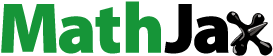
Abstract
Objective
To investigate cross-linked hydrogels prepared via inverse emulsion polymerization to entrap poorly aqueous soluble drugs. Polyethylene glycol cross-linked acrylic polymers were synthesized and the loading and release of curcumin, a model hydrophobic drug, was investigated.
Methods
Physicochemical characteristics of hydrogels were studied with 13C nuclear magnetic resonance, Fourier transform infrared spectroscopy, transmission electron microscopy, scanning electron microscopy, differential scanning calorimetry, and swelling. Polymerization of the acrylic acid with cross-linked polyethylene glycol diacrylate was characterized with 13C nuclear magnetic resonance imaging and Fourier transform infrared spectroscopy.
Results
The in vitro release rate of curcumin showed that there was a sustained release from the hydrogel with increased cross-linking; the release rate depended on the pH of the releasing medium. Intracellular and cytotoxicity studies were carried out in human cervical cancer cell lines.
Conclusion
The results suggest cross-linked acrylic polymers can be used as efficient vectors for pH-sensitive, controlled delivery of hydrophobic drugs.
Introduction
Hydrogels are three-dimensional polymer networks that exhibit a semisolid morphology and can absorb a large amount of water. The water content of hydrogels occurs due to the presence of ionizable functional groups, which affects characteristics such as permeability, mechanical stability, and biocompatibility.Citation1 Hydrogels’ highly porous structure can easily be tuned by controlling the density of cross-links in the gel matrix and the affinity of the hydrogels for the aqueous environment in which they are swollen. Their porosity also permits loading of drugs into the gel matrix and subsequent drug release at a rate dependent on the diffusion coefficient of the small molecule or macromolecule through the gel network. Hydrogels are extremely useful for application in various fields such as drug delivery and tissue engineering due to biocompatibility, stimuli responsive behavior, and susceptibility for chemical modification.Citation2–Citation4 Biodegradable polymer systems for controlled-release drug delivery have been extensively studied because an invasive technique such as surgery is not required after their use.Citation5–Citation7 Especially, biodegradable hydrogels have been represented as an attractive drug formulation because of their advantages such as biocompatibility,Citation8 high responsibility for specific degradation, and a feasible approach for incorporating drugs in matrices.
Hydrogel-based devices belong to the group of swelling-controlled drug delivery systems.Citation9 When the polymer network comes in contact with aqueous solutions, the thermodynamic compatibility of the polymer chains and water causes the polymer to swell.Citation10 As water penetrates inside the glassy network, the glass temperature of the polymer decreases, and the hydrogel becomes rubbery. In the case of nonswelling-controlled delivery systems, the relaxation rate of the polymer is very slow compared with the water transport inside the hydrogel. Hydrogels have been extensively exploited for biomedical applications due to their high water content, which imparts excellent biocompatibility.Citation11 The high water content and large pore sizes of most hydrogels often result in relatively rapid drug release, over a few hours or days. Hydrogels have been reported as novel vectors for controlled drug-release systems since they exhibit dramatic changes in their swelling behavior,Citation12 network structure, permeability, or mechanical strength in response to different stimuli. Hydrogels can be prepared from natural and synthetic polymers. Those prepared from natural polymers possess inherent bioactive properties whereas those prepared from synthetic polymers have well-defined structures that can be modified to yield biocompatibility and functionality that can be tailored.Citation13 Moreover, natural polymers may not provide sufficient mechanical properties.Citation14 Polyacrylic acid-based polymers are ideal candidates for drug delivery due to their swelling behavior in aqueous environments.Citation15 These polymers are novel for pH-sensitive drug delivery, which shows swelling at a higher pH due to the ionizable carboxyl groups, and can release drugs at a neutral pH. One of the major disadvantages reported for a polyacrylic acid-based drug delivery system is rapid drug release, which can be controlled with cross-linking. Highly cross-linked systems provide slow drug release due to the small mesh size, which can be advantageous in drug delivery applications. The polymer also determines the physical properties of the hydrogel, including mechanical strength, degradability, and diffusivity of the releasing molecule. Dissolution of the polymer in an aqueous environment can be prevented with cross-linking.Citation16
Curcumin, a naturally occurring yellow polyphenol from the rhizome of the perennial herb Curcuma longa, has potent anticancer properties.Citation17 Curcumin inhibits proliferation as well as induces apoptotic cell death in numerous cell lines established from malignancies such as leukemia, breast, lung, prostate, and colon tumors.Citation18–Citation20 Studies in numerous carcinogenesis modelsCitation21–Citation25 and recent clinical trials in patients have confirmed the potential of curcumin as a tool for chemoprevention. However, clinical application is limited due to poor aqueous solubility, minimal systemic bioavailability, degradation at alkaline pH, and photodegradation. The therapeutic index can be improved by entrapping curcumin in a biocompatible polymer, which can enhance solubility in an aqueous solution and extend the release.
However, hydrogel systems for hydrophobic drug delivery are rarely reported due to the incompatibility of the hydrophilicity of the polymer network and the hydrophobic drug. This problem can be solved by preparing semi-interpenetrating networks to tune the hydrophilicity to entrap hydrophobic drugs. In the current investigation, we developed a pH-sensitive, biodegradable, and biocompatible hydrogel to achieve site-specific drug delivery. We synthesized polyethylene glycol (PEG) cross-linked acrylic polymers and investigated the loading and release of curcumin. The method adopted for polymerizing the acrylic acid was the inverse emulsion polymerization method proposed by Vanderhoff et al in which an aqueous solution of a hydrophilic monomer, acrylic acid, is dispersed in a continuous lipophilic phase with the aid of surfactants to promote the formation of water in oil emulsion.Citation26 Cell uptake and cytotoxicity studies were carried out in human cervical cancer cell lines (HeLa).
Methods and materials
Materials
Acrylic acid (molecular weight = 72), cross-linked PEG diacrylate (molecular weight = 238), curcumin, ammonium persulfate, Span 80 (Sorbitan monooleate), Tween 80 (PEG sorbitan monooleate), and 3-(4,5-dimethylthiazol-2-yl)-2,5-diphenyltetrazolium bromide (MTT) were purchased from Sigma-Aldrich (Steinheim, Germany). Immobilon Western reagent was purchased from Millipore (Billerica, MA). Rabbit polyclonal antibody against poly (ADP-ribose) polymerase (PARP) was obtained from Santa Cruz Biotechnology (Santa Cruz, CA). Rabbit monoclonal antibody against caspase-3 and rabbit polyclonal antibody against caspase-9 were obtained from Cell Signaling (Beverly, MA). All other reagents and chemicals were of analytical grade or above, and used without further purification.
Nanosphere preparation
Nanoparticles were prepared with the inverse emulsion polymerization method. Emulsification was performed by dispersing the aqueous phase consisting of 10% acrylic acid, 5% sodium hydroxide, and 15% water with the continuous lipophilic phase consisting of liquid paraffin (68%) and emulsifiers (2%) and Span 80 and Tween 80 (75:25 ratio). One percent PEG diacrylate was added to the mixture as a cross-linker, and then the initiator, ammonium persulfate, was added. The temperature for polymerization was maintained at 60° C for 6 hours. The particles were isolated with centrifugation (10,000 rpm) for 30 minutes. The isolated nanospheres were washed several times with hexane and then freeze-dried to remove the solvent and water. The freeze-dried products were stored in a vacuum. The polymerization of the acrylic acid with cross-linked PEG diacrylate was characterized with 13C nuclear magnetic resonance spectroscopy (NMR) and Fourier transform infrared (FTIR) spectroscopy.
Swelling studies
The gravimetric method was used to study the swelling behavior of the cross-linked polymers as a function of pH. A known quantity of the polymers was measured and immersed in an excess quantity of phosphate buffers with pH 2.2 and in phosphate buffered saline with pH 7.4 for 24 hours to attain the swelling equilibrium. The weight of the swollen polymers was measured after excess solvent was removed. The swelling behavior was computed by calculating the percentage swelling (%S):
Drug loading
Curcumin was loaded in polymer nanoparticles using the postpolymerization method. About 100 mg of the lyophilized powder was dispersed in 10 mL of distilled water. Curcumin was dissolved in chloroform, and the drug solution in chloroform was added to the polymer solution with constant vortexing and sonication. The curcumin-loaded nanoparticles were then lyophilized to obtain dried powder.
Entrapment efficiency
A known amount of the lyophilized curcumin-loaded polymers was dissolved in methanol, vigorously vortexed to get a clear solution, and maintained for 24 hours, then filtered through a 0.1 μM membrane filter. Absorbance of the filtrate was measured at 420 nm using LAMBDA 25 ultraviolet absorbance (PerkinElmer, Waltham, MA). The entrapped curcumin content was determined with the actual entrapment ratio (AER), expressed in terms of the amount of curcumin per the weight of the nanospheres.Citation27 Entrapment efficiency can be calculated with the following equation:
Transmission electron microscopy
Morphological analysis of the nanospheres was characterized with transmission electron microscopy (TEM; JEOL 1011, Tokyo, Japan). The samples of the nanoparticle suspension in Milli-Q® (Millipore Corporation, Billerica, MA) water at 25° C were dropped onto Formvar-coated grids, and measurements were taken only after the samples had completely dried.
Differential scanning calorimetry
Differential scanning calorimetry (DSC) was performed to analyze the state of the curcumin inside the polymer matrix. DSC thermograms of free curcumin and curcumin-loaded hydrogel were compared to analyze the physicochemical behavior of the nanoparticles. The DSC thermograms were obtained using an automatic thermal analyzer system (Pyris 6 DSC, PerkinElmer). Samples were crimped in standard aluminium pans and heated from 20°C to 250°C at a rate of 10°C/minute under constant purging of N2 at 10 mL/minute. An empty pan, sealed in the same way as the sample, was used as a reference.
In vitro release kinetics
A known amount of curcumin-loaded polyacrylic acid was dispersed in 10 mL phosphate buffer (pH 7.4) and was left in a shaking incubator at 37°C ± 0.5°C. At predetermined time intervals, a known quantity of the sample was withdrawn and replaced with fresh medium to maintain the total volume constant. The amount of curcumin released from the cross-linked hydrogel nanoparticles was measured with an ultraviolet spectrophotometer (PerkinElmer) at 420 nm.
Cell uptake studies
Internalization of the curcumin-loaded hydrogel was studied in HeLa cells using confocal laser scanning microscopy that exploits the inherent fluorescence of curcumin (Leica TCS SP2, Leica Microsystems GmbH, Wetzlar, Germany). HeLa cells were grown in cover slips placed in 24 well plates, up to 80% confluency. Cells were then treated with 25 μM free curcumin, curcumin-loaded hydrogels, and plain hydrogels. After 2 hours, the cells were washed, and the nuclei were stained with propidium iodide and examined under a confocal laser-scanning microscope for the intracellular fluorescence of curcumin.
MTT assay
Cytotoxicity studies of free curcumin and curcumin nanogels were carried out in HeLa cells using MTT dye assay.Citation28 HeLa cells were seeded (3.0 × 103/well) in a 96-well culture plate and grown for 24 hours before the assay. The cells were then treated with different concentrations of free and curcumin nanogels (5–50 μM) for 72 hours and then 20 μL MTT (5 mg/mL) was added in 80 μL culture medium (Dulbecco’s modified Eagle’s medium purchased from Invitrogen, Grand Island, NY, USA) to each well. After incubating for 2 hours at 37° C, cells were lysed using lysis buffer, incubated for 1 hour, and the optical densities were measured at 570 nm using a microplate reader (Bio-Rad Laboratories, Hercules, CA). The relative cell viability in percentage was calculated as:
Western blot analysis
To detect the cleavage of caspases and PARP by curcumin/curcumin nanogels, Western blot was performed as previously described.Citation28 Briefly, 0.6 × 106 cells were plated in 60 mm plates and treated with curcumin for 24 hours. The total protein isolated was resolved in a sodium dodecyl sulfate–polyacrylamide gel electrophoresis, immunoblotted using anticaspase-3, anticaspase-9, and anti-PARP antibody, and detected with enhanced chemiluminescence.
Clonogenic assay
The cells were seeded in six-well plates and treated with curcumin/curcumin nanogels for 72 hours after which the clonogenic assay was performed as previously described.Citation29 Then 104 cells in six-well plates were treated with curcumin for 72 hours. Following this, fresh medium was added and incubated for 1 week. The clones developed were fixed in glutaraldehyde and stained using crystal violet. A colony containing more than four cells was counted as one clone. The clones were counted and compared with those of the untreated control.
Fluorescence activated cell sorting
The cells were subjected to curcumin/curcumin nanogel (25 μM) treatment for 24 hours and analyzed with fluorescence activated cell sorting (FACS) as described previously.Citation30 Briefly, the cells were trypsinized, and the cell pellets were resuspended in 70% cold ethanol and incubated for 30 minutes at 4°C. The permeabilized cells were spun down and suspended in 250 μL of phosphate buffered saline. To this, 5 μL of RNase A (10 mg/mL) was added, incubated at 37°C for 30 minutes, stained with 10 μL of propidium iodide (1 mg/mL), and analyzed using the FACS Aria flow cytometer (BD Biosciences, Franklin Lakes, NJ).
Results
Characterization of nanogels
Curcumin nanogels in the cross-linked polymer combinations (0.5% and 1%) were successfully prepared using the inverse emulsion polymerization method (). The particles’ morphology and size were studied using TEM. The nano-particles obtained ranged from 50 to 100 nm with a narrow size distribution. Cross-linking was confirmed with FTIR spectroscopy () and 13C NMR ().
Figure 1 Synthesis of polyethylene glycol cross-linked acrylic polymers.
Abbreviation: APS, ammonium persulfate.
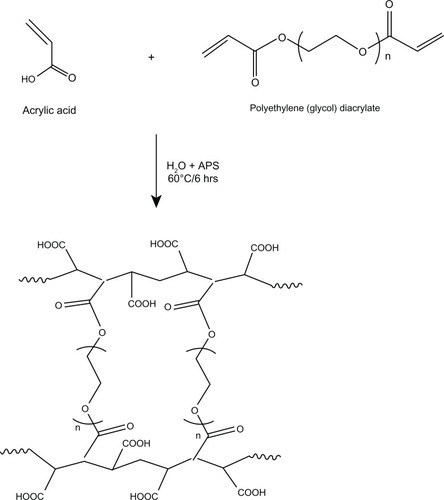
The FTIR spectrum shows an absorption band at 1721 cm−1 due to the C=O of PEG diacrylate and at 2926 cm−1 due to asymmetrical stretching of the methylene groups. The peaks at 3556 cm−1 and 1038 cm−1 were observed due to the –OH and –COC stretching frequencies. Furthermore, absorption peaks at 1560 cm−1 and 1404 cm−1 were assigned to asymmetric and symmetric stretching vibration of the carboxylate ion.
13C NMR showed a peak at around 39 ppm, which corresponds to the C–C bond, and around 180 ppm, which corresponds to the peak for the C=O bond. The peak at 61 ppm and the peak at 63 ppm are attributed to the chemical shift of the C–O and –OH bonds, respectively. The peak at 70 ppm shows the CH2 of PEG diacrylate.
The degree of cross-linking, variation in particle size, and encapsulation efficiency are summarized in . The entrapment efficiency of the 0.5% and 1% cross-linked polymers was 71.6% ± 1.6% and 67.5% ± 0.51%, respectively. Results showed the entrapment efficiency decreased as cross-linking increased, which might be due to differences in the swelling behavior.
Table 1 Degree of swelling, entrapment efficiency, and particle size of hydrogels
The influence of pH on the nanogels’ swelling capacity was also analyzed (). The effect of pH on the swelling of the polymers was studied with gravimetric analysis. Sample A (0.5%) showed swelling of 36.6 ± 0.82 g/g of nanogel at pH 7.4 and 16.4 ± 0.72 g/g of nanogel at pH 2.2 whereas B (1%) showed swelling of 21.2 ± 1.34 g/g of nanogel and 17.8 ± 1.22 g/g of nanogel at pH 7.4 and 2.2, respectively.
TEM images showed the mean diameter of the particles with 0.5% and 1% cross-linking was 50 and 100 nm, respectively (). Particle size increased when cross-linking increased.
In vitro drug release and cellular uptake
Free curcumin is poorly soluble in aqueous medium and forms flakes but readily soluble in dimethyl sulfoxide (DMSO). This makes it very difficult to administer curcumin for in vivo studies, as DMSO is a highly toxic chemical and cannot be used in vivo. The in vitro release profile of the entrapped anticancer drug curcumin from two different cross-linked polymer systems is shown in . In the case of the 0.5% cross-linked polymers, there was an initial burst release of 40% and complete release occurred in 4 hours due to the swelling of the nanogels at pH 7.4 and the increase in the mesh size, which might make it difficult to control the diffusion of curcumin. However, in the case of the 1% cross-linked polymers, there was an initial release of around 12% and thereafter the release occurred in a sustained manner, which might be due to the decrease in the swelling because of the increase in cross-linking. Curcumin exhibits green fluorescence after exhibiting excitation at 420 nm. We observed a strong fluorescence in cells treated with curcumin nanogels suspended in medium and curcumin dissolved in DMSO, indicating the cellular uptake of curcumin nanogels is equivalent to that of curcumin dissolved in DMSO ().
Significant cytotoxicity exhibited by the nanogel form of curcumin-encapsulated 1% cross-linked polymers
MTT assay was used to compare the cytotoxicity of the curcumin-encapsulated polymers suspended in aqueous medium with that of curcumin dissolved in DMSO. The cytotoxicity of curcumin in DMSO was compared with that of curcumin-encapsulated cross-linked polymers in HeLa cells (). The curcumin nanogels with 1% cross-linked polymers showed slightly more cytotoxicity compared with the 0.5% cross-linked polymers and curcumin in DMSO especially at the half-maximal inhibitory concentration (IC50) range while the blank polymers showed no significant toxicity.
Figure 7 Gel form of curcumin is as effective or slightly more effective in inducing cytotoxicity: (4,5-dimethylthiazol-2-yl)-2, 5-diphenyltetrazolium bromide assay was conducted in human cervical cancer cell lines at various concentrations and time intervals as indicated.
Abbreviations: HeLa, human cervical cancer cell lines; DMSO, dimethyl sulfoxide.
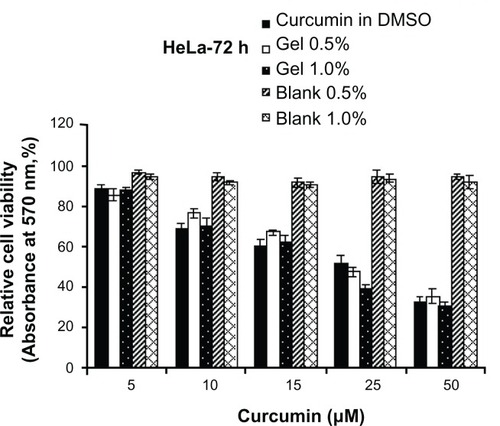
Better apoptosis of curcumin nanogels compared with curcumin in DMSO
The ability of curcumin nanogels to induce apoptosis in HeLa cells was evaluated by the extent of the cleavage of the initiator caspase-9, the effector caspase-3, and the DNA-repairing protein, PARP, the downstream substrate of caspase-3 and a hallmark of apoptosis,Citation31 and compared with that of free curcumin in DMSO, which has been reported by our group.Citation32 The 0.5% and 1% curcumin nanogels cleaved the caspases-3 and −9 more effectively than free curcumin in DMSO (). Similarly, when the cells were treated with 10 and 25 μM curcumin, the nanogel forms induced PARP cleavage, and the curcumin nanogels with 1% cross-link showed better efficacy compared with curcumin in DMSO (). In the cells treated with curcumin nanogels with 1% cross-link, the 116 kDa form was almost completely degraded to the cleaved 85 kDa form, which was further degraded into smaller fragments, indicating the extent of caspase-3 activation induced by these polymers was much higher than that of the 0.5% polymers and curcumin in DMSO.
Figure 8 Curcumin nanogels enhance poly(ADP-ribose) polymerase cleavage in human cervical cancer cell lines: Human cervical cancer cell lines cells were treated with the indicated concentrations of free curcumin/curcumin nanogels for 24 hours, and the entire cell lysate was immunoblotted against (A) anticaspase-3, (B) anticaspase-9, and (C) antipoly(ADP-ribose) polymerase antibodies.
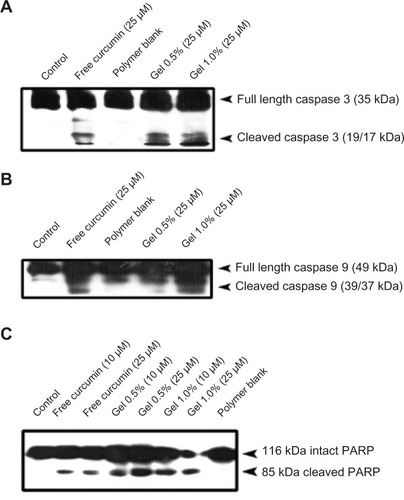
Downregulation of clonogenic potential of HeLa cells by curcumin nanogels compared with that of curcumin in DMSO
Using clonogenic assay, we investigated whether curcumin or curcumin nanogels with different percentages of cross-linking can downregulate the clonogenic potential of HeLa cells. The results clearly indicate the clonogenic potential of HeLa cells was significantly inhibited by the curcumin nanogels (P < 0.001) and by curcumin in DMSO (). The inhibition of clonogenic potential by curcumin nanogels was slightly higher compared with curcumin in DMSO.
Figure 9 Curcumin and curcumin nanogels inhibit the clonogenic potential of human cervical cancer cell lines: Human cervical cancer cell lines were treated with curcumin dissolved in dimethyl sulfoxide or curcumin nanogels for 72 hours, fresh medium was added and maintained for 1 week, and the clones developed were stained and counted.
Abbreviation: DMSO, dimethyl sulfoxide.
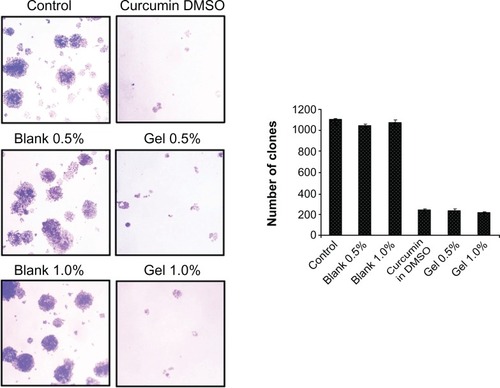
The nanogel forms of curcumin induce more G2/M arrest compared to curcumin in DMSO
Cell cycle arrest induced by curcumin in HeLa cells was also examined. The results clearly indicate that curcumin/curcumin nanogels induce G2/M arrest in HeLa cells. While curcumin in DMSO induced 28% G2/M arrest, the 0.5% and 1% curcumin nanogels induced 19.5% and 24.7% G2/M arrest, respectively. Although not much difference was observed in the cell cycle arrest between the various forms of curcumin, the 1% curcumin nanogels (1.0%) were slightly better than the 0.5% curcumin nanogels, as shown in .
Figure 10 Curcumin and nanogel curcumin induce G2/M arrest of human cervical cancer cell lines.
Notes: Nanogel 1.0% is slightly better than nanogel 0.5%. A24: Blank 0.5%; B24: Blank 1.0%; C24: Control; A25-24: Curcumin nanogel 0.5%; B25-24: Curcumin nanogel 1.0%; C25-24: Curcumin in DMSO. Curcumin concentration is 25 μM.
Abbreviation: DMSO, dimethyl sulfoxide.
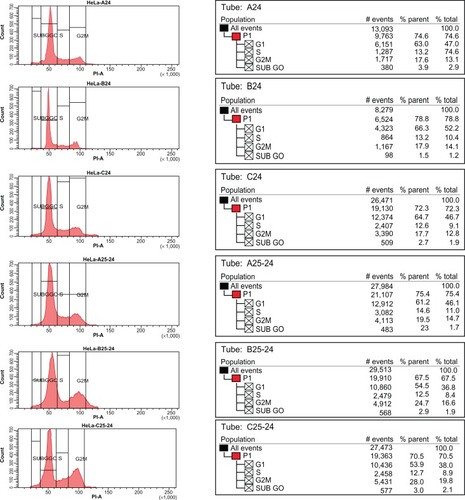
Discussion
Curcumin, a yellow pigment present in the spice turmeric (Curcuma longa), has been linked with antioxidant, antiinflammatory, antiproliferative, anticancer, antidiabetic, antirheumatic, and antiviral effects, but the phytochemical’s optimum potential is limited by a lack of solubility in aqueous solvents and poor oral bioavailability, which limit its use as a potential chemotherapeutic drug.Citation33 Nanoparticle-mediated drug delivery sytems have proved excellent solutions to these problems.Citation34
Inverse emulsion polymerization is a controllable method for preparing well-defined nanoparticles. In this study, we prepared acrylic polymers cross-linked with two different molecular weight percentages of PEG diacrylate using the inverse microemulsion polymerization method (the schematic representation is given in ). This method facilitates free radical polymerization of an acrylic monomer with PEG diacrylate in the presence of ammonium persulfate. PEG is a highly biocompatible and hydrophilic polymer with a low glass transition temperature. Including polyacrylic acid in the system imparts pH sensitivity. The nanogels we prepared also displayed a pH-sensitive nature, which can be exploited for site-specific controlled drug delivery.
Cross-linking was confirmed with FTIR spectroscopy () and 13C NMR (). The effect of size on the biological performance of nanoparticles is well documented; an optimal diameter range is believed to favor prolonged circulation times and passive targeting of carriers thanks to the enhanced permeation retention effect.Citation35 Below 200 nm and above 10–20 nm is the optimum size for the enhanced permeation retention effect.Citation36 TEM images showed that the mean diameter of the particles with 1% cross-linking was greater than that of the particles with 0.5% cross-linking, which shows nanogels with 1% cross-linked polymers have better encapsulation efficiency – one of the reasons for better anticancer activity. Particle size increased as cross-linking increased. DSC of the PEG diacrylate cross-linked acrylic polymers indicates an endothermic transition at 120° C that can be attributed to the loss of loose and bound water in the hydrogel. The nanogel appeared to be thermally stable up to 200° C. Curcumin was loaded onto the cross-linked acrylic polymers through physical adsorption using the postloading method. Cross-linking using PEG diacrylate provides hydrophobicity to the acrylic hydrogel, which enhances the uptake of curcumin, a hydrophobic drug. Results showed that entrapment efficiency decreased when cross-linking increased, which might be due to differences in the swelling behavior (). Swelling studies revealed that pH and cross-linking influence polymer swelling. Polymer swelling occurs at a pH above the pKa of the carboxyl group of acrylic acids. Swelling increases as the COO concentration increases, which can be reduced by enhancing the cross-linking density.
The in vitro release kinetic profile followed a biphasic pattern: an initial burst release followed by a sustained release (). The burst release of the curcumin from the polymers was controlled by increasing the cross-linking. Hydrogels with 1% cross-linked polymers showed an initial release of around 12%, and thereafter the release occurred in a sustained manner, which might be due to the decrease in the swelling because of the increase in cross-linking. Controlled delivery by cross-linked polymers is a desirable property for drug delivery applications.
To biologically evaluate curcumin nanogels, we compared the intracellular fluorescence of curcumin nanogels dispersed in aqueous medium with that of free curcumin in DMSO. The confocal images of the cell uptake studies show curcumin nanogels have almost similar cellular uptake, in vitro, as their free counterpart (). These data confirm curcumin nanogels overcome the barrier of curcumin’s aqueous dispersibility, which facilitates administration in vivo, by avoiding use of toxic solvents like DMSO.
We observed that curcumin nanogels show more cytotoxicity and apoptotic effects toward cancer cells than free curcumin, as assessed by cleavage of caspases and their downstream target, PARP, in HeLa cells. Previous studies indicate that nanoencapsulation of curcumin can downregulate the anchorage-independent proliferation of tumor cells.Citation37 We observed a marked difference in the number and size of the clones between curcumin nanogels and the untreated control, which is in concordance with the results of the cytotoxicity study. Inhibition of colony formation by curcumin nanogels is as effective as free curcumin, which supports the idea of using curcumin nanogels for sustained anticancer activity. We observed the curcumin-adsorbed acrylic nanogels are water soluble and as effective as or more active than free curcumin as assessed with viability assays as well as Western blotting, clonogenic assay, and FACS analysis.
Drugs can be entrapped and released from hydrogel nanoparticles due to the good swelling, biodegradability, and size distribution properties. The in vitro release rate of curcumin showed the sustained release from hydrogels with increased cross-linking, and the releasing rate was also dependent on the pH of the releasing medium. Although further studies are required to prove the efficacy of this formulation in vivo, successful implementation of curcumin nanogels with some modifications may prove very advantageous to assign curcumin as a chemotherapeutic rather than a chemopreventive agent.
Conclusion
Biocompatible, ph-sensitive, and biodegradable hydrogels as novel drug delivery systems were developed to achieve localized drug delivery. The inverse microemulsion polymerization method was used to synthesize PEG cross-linked acrylic polymers and the loading and release of curcumin was investigated. Intracellular and cytotoxicity studies were carried out in HeLa cell lines. A possible cause for higher anticancer activity could be higher cellular uptake of curcumin nanogels. Encapsulation of curcumin within cross-linked hydrogels shows comprehensive results, justifying the potential use of curcumin nanogels with better solubility, greater cellular uptake, sustained release, and enhanced antiproliferative effect to induce apoptosis in aqueous medium compared with free curcumin in DMSO. Overall, the results suggest curcumin nanogels are likely to have great potential as therapeutic agents.
Acknowledgements
The authors thank the Department of Biotechnology, Government of India, for financial support and the Sophisticated Analytical Instruments Facility (SAIF) Kerala for carrying out IR, DSC, and NMR.
Disclosure
The authors report no conflicts of interest in this work.
References
- KimISJeongYIKimDHLeeYHSung-Ho KimSHAlbumin release from biodegradable hydrogels composed of dextran and poly(ethylene glycol) macromerArch Pharm Res200124697311235815
- PeppasNAKhareARPreparation, structure and diffusional behavior of hydrogels in controlled releaseAdv Drug Deliv Rev199311135
- PeppasNABuresPLeobandungWIchikawaHHydrogels in pharmaceutical formulationsEur J Pharm Biopharm200050274610840191
- BrazelCSPeppasNAMechanisms of solute and drug transport in relaxing, swellable, hydrophilic glassy polymersPolymer19994033833398
- SiegalRAFirestoneBNAPH-Dependent equilibrium swelling properties of hydrophobic polyelectrolyte copolymer gelsMacromolecules19882132543259
- NairKLJagadeeshanSNairSAKumarGSVBiological evaluation of 5-fluorouracil nanoparticles for cancer chemotherapy and its dependence on the carrier, PLGAInt J Nanomedicine201161685169721980233
- NairKLJagadeeshanSNairSAKumarGSVEvaluation of triblock copolymeric micelles of δ-Valerolactone and poly(ethylene glycol) as a competent vector for doxorubicin delivery against cancerJ Nanobiotechno2011942
- GanderBGurryRDoelkerPeppasNAEffect of polymeric network structure on drug release from crosslinked poly (vinyl alcohol) micromatricesPharm Res198965785842508077
- ColomboPSantiPBettiniRBrazelCSDrug release from swelling-controlled systemsWiseDHandbook of Pharmaceutical Controlled Release TechnologyNew YorkMarcel Dekker2000183209
- Brannon-PeppasLRecent advances in the use of biodegradable microparticles and nanoparticles in controlled drug deliveryInt J Pharm199511619
- KostJLangerREquilibrium swollen hydrogels in controlled release applicationsPeppasNAHydrogels in Medicine and PharmacyBoca RatonCRC Press1986395108
- KimBLa FlammeKPeppasNADynamic swelling behavior of pH-sensitive anionic hydrogels uses for protein deliveryJ Appl Polym Sci20038916061613
- KhareARPeppasNASwelling/deswelling of anionic copolymer gelsBiomaterials1995165595677492721
- LinCCMettersATHydrogels in controlled release formulations: network design and mathematical modellingAdv Drug Delivery Rev20065813791408
- BurugapalliKBhatiaDKoulVChoudharyVInterpenetrating polymer networks based on poly(acrylic acid) and gelatin I: swelling and thermal behaviorJ Appl Polym Sci200182217227
- HennickWEvan NostrumCFNovel cross linking methods to design hydrogelsAdv Drug Delivery Rev2002541326
- GoelAKunnumakkaraABAggarwalBBCurcumin as “Curecumin”: from kitchen to clinicBiochem Pharmacol20087578780917900536
- ShishodiaSSethiGAggarwalBBCurcumin: getting back to the rootsAnn N Y Acad Sci2005105620621716387689
- StrimpakosASSharmaRACurcumin: preventive and therapeutic properties in laboratory studies and clinical trialsAntioxid Redox Sign200810511546
- AnandPSundaramCJhuraniSKunnumakkaraABAggarwalBBCurcumin and cancer: an “old-age” disease with an “age-old” solutionCancer Lett200826713316418462866
- ChuangSEKuoMLHsuCHCurcumin-containing diet inhibits diethylnitrosamine-induced murine hepatocarcinogenesisCarcinogenesis20002133110657978
- InanoHOnodaMInafukuNChemoprevention by curcumin during the promotion stage of tumorigenesis of mammary gland in rats irradiated with {gamma}-raysCarcinogenesis199920101110357781
- KawamoriTLubetRSteeleVEChemopreventive effect of curcumin, a naturally occurring anti-inflammatory agent, during the promotion/progression stages of colon cancerCancer Res1999595979973206
- LiNChenXLiaoJInhibition of 7,12-dimethylbenz[a]anthracene (DMBA)-induced oral carcinogenesis in hamsters by tea and curcuminCarcinogenesis200223130712151348
- SinghSVHuXSrivastavaSKMechanism of inhibition of benzo[a]pyrene-induced forestomach cancer in mice by dietary curcuminCarcinogenesis19981913579744529
- VanderhoffJWBranfordEBTarkowskiHLInverse emulsion polymerizationAdv Chem Ser1962343251
- QiuYParkKEnvironment-sensitive hydrogels for drug deliveryAdv Drug Delivery Rev200153321339
- AntoRJVenkatramanMKarunagaranDInhibition of NF-kappaB sensitizes A431 cells to epidermal growth factor-induced apoptosis, whereas its activation by ectopic expression of RelA confers resistanceJ Biol Chem2003278254902549812714587
- PuliyappadambaVTCheriyanVTThulasidasanAKTNicotine-induced survival signaling in lung cancer cells is dependent on their p53 status while its down-regulation by curcumin is independentMolecular Cancer2010922020727180
- SreekanthCNBavaSVSreekumarEAntoRJMolecular evidences for the chemosensitizing efficacy of liposomal curcumin in paclitaxel chemotherapy in mouse models of cervical cancerOncogene2011303139315221317920
- Simbulan-RosenthalCMRosenthalDSIyerSBoularesAHSmulsonMETransient poly(ADP-ribosyl) ation of nuclear proteins and role of poly(ADP-ribose) polymerase in the early stages of apoptosisJ Biol Chem1998273137039593711
- BavaSVPuliappadambaVTDeeptiANairAKarunagaranDAntoRJSensitization of taxol-induced apoptosis by curcumin involves down-regulation of nuclear factor-kappaB and the serine/threonine kinase Akt and is independent of tubulin polymerizationJ Biol Chem20052806301630815590651
- AggarwalBBSungBPharmacological basis for the role of curcumin in chronic diseases: an age-old spice with modern targetsTrends in Pharmacol Sci200930859419110321
- MosesMABremHLangerRNovel delivery systems in cancer chemotherapySci Med20039264273
- MaedaHWuJSawaTMatsumuraYHoriKTumor vascular permeability and the EPR effect in macromolecular therapeutics: a reviewJ Control Release20006527128410699287
- PerraultSDWalkeyCJenningsTFischerHCChanWCMediating tumor targeting efficiency of nanoparticles through designNano Lett200991909191519344179
- MohantyCSahooSKThe in vitro stability and in vivo pharmacokinetics of curcumin prepared as an aqueous nanoparticulate formulationBiomaterials2010316597661120553984