structural support for the capillaries. As the vessel wall was no longer functioning well, it was incapable of counteracting the intravascular pressure, and then the capillaries dilated and became leaky, even cascading to edema. shows the histological results, in which the peritumor edema was a characteristic finding in the tumor morphology. This implies that the mild intercellular fluid across the entire tumor with nanodrug solution might further induce tumor damage for the PLD + MBs + US group, while MBs + US alone enhanced the flow perfusion without cellular damage. Therefore, nanodrug delivery enhancement with MBs + US was correlated with structural changes created in the tissue.
In this study, a significant hindrance to tumor growth was achieved using MBs + US to disrupt tumor blood vessels, while targeted delivery of nanodrug into tumor tissue was enhanced. However, the associated toxicities may appear in other tissue when the high-pressure region of the US beam is scanned through those tissues, and it is likely to cause some vascular damage and chemotherapeutic effects on sonicated nontumor tissue. Furthermore, the disruption of blood vessels in the sonicated tumor can enhance the delivery of nanodrug into tumor tissues, while it may have a double-edged-sword effect in facilitating the intravasation of tumor cells into circulation to increase metastasis. In future studies, it will be valuable to investigate the possibility of metastasis increase for the PLD + MBs + US group.
Acknowledgments
This work was supported by the National Health Research Institutes (No NHRI: ME-100-PP-13) and the National Science Council of Taiwan (No NSC: 99-2221-E-002-005-MY3).
Disclosure
The authors report no conflicts of interest in this work.
References
- YouSZuoLLiWOptimizing the time of Doxil injection to increase the drug retention in transplanted murine mammary tumorsInt J Nanomedicine2010522122920463938
- MatsumuraYMaedaHA new concept for macromolecular therapeutics in cancer chemotherapy: mechanism of tumoritropic accumulation of proteins and the antitumor agent smancsCancer Res198646638796322946403
- FerrariMCancer nanotechnology: opportunity and challengesNat Rev Cancer2005516117115738981
- MatsumuraYPoly(amino acid) micelle nanocarriers in preclinical and clinical studiesAdv Drug Deliv Rev20086089991418406004
- SengerDRGalliSJDvorakAMPerruzziCAHarveyVSDvorakHFTumor cells secrete a vascular permeability factor that promotes accumulation of ascites fluidScience19832199839856823562
- DvorakHFSioussatTMBrownLFDistribution of vascular permeability factor (vascular endothelial growth factor) in tumors: concentration in tumor blood vesselsJ Exp Med1991174127512781940805
- FerraraNGerberHPLeCouterJThe biology of VEGF and its receptorsNat Med2003966967612778165
- JainRKBarriers to drug delivery in solid tumorsSci Am199427158658066425
- YamauraHSatoHQuantitative studies on the developing vascular system of rat hepatomaJ Natl Cancer Inst197453122912404372377
- SilvaACOliveiraTRMamaniJBApplication of hyperthermia induced by superparamagnetic iron oxide nanoparticles in glioma treatmentInt J Nanomedicine2011659160321674016
- MaedaHTumor-selective delivery of macromolecular drugs via the EPR effect: background and future prospectsBioconjugate Chem201021797802
- HancockHASmithLHCuestaJInvestigations into pulsed high-intensity focused ultrasound-enhanced delivery: preliminary evidence for a novel mechanismUltrasound Med Biol2009351722173619616368
- BohmerMRChlonCHTRajuBIFocused ultrasound and microbubbles for enhanced extravasationJ Control Release2010148182420600402
- ZhaoYZLuCTZhouZCEnhancing chemotherapeutic drug inhibition on tumor growth by ultrasound: an in vivo experimentJ Drug Target20111915416020429773
- LinCYHuangYLLiJRChangFHLinWLEffects of focused ultrasound and microbubbles on the vascular permeability of nanoparticles delivered into mouse tumorsUltrasound Med Biol2010361460146920800173
- LinCYLiuTMChenCYQuantitative and qualitative investigation into the impact of focused ultrasound with microbubbles on the triggered release of nanoparticles from vasculature in mouse tumorsJ Control Release201014629129820621645
- RapoportNKennedyAMSheaJEControlled and targeted tumor chemotherapy by ultrasound-activated nanoemulsions/microbubblesJ Control Release200913826827619477208
- CoussiosCCFarnyCHHaarGTRoyRARole of acoustic cavitation in the delivery and monitoring of cancer treatment by high-intensity focused ultrasound (HIFU)Int J Hyperthermia20072310512017578336
- PriceRChappellJSongJKlibanovANanoparticle delivery into biological tissues by ultrasonic microbubble destructionNanomedicine20062269312
- FrenkelVEtheringtonAGreeneMDelivery of liposomal doxorubicin (Doxil) in a breast cancer tumor model: investigation of potential enhancement by pulsed-high intensity focused ultrasound exposureAcad Radiol2006446947916554227
- HoriKNishiharaMShiraishiKYokoyamaMThe combretastatin derivative (Cderiv), a vascular disrupting agent, enables polymeric nanomedicelles to accumulate in microtumorsJ Pharm Sci2010992914292520039393
- HuwylerJDreweJKrahenbuhlSTumor targeting using liposomal antineoplastic drugsInt J Nanomedicine20083212918488413
- GabizonAShiotaRPapahadjopuulosDPharmacokinetics and tissue distribution of doxorubicin encapsulated in stable liposomes with long circulation timesJ Natl Cancer Inst198981148414882778836
- Al-JamalWTAl-JamalKTTianBLipid-quantum dot bilayer vesicles enhance tumor cell uptake and retention in vitro and in vivoACS Nano2008240841819206564
- HoriKNishiharaMYokoyamaMVital microscopic analysis of polymeric micelle extravasation from tumor vessels: macromolecular delivery according to tumor vascular growth stageJ Pharm Sci20109954956219544373
- BunteRMAnsaloniSSehgalCMLeeWMWoodAKHistopathological observations of the antivascular effects of physiotherapy ultrasound on a murine neoplasmUltrasound Med Biol20063245346116530105
- WoodAKBunteRMCohenJDThe antivascular action of physiotherapy ultrasound on a murine tumor: role of a microbubble contrast agentUltrasound Med Biol2007331901191017720299
- WoodAKAnsaloniSZiemerLSLeeWMFeldmanMDSehgalCMThe antivascular action of physiotherapy ultrasound on murine tumorsUltrasound Med Biol2005311403141016223644