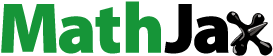
Abstract
Background
The intercalation of perindopril erbumine into Zn/Al-NO3-layered double hydroxide resulted in the formation of a host-guest type of material. By virtue of the ion-exchange properties of layered double hydroxide, perindopril erbumine was released in a sustained manner. Therefore, this intercalated material can be used as a controlled-release formulation.
Results
Perindopril was intercalated into the interlayers and formed a well ordered, layered organic-inorganic nanocomposite. The basal spacing of the products was expanded to 21.7 Å and 19.9 Å by the ion-exchange and coprecipitation methods, respectively, in a bilayer and a monolayer arrangement, respectively. The release of perindopril from the nanocomposite synthesized by the coprecipitation method was slower than that of its counterpart synthesized by the ion-exchange method. The rate of release was governed by pseudo-second order kinetics. An in vitro antihypertensive assay showed that the intercalation process results in effectiveness similar to that of the antihypertensive properties of perindopril.
Conclusion
Intercalated perindopril showed better thermal stability than its free counterpart. The resulting material showed sustained-release properties and can therefore be used as a controlled-release formulation.
Background
The administration of drugs designed to be given as a single dose rather than as multiple doses has recently been made possible using controlled-release formulations. In this way, drug release can be accomplished over long periods of time, enabling an almost constant level of the drug to be maintained in the bloodstream. Moreover, sustained-release formulations increase the clinical efficacy of drugs.Citation1 The introduction of drug nanocomposites as sustained-release vehicles has provided a breakthrough in novel drug delivery systems in the field of pharmaceutical technology. Citation2 Layered double hydroxides (LDH) are widely used for this purpose. Because of their unique properties, such as ease of preparation, low cost, good biocompatibility, low cytotoxicity, and full protection of the drugs loaded,Citation3–Citation5 LDHs have received considerable attention in the development of sustained-release or controlled-release drug delivery systems.
Layered double hydroxides are also known as hydrotalcite-like materials and anionic clays. They consist of brucite-like layers containing hydroxides of metal cations M2+ and M3+, and possess exchangeable anions, An− and a variable number of water molecules in the interlayer space. The chemical composition of LDH can be represented by the general formula: [M1−x2+ Mx3+(OH)2]x+(An−)x/n · mH2O.Citation5
Due to the anionic exchange properties of LDH, many pharmaceutically active compounds, especially those with negative charges or anions, have been intercalated into LDH brucite-like interlayers. As a result of ionic interactions, the intercalated actives are more stable, and usually show sustained release of the cargo molecules, which subsequently results in sustained drug levels. Such a property has been exploited for the controlled-release formulation of drugs such as the anticancer agent, 5-fluorouracil,Citation6 the anti-inflammatory drug fenbufen,Citation7 the antihypertensive drugs captopril, lisinopril, enalapril, and ramipril,Citation8 and cardiovascular drugs, such as pravastatin and fluvastatin.Citation9
Perindopril, (2S, 3aS, 7aS)-1-[(2S)-2-[[(2S)-1-ethoxy-1- ox-opentan-2-yl]amino]propanoyl]-2,3,3a,4,5,6,7,7a-octahydroin- dole-2-carboxylic acid is an antihypertensive drug that acts by inhibiting angiotensin-converting enzyme (ACE).Citation10 Perindopril is a prodrug ester that is converted into the active diacid, perindoprilat, by hydrolysis after administration.Citation11 It is administered orally in the form of tablets in a 1:1 ratio of perindopril salt with erbumine (tert-butylamine).
Complementary to previous research work,Citation12,Citation13 and due to the lack of previous studies on the intercalation of perindopril erbumine (PE), we selected this agent as a model drug and intercalated it with Zn/Al-LDH using ion-exchange and coprecipitation techniques. We focused our work on the structure, thermal properties, and slow-release properties of the as-synthesized drug-LDH nanocomposite, with the aim of using the resulting LDH-perindopril nanocomposite as a controlled-release formulation of the active drug, and study the effect of resulting nanocomposites on the angiotensin-converting enzyme.
Materials and methods
Materials
PE (C23H43N3O5, molecular weight 441.6) was purchased from CCM Duopharma (Berhad, Malaysia) at 99.79% purity and used as received. Other materials, including Zn (NO3)2 · 6H2O, Al (NO3)3 · 9H2O, NaOH, ACE, and hippuryl-histidyl-leucine, were purchased from Sigma (St Louis, MO) and used as received. Deionized water was used in all experiments.
Synthesis of Zn/Al-NO3 LDH
Zn/Al-NO3 was prepared according to the method described by MiyataCitation14 with minor modifications. An aqueous solution of 2 mol/L NaOH was added dropwise to a solution (250 mL) containing Zn2+ and Al3+ (molar ratio of Zn2+ to Al3+ is 4:1) with vigorous stirring until a pH of 7 was reached; the resulting solution was kept under a nitrogen gas environment. The slurry was aged at 70°C for 18 hours, and then centrifuged and washed with deionized water and dried in an oven at 60°C.
Synthesis of PE-Zn/Al nanocomposite by ion-exchange
PE was intercalated into Zn/Al-NO3 using the ion-exchange method. An aqueous solution containing 3.54 g (0.008 mol) of PE in 100 mL of decarbonated water was added to 50 mL of an aqueous suspension containing 0.2 g of Zn/Al-NO3. The mixture was magnetically stirred for 2 hours at room temperature. The resulting material was filtered, washed with water, and finally dried in an oven at 60°C. The product was denoted as PZAE (perindopril intercalated into Zn/Al by ion-exchange).
Synthesis of PE-Zn/Al nanocomposite by coprecipitation
The PE-intercalated Zn/Al-NO3 was prepared using the coprecipitation method. An aqueous solution containing 2 mol/L NaOH and PE (4.43 g, 0.01 mol) was added dropwise into a solution (250 mL) containing Zn2+ and Al3+ nitrate (molar ratio of Zn2+ to Al3+ was 4:1) under a nitrogen atmosphere with vigorous stirring until a pH of 7 was reached. The resulting slurry was kept at 70°C for 18 hours. The aged slurry was then filtered, washed with water, and finally dried in an oven at 60°C. The product was denoted as PZAC (perindopril intercalated into Zn/Al by coprecipitation method).
Controlled-release study
Drug release profiles were determined at room temperature using phosphate-buffered saline at a concentration of 0.01 mol/L and pH 4.8 and 7.4. About 85 mg of each nanocomposite was added to 500 mL of the media. The cumulative amount of PE released into the solution was measured at preset time intervals at λmax = 215 nm using a Perkin Elmer ultraviolet-visible spectrophotometer (model Lambda 35; PerkinElmer, Waltham, MA).
To compare the release rate of perindopril from LDH with that from the physical mixture of PE and Zn/Al-LDH, 0.62 mg of a physical mixture of PE (0.2 mg) and the pristine LDH (0.42 mg) was used. Release of the active perindopril was determined as described above.
MTT cytotoxicity and in vitro antihypertensive assay
Normal Chang liver cells was seeded at a density of 1 × 104 cells/well into 96-well plates and kept at 5% CO2 and 37°C for 24 hours. The cell was then treated with PE, PZAE, and PZAC at concentrations of 1.25, 0.625, 0.313, and 0.156 μg/mL. After 24 hours of incubation, cell viability was determined using a colorimetric assay based on the uptake of MTT by viable cells. The MTT-containing medium was removed gently and replaced with dimethylsulfoxide (200 μL/well) to mix the formazan crystals until dissolved. Absorbance at 570 nm and 630 nm (background) was measured with a microtiter plate reader. All experiments were carried out in triplicate. The IC50 was generated from the dose-response curves for the cell line.
The in vitro antihypertensive activity of free perindopril and intercalated perindopril in LDH, (PZAE and PZAC) was assayed by measuring their ACE inhibition activity using the method reported by Cushman and Cheung.Citation15 In vitro ACE inhibition activity was quantified by converting hippuryl-histidyl-leucine to hippuric acid through ACE in the presence of the inhibitor; absorbance was measured at 228 nm. Any reduction in absorbance is proportional to the inhibition exerted by the assayed inhibitor. Perindopril, PZAE, and PZAC (0.5 μg/mL) were preincubated with ACE for 20 minutes in a 25 μL volume in buffer (pH 8.3), then mixed with 10 μL of 3.5 mM hippuryl-histidyl-leucine. The assay mixture was incubated at 37°C for 30, 60, and 90 minutes. The reaction was stopped by adding 50 μL of 3 mol/L HCl. The hippuric acid formed by ACE was extracted with 1 mL of ethyl acetate. The solvent was removed by heat evaporation and re-dissolved in deionized water. The amount of hippuric acid was measured using a fluorescent plate reactor at 228 nm.
Characterization
Powder x-ray diffraction patterns were recorded in the range of 2°C–70°C on a Shimadzu diffractometer, XRD-6000, using CuKα radiation (λ = 1.5418 Å) at 30 kV and 30 mA, with a dwell time of 0.5 degrees per minute. Fourier transform infrared (FTIR) spectra of the materials were recorded over the range of 400–4000 cm−1 on a Thermo Nicolet Nexus FTIR (model Smart Orbit) with 4 cm−1 resolution, using the KBr disc method with a 1% sample in 200 mg of spectroscopic grade potassium bromide, and making the pellets by pressing at 10 tonnes. The chemical composition of the samples was analyzed for zinc and aluminum ions by inductively coupled plasma atomic emission spectrometry using a Perkin-Elmer spectrophotometer (model Optima 2000DV) under standard conditions. For carbon, hydrogen, nitrogen, and sulfur (CHNS) analyses, a CHNS-932 LECO instrument was used. Thermogravimetric and differential thermogravimetric analyses were carried out using a Mettler Toledo instrument with a heating rate of 10°C/minute, in the range of 20°C –1000°C under a nitrogen atmosphere (N2 flow rate 50 mL/minute). Surface characterization of the material was carried out using the nitrogen gas adsorption-desorption technique at 77 K and a Micromeritics ASAP 2000 instrument. A field emission scanning electron microscope (Nova Nanosem 230 model) was used to determine the surface morphology of the samples. Ultraviolet-visible spectra were measured to determine the optical properties, and a controlled release study was performed using a Perkin Elmer ultraviolet-visible spectrophotometer (Lambda 35).
Results and discussion
Powder X-ray diffraction
The x-ray diffraction patterns of perindopril intercalated into Zn/Al-LDH for the formation of nanocomposites obtained by both the ion-exchange and coprecipitation routes are depicted in , respectively. represents the x-ray diffraction pattern of Zn/Al-LDH. As shown in , the 003, 006, and 009 reflections were found for 2θ at about 9.79, 19.72, and 29.74 degrees, respectively. In addition, the two 110 and 113 reflections were found at 60.3 and 61.2 degrees, respectively. The basal spacing value (d003, obtained by averaging the higher order peaks) of the pristine Zn/Al-NO3 was 9.01 Å, which is consistent with the data in the literature.Citation16 The characteristic reflections of perindopril shown in were absent from the x-ray diffraction pattern of the product of reaction of PE with Zn/Al-NO3 LDH, suggesting that perindopril was intercalated into the interlayer galleries of the LDH rather than adsorbed onto the surface. This was confirmed by the shifting of the 003, 006, and 009 diffraction peaks to low 2θ angles at 4.00, 8.31, and 12.5 degrees, respectively, when using the ion-exchange method (), and 4.29, 8.87, and 13.26 degrees, respectively, when using the coprecipitation method ().
Figure 1 Powder X-ray diffraction patterns for pristine Zn/Al-NO3 (A), the nanocomposite prepared using the ion-exchange method, PZAE (B), the coprecipitation method, PZAC (C), and perindopril erbumine (D).
Abbreviations: Zn, zinc; Al, aluminum; LDH, layered double hydroxide; PZAE, perindopril intercalated into Zn/Al by ion-exchange; PZAC, perindopril intercalated into Zn/Al by coprecipitation method.
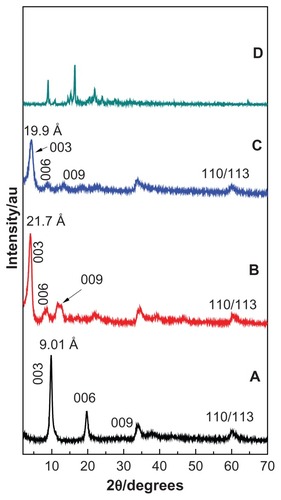
The lattice parameters of the Zn/Al-LDH, PZAE, and PZAC samples were calculated from the positions of the maxima due to reflection by planes, ie, 003 for parameter c, where [c = 1/3(3d003 + 6d006 + 9d009), and 110 for parameter a, where (a = 2d110)], as shown in . The reflection line of diffraction peak 110 (1.53 Å) for the Zn/Al-LDH sample did not shift after the intercalation process, which indicates that intercalation of perindopril did not change the structure of the layer, but only changed the interlayer spacing.Citation17
Table 1 Lattice parameters and surface properties of samples
Molecular structure and spatial orientation of intercalated perindopril
The PE consisted of perindopril (free acid, ) combined with erbumine (tert-butylamine). This combination incorporates hydrogen bonds () or involves the transfer of protons from the carboxylic group of perindopril to tert-butylamine (). During the intercalation process, the free acid of PE (), which is negatively charged on the carboxylic acid group, is preferentially intercalated into the inorganic interlayers of LDH. Therefore, we presumed that the perindopril anion of PE was intercalated into the inorganic interlayers. We subsequently estimated the dimensions of the perindopril anion and its spatial orientation.
Figure 2 Structure of perindopril erbumine (A–C) and molecular structural models of perindopril intercalated between the interlayers of LDH (D and E).
Abbreviation: LDH, layered double hydroxide.
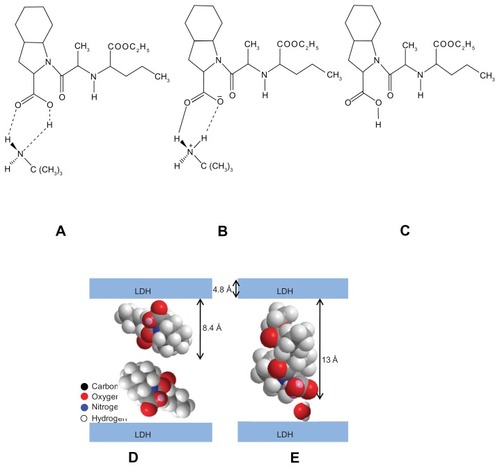
The X-ray diffraction pattern shows that the d-spacing (d003) of the nanocomposites increased to 21.7 (obtained by averaging the higher three order peaks) and 19.9 Å (obtained by averaging the higher five order peaks) for PZAE and PZAC, respectively. Because the thickness of the LDH layer is constant at 4.8 Å,Citation18 the gallery height of LDH after intercalation can thus be calculated by the d-spacing minus the thickness of the LDH layer; these values were 16.9 Å (21.7–4.80 Å) and 15.1 Å (19.9–4.80 Å) for PZAE and PZAC, respectively. The long and short axes (x-axes and y-axes, respectively) and molecular thickness (z-axis) of perindopril were calculated using Chemoffice software (Cambridge, MA), giving values of 13 Å, 8.4 Å, and 12.6 Å, respectively. The gallery height of PZAE was 16.9 Å, which is much larger than the value of the long axis (13 Å), and slightly similar to double the short axis (16.8 Å). This suggests that the perindopril anions in PZAE were accommodated as an alternate bilayer, as illustrated in . A comparison of the long axis of perindopril (13 Å) with a gallery height of PZAC (15.1 Å) suggests that, in PZAC, perindopril anions were accommodated as a vertical monolayer (along the axis of perindopril) with the presence of water between the perindopril anions and this layer ().Citation19
Infrared spectroscopy
The FTIR spectrum of Zn/Al-NO3 LDH is shown in . The absorption peak at 1384 cm−1 is due to nitrate groups. The broad absorption peak at 3452 cm−1 can be attributed to stretching of the O-H group, which is contained within the layer. The absorption peak at 1628 cm−1 is due to the bending of H2O,Citation20 while the absorption at 428 cm−1 is due to Zn-Al-OH stretching.Citation21
Figure 3 Fourier transform infrared patterns of (A) perindopril erbumine, (B) Zn/Al-NO3, (C) PZAE, and (D) PZAC.
Abbreviations: Zn, zinc; Al, aluminum; LDH, layered double hydroxide; PZAE, perindopril intercalated into Zn/Al by ion-exchange; PZAC, perindopril intercalated into Zn/Al by coprecipitation method.
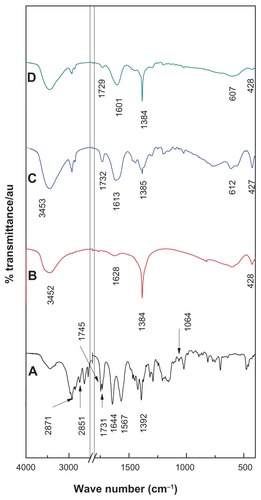
The FTIR spectra of PE ( and ) show many intense, sharp absorption peaks that are due to the different functional groups present in the molecules, ie, primary amine, secondary amine, ester, carboxylic acid and methyl groups. Tert-butylamine shows three absorption peaks at 2871 cm−1, which can be attributed to the asymmetric stretching of CH3,Citation22 while the peak at 1247 cm−1 is due to C3C−N stretchingCitation22 and 1022 cm−1 indicates C−N stretching.Citation23 The band at 2851 cm−1 can be attributed to CH in CH3−CH−NH and the band at 2929 cm−1 is due to CH in NH−CH-propyl. The secondary amine functional group shows a peak at 1154 cm−1 which is related to the symmetric stretching of C−N−C.Citation23 The bands recorded at 1745, 1209, and 1064 cm−1 are due to C=O, C−C−O, and O−C−C stretching, respectively, in the ester group.Citation23 The band at 1731 cm−1 is due to the ν(C=O) stretching mode of the carboxylic group, which indicates that PE can exist in a neutral formCitation24 (). The band at 1291 cm−1 is due to C−O stretching in the carboxylic acid group.Citation25
Table 2 Assignment of FTIR absorption bands from PE, PZAE, and PZAC nanocomposites
The FTIR spectra of the PZAE and PZAC are shown in , respectively, and the spectral assignments are shown in . The nanocomposite spectrum shows the characteristic bands of pure perindopril, which indicate that the perindopril anions are intercalated into the interlayer galleries of LDH. shows that some of the bands are slightly shifted in position due to the interaction between the perindopril anion and the interlayers as a result of the intercalation process. The bands recorded at 1732 cm−1 and 1729 cm−1 for the PZAE and PZAC nanocomposites, respectively, are due to the presence of perindopril in a neutral form adsorbed on the layer or due to an ester group.Citation26,Citation27 The appearance of new broad peaks at 1613 cm−1 for PZAE and 1601 cm−1 for PZAC can be assigned to asymmetry stretching vibration of the COO− group. The shift in COO− asymmetry for the PZAC nanocomposite to a lower wave number compared with PZAE presumably occurred due to the presence of water molecules between the layers, which bind the perindopril molecules and the inorganic interlayer.Citation28 The band at 1384 cm−1 is due to the nitrate anion, which may not be completely removed from the interlayers during the intercalation process, and to the symmetric stretching vibration of the COO− functional group.Citation29
Figure 4 Thermogravimetric and differential thermogravimetric analyses of perindopril (A), Zn/Al-NO3 (B), PZAE (C), and PZAC (D)
Abbreviations: Zn, zinc; Al, aluminum; NO3, nitrate; PZAE, perindopril intercalated into Zn/Al by ion-exchange; PZAC, perindopril intercalated into Zn/Al by coprecipitation method.
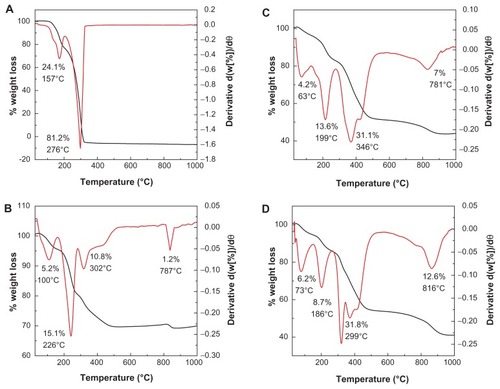
The bands below 600 cm−1 are due to metal-oxygen bonds; ν(M-O-M) stretching modes in the layers indicate that the structure of the layers is well maintained.Citation30,Citation31
Elemental analysis
Elemental analysis was conducted to determine the organic and inorganic composition of PZAE, PZAC, and Zn/Al-NO3 LDH. As expected, the PZAE and PZAC nanocomposites contained both organic and inorganic constituents. This indicates that intercalation occurred in which perindopril was intercalated into the LDH inorganic interlayers.
shows that the Zn2+/Al3+ molar ratio in Zn/Al-NO3 LDH, PZAE, and PZAC is 3.5, 3.45, and 2.6, respectively. As a result of the elemental chemical analysis and thermogravimetric studies, the empirical formula for LDH was determined to be [Zn0.78Al0.22(OH)2](NO3−)0.22 · 0.31H2O. According to the FTIR spectra of PZAC and PZAE, the strong bands at 1384 cm−1 and 1385 cm−1 are due to the presence of trace amounts of a nitrate group in the interlamellar spacing of LDH. Based on the results of elemental chemical analysis and thermogravimetric studies, the empirical formula for PZAC was proposed to be [Zn0.72Al0.28(OH)2](PE−)0.177(NO3−)0.103 · 0.91H2O. The empirical formula for the PZAE nanocomposite can be given as [Zn0.78Al0.22(OH)2](PE−)0.19(NO3−)0.03 · 0.4H2O.
Table 3 Chemical compositions of Zn/Al-NO3 LDH, PZAE, and PZAC nanocomposites
Thermal analysis
The thermal behavior of the compounds before and after intercalation of perindopril into LDH was examined using thermogravimetric and differential thermogravimetric analyses. The thermal analysis of Zn/Al-LDH, PZAE, PZAC, and pure PE are shown in . For PE (), two main thermal events were clearly observed. The first event, which occurred in the region of 90°C–192°C, was attributed to the melting of perindopril, corresponding to a sharp peak in the differential thermogravimetric curve at 157°C with 24.1% weight loss. This was followed by the second stage at 192°C–312°C, due to the decomposition and subtle combustion of perindopril,Citation32 corresponding to the strong peak at 276°C and 81.2% weight loss. shows that the thermal decomposition of Zn/Al-LDH progressed through four major stages of weight loss; these occurred at temperature maxima of 100°C, 226°C, 302°C, and 787°C, with weight losses of 5.2%, 15.1%, 10.8%, and 1.2%, respectively. The first stage of weight loss in the range 32°C–152°C is due to the removal of water physisorbed on the external surface of LDH as well as structured water. The second and third stages of weight loss can be attributed to dehydroxylation of the metal hydroxide layers and decomposition of nitrate ions, and were completed at 484°C. The final stage of weight loss is related to the formation of spinel.Citation33
Following the intercalation process, the thermal decomposition characteristics of the resulting product were significantly different from those of the precursors. The thermogravimetric analysis curves for PZAE and PZAC showed four stages of weight loss. For PZAE (), the first weight loss of 4.2% was attributed to the elimination of surface water or physically adsorbed water and interlayer water at 63°C.Citation34 The second and third weight losses were attributed to dehydroxylation of the layers and combustion of the perindopril at 199°C and 346°C, respectively. The second and third stages usually overlapped.Citation35 The fourth step with 7% weight loss at 781°C was due to the formation of ZnAl2O4.
The thermal decomposition characteristics of PZAC were similar to those of PZAE. Three weight losses at 73°C, 186°C, and 299°C were associated with the removal of surface-adsorbed water, interlayer water, and dehydroxylation of the layer, as well as decomposition of perindopril, accompanied by 6.15%, 8.69%, and 31.8% weight loss, respectively (). Decomposition of perindopril in the PZAE and PZAC nanocomposites occurred at 346°C and 299°C, respectively, which is higher than that for free perindopril (276°C). This suggests that the thermal stability of perindopril in nanocomposites was clearly enhanced due to the intercalation process, which involves electrostatic attraction between the negatively charged functional group of perindopril and the positively charged brucite-like layers of LDH.
Surface properties
shows the nitrogen adsorption-desorption isotherms for Zn/Al-NO3, PZAE, and PZAC. All adsorption isotherms were Type IV according to the International Union of Pure and Applied Chemistry classification, indicating a mesoporous-type material.Citation36 Adsorption increased slowly at low relative pressure in the range of 0.0–0.8, followed by rapid adsorption at relative pressures >0.8, reaching an optimum at more than 7.1, 6.9, and 18.9 cm3/g at standard temperature and pressure for Zn/Al-NO3, PZAE, and PZAC, respectively. The desorption branch of the hysteresis loop in PZAE and PZAC was wider than that of Zn/Al-NO3, indicating a difference in pore texture. This was due to modification of the pores as a result of the expansion of basal spacing due to the formation of the new nanocomposites.Citation21
Figure 5 Adsorption-desorption isotherms (A) and BJH pore size distributions (B) for Zn/Al-NO3, PZAE, and PZAC.
Abbreviations: BJH, Barret-Joyner-Halenda; Zn, zinc; Al, aluminum; NO3, nitrate; PZAE, perindopril intercalated into Zn/Al by ion-exchange; PZAC, perindopril intercalated into Zn/Al by coprecipitation method; STP, standard temperature pressure.

shows the Br unauer-Emmet t -Tel ler (BET)-specific surface area of Zn/Al-NO3 LDH and the nanocomposites, PZAE and PZAC. As shown in , the BET-specific surface area of the resulting nanocomposites was higher than that of LDH. The BET surface area increased from 1 m2/g for Zn/Al-NO3 to 2 m2/g for PZAE and 7 m2/g for PZAC.
shows the Barret-Joyner-Halenda (BJH) desorption pore size distribution for Zn/Al-NO3, PZAE, and PZAC. As shown, a single-peaked pore size distribution was observed for Zn/Al-NO3, centered around 178 Å. On the other hand, PZAE and PZAC showed single peaks at 185 Å and 109 Å, respectively. The average pore volume and pore diameter shown by BJH desorption are given in . The BJH average pore diameters for Zn/Al-NO3, PZAE and PZAC were 243 Å, 135 Å, and 157 Å, respectively; the BJH pore volumes for the same samples were 0.008, 0.009, and 0.025 cm3/g, respectively.
The surface morphology of Zn/Al-NO3 LDH, PZAE, and PZAC is illustrated in . All samples showed typical non-uniform irregular agglomerates of compact and non-porous plate-like structures, similar to the morphology of nanocomposites discussed in the literature.Citation37 PZAC had a smoother surface morphology than Zn/Al-NO3 LDH and PZAE.
Sustained-release study
The cumulative release profiles of perindopril from PZAE and PZAC, and its physical mixture, PE, with Zn/Al-LDH in solution at pH 7.4 and 4.8, are shown in . The physical mixture showed no sustained-release effects in both solutions at pH 7.4 and 4.8, with the total PE content released immediately. At pH 7.4 and 4.8, the release rate within the first 12 minutes reached 87% and 97%, respectively. However, the perindopril-intercalated LDH nanocomposite gradually released perindopril anions over time, both at pH 7.4 and 4.8. At pH 4.8 ( [B and D]), the first aliquot contained a large number of perindopril anions due to the “burst effect”,Citation38 together with the release of perindopril anions adsorbed onto the outer surface of LDH. Subsequently (in acid media at pH 4.8), the LDH particles were destroyed and the intercalated perindopril drug anions were released. The destruction of the layers increased the acidity (pH) of the solution and increased the rate of perindopril release in this medium. Therefore, release was faster at pH 4.8 than at pH 7.4. When the drug–LDH dissolution process reached equilibrium, 87% was released in 1100 minutes and 1020 minutes for PZAE and PZAC, respectively ().
Figure 7 (I) Release profiles of perindopril from the PZAE sample at pH 7.4 (A) and pH 4.8 (B), and from the PZAC sample at pH 7.4 (C) and pH 4.8 (D) up to 400 minutes, (II) release profiles of perindopril up to 1260 minutes. Inset shows the release profiles of perindopril from its physical mixture of perindopril with Zn/Al-NO3 at pH 7.4 (E) and pH 4.8 (F).
Abbreviations: Zn, zinc; Al, aluminum; NO3, nitrate; PZAE, perindopril intercalated into Zn/Al by ion-exchange; PZAC, perindopril intercalated into Zn/Al by coprecipitation method.
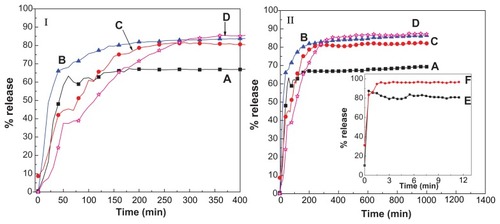
At pH 7.4 (), the release of perindopril was rapid within the first 200 and 300 minutes, which can be attributed to the release of perindopril anions adsorbed on the outer surface of LDH as well as intercalated perindopril. Subsequently, however, the release of perindopril became much slower and sustained compared with the solution at pH 4.8, with a total release equilibrium of 83% in 1263 minutes for PZAC and 70% in 1000 minutes for PZAE (). This sustained-release process may be attributed to the ion-exchange process between the perindopril anions and the anions in the buffer solution.Citation39,Citation40 The release profile of perindopril from PZAE and PZAC shown in is similar to that of other drugs from the host.Citation6,Citation8,Citation41–Citation43 Additionally, at pH 7.4, the duration of release of perindopril, which was arranged as a monolayer in the PZAC sample, was much longer than that of PZAE, which was arranged in a bilayer fashion (). It is possible that the perindopril anions that were arranged in a monolayer fashion in the interlayer showed weaker repulsive forces and a stronger affinity for the LDH interlayers. Thus, these structures may have been more difficult to destroy compared with the anions arranged in bilayers.
Figure 8 Fitting the perindopril release data from PZAE samples (A and B) and from PZAC samples (C and D) at pH 4.8 and 7.4.
Abbreviations: PZAE, perindopril intercalated into Zn/Al by ion-exchange; PZAC, perindopril intercalated into Zn/Al by coprecipitation method.
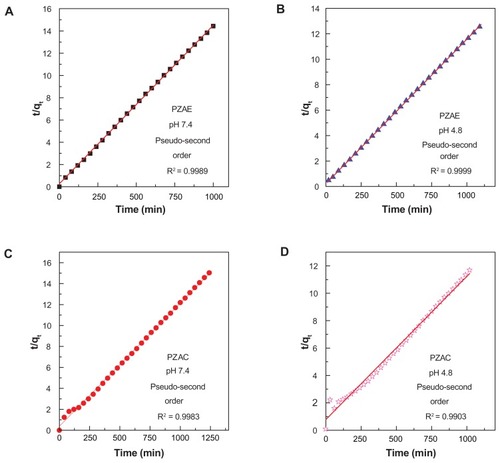
To gain more insight into the mechanism of release of perindopril from the nanocomposites, we used three types of kinetic models to fit the release data:
The pseudo-first order model,Citation41 which expresses release of perindopril from LDH; here the dissolution rate depends on the amount of perindopril in the nanocomposite and can be expressed as:
(1)
The pseudo-second order kinetic equation,Citation41 which is represented in the form:
(2)
where qe and qt are the equilibrium release amount and the release amount at any time (t), respectively, and h = k2qe2.
The parabolic diffusion model,Citation44 which can be expressed as:
(3)
where a is constant and kd is the overall diffusion constant for release. Mo and Mt are the amount of perindopril between the layers at release time 0 and t, respectively.
The data on perindopril release from the nanocomposites into the solutions were fitted into the above mentioned equations. The corresponding linear coefficient (R2) was evaluated, is summarized in Table 4, and is plotted in . As shown, the pseudo-second order model was the most satisfactory for describing the release kinetics of perindopril from the nanocomposites, similar to previous studies reported in the literature.Citation41 shows the plots of t/qt against t for the release of perindopril at pH 4.8 and 7.4. As shown, straight lines were obtained. For the release of perindopril at pH 4.8, the correlation coefficient values were 0.9999 and 0.9903 for PZAE and PZAC, respectively, whereas the correlation coefficients at pH 7.4 were 0.9989 and 0.9983 for PZAE and PZAC, respectively.
shows the pseudo-second order rate constant for the release of perindopril from PZAE and PZAC. At pH 4.8, this was 5.7 × 10−4 and 1.3 × 10−4 mg/minute, respectively. At 7.4, the values were 7.4 × 10−4 and 3.8 × 10−4 mg/minute, respectively.
Table 4 Correlation coefficient (R2), rate constants (k), and half-time (t1/2) obtained by fitting the perindopril release data from PZAE and PZAC nanocomposites into solutions at pH 4.8 and 7.4
MTT cytotoxicity and in vitro antihypertensive activity
show the effect of PE, PZAE, and PZAC on viability, using Chang cells at various concentrations of 0.156, 0.313, 0.625, and 1.25 μg/mL and with 24-hour incubation times. As shown in , PE did not have a cytotoxic effect on the viability of these cells. Interestingly, the PZAE and PZAC also had no toxic effect on the Chang cells at a concentration up to 1.25 μg/mL. These results are consistent with those of a study reported by Posati et al who showed that cytotoxicity is apparently selective for human cervical adenocarcinoma epithelial (HeLa) cells, because there is no significant cytotoxicity towards non-malignant Madin-Darby canine kidney cells.Citation45
Figure 9 MTT assays of normal Chang liver cells after 24 hours of treatment with perindopril erbumine, PZAE, and PZAC.
Abbreviations: PZAE, perindopril intercalated into Zn/Al by ion-exchange; PZAC, perindopril intercalated into Zn/Al by coprecipitation method.

Several other investigations have demonstrated the antihypertensive potential of PE.Citation46–Citation48 and show the first report of free Zn/Al layered double hydroxide and PE intercalated into Zn/Al, with ACE inhibition activity. The ACE inhibition activity of perindopril in PZAE and PZAC nanocomposites was determined in vitro by monitoring the transformation from a substrate hippuryl-histidyl-leucine to the product hippuric acid. Of the two formulations, the PZAE showed the most potent ACE inhibition activity. That may be attributable to the high level of PE release from the PZAE nanocomposite when compared with PZAC. However, the ACE inhibition activity of both formulations was time-dependent. Zn/Al layered double hydroxide did not show that inhibition. Following incubation of ACE with 0.5 μg/mL of PZAE, approximately 43% and 68% decreases in ACE activity were observed at 30 and 60 minutes of incubation, respectively, as compared with the untreated cells. Under these same conditions, incubation of ACE with PZAC (0.5 μg/mL) resulted in 21% and 55% decreases in ACE activity at these same time points, respectively. shows slightly decreases in ACE activity with 0.5 μg/mL of PZAE and PZAC for 90 minutes as compared with that for 60 minutes.
Table 5 Angiotensin-converting enzyme activity for PE, PZAE, and PZAC with different exposure times
Figure 10 Angiotensin-converting enzyme inhibition (%) for free perindopril and perindopril intercalated into PZAE and PZAC after 30, 60, and 90 minutes of exposure time.
Abbreviations: PE, perindopril erbumine; PZAE, perindopril intercalated into Zn/Al by ion-exchange; PZAC, perindopril intercalated into Zn/Al by coprecipitation method.

Conclusion
This work shows that intercalation of perindopril, an antihypertensive drug, into Zn/Al-LDH can be accomplished using two methods, namely ion-exchange and coprecipitation. The basal spacing of the nanocomposites was expanded to 21.7 Å and 19.9 Å when using ion-exchange and coprecipitation, respectively. The results suggest that the perindopril molecules were arranged as a monolayer within the interlayers when using the coprecipitation method and as a bilayer when using the ion-exchange method. As a result of the intercalation process, the thermal stability and sustained release of the intercalated perindopril were considerably enhanced compared with its free counterpart. The duration of release of perindopril from the nanocomposite prepared by the coprecipitation method was much longer than that of perindopril prepared by the ion-exchange method at pH 7.4. It is possible that the intercalated guest molecules, arranged in a monolayer fashion within the interlayers, have a weaker repulsive force and stronger affinity towards the LDH interlayers. The release of perindopril from the nanocomposite was found to be governed by pseudo-second order kinetics. An in vitro antihypertensive assay showed that the intercalation process has similar effectiveness on the antihypertensive properties of perindopril.
Acknowledgment
We would like to thank the Ministry of Higher Education of Malaysia for funding the project under grant 05-03-10-1035 RUGS (Vot 9199644). The author is grateful to Universiti Putra Malaysia for International Graduate Research Fellowship (IGRF).
Disclosure
The authors declare that they have no competing interests in this work.
References
- RathboneMJHadgraftJRobertsMSModified-Release Drug Delivery Technology126New York, NYMarcel Dekker2003
- NeerajVTaehongMRhulMDevelopment of PEGylated PLGA nanoparticles for controlled and sustained drug delivery in cystic fibrosisJ Nanobiotechnology201082211820145735
- ChoiS-JChoyJHLayered double hydroxide nanoparticles as target-specific delivery carriers: uptake mechanism and toxicityNanomedicine20116580381421793673
- ShiWWeiMJinLLiCCalcined layered double hydroxides as a “biomolecular vessel” for bromelain: immobilization, storage and releaseJ Mol Catal B Enzym2007471–25865
- WilliamsGRO’HareDTowards understanding, control and application of layered double hydroxide chemistryJ Mater Chem2006163030653074
- WangZWangEGaoLXuLSynthesis and properties of Mg2 Al layered double hydroxides containing 5-fluorouracilJ Solid State Chem20051783736741
- LiBHeJGevansDDuanXInorganic layered double hydroxides as a drug delivery system – intercalation and in vitro release of fenbufenAppl Clay Sci2004273–4199207
- XiaSJNiZMXuQHuBXHuJLayered double hydroxides as supports for intercalation and sustained release of antihypertensive drugsJ Solid State Chem20081811026102619
- PandaHSSrivastavaRBahadurDIn-vitro release kinetics and stability of anticardiovascular drugs – intercalated layered double hydroxide nanohybridsJ Phys Chem B200911345150901510019831399
- OpieLHAngiotensin-Converting Enzyme Inhibitors: Scientific Basis for Clinical Use22nd edNew York, NYWiley-Liss New York1994
- PascardCGuilhemJVincentMRemondGPortevinBLaubieMConfiguration and preferential solid-state conformations of perindoprilat (S-9780). Comparison with the crystal structures of other ACE inhibitors and conclusions related to structure-activity relationshipsJ Med Chem19913426636691995891
- HusseinMZAl AliSHZainalZHakimMNDevelopment of antiproliferative nanohybrid compound with controlled release property using ellagic acid as the active agentInt J Nanomedicine201161373138321796241
- Al AliSHHAl-QubaisiMHusseinMZZainalZHakimMNPreparation of hippurate-zinc layered hydroxide nanohybrid and its synergistic effect with tamoxifen on HepG2 cell linesInt J Nanomedicine201163099311122163163
- MiyataSHydrotalcites in relation to compositionClays Clay Miner19802815056
- CushmanDWCheungHSSpectrophotometric assay and properties of the angiotensin-converting enzyme of rabbit lungBiochemical Pharmacology197120716371648
- TangPXuXLinYLiDEnhancement of the thermo- and photostability of an anionic dye by intercalation in a zinc-aluminum layered double hydroxide hostInd Eng Chem Res200847824782483
- KongXShiSHanJZhuFWeiMDuanXPreparation of glycy- L-tyrosine intercalated layered double hydroxide film and its in vitro release behaviorChem Eng J20101572–3598604
- CavaniFTrifiroFVaccariAHydrotalcite-type anionic clays: Preparation, properties and applicationsCatal Today1991112173301
- MeynMBenekeKLagalyGAnion-exchange reactions of layered double hydroxidesInorg Chem1990292652015207
- Carbajal ArizagaGGWypychFCastillon BarrazaFContreras LopezOEReversible intercalation of ammonia molecules into a layered double hydroxide structure without exchanging nitrate counter-ionsJ Solid State Chem20101831023242328
- HusseinMZHwaTKSynthesis and properties of layered organic-inorganic hybrid material: Zn-Al layered double hydroxide-dioctyl sulfosuccinate nanocompositeJ Nanopart Res200023293298
- KipkemboiPKKipronoPCSangaJJVibrational spectra of t-butyl alcohol, t-butylamine and t-butyl alcohol+ t-butylamine binary liquid mixturesB Chem Soc Ethiopia2003172211218
- SmithBCInfrared Spectral Interpretation: A Systematic ApproachBoca Raton, FLCRC1999
- MilanRMolecular structure and stability of per-indopril erbumine and perindopril l-arginine complexesEur J Med Chem200944110110818436349
- TrontoJReisMJDSilverioFBalboVRMarchettiJMValimJBIn vitro release of citrate anions intercalated in magnesium aluminium layered double hydroxidesJ Phys Chem Solids2004652–3475480
- KempaTJBartonZMCunliffeAVMechanism of the thermal degradation of prepolymeric poly(3-nitratomethyl-3-methyloxetane)Polymer19994016593
- Kir’yalovNAmirovaGTriterpene acids from the roots of Meristotropis triphylla Fisch. et MeyChem Nat Compd196515243245
- Morzyk-OciepaBMichalskaDPietraszkoAStructures and vibrational spectra of indole carboxylic acids. Part I. Indole-2-carboxylic acidJ Mol Struct20046881–37986
- YuanQWeiMEvansDGDuanXPreparation and investigation of thermolysis of L-aspartic acid-intercalated layered double hydroxideJ Phys Chem B2004108331238112387
- RivesVLayered Double Hydroxides: Present and FutureNew York, NYNova Science Publishing Inc2001
- KloproggeJTFrostRLFourier transform infrared and Raman spectroscopic study of the local structure of Mg-, Ni-, and Co-hydrotalcitesJ Solid State Chem19991462506515
- MacêdoROGomes do NascimentoTSoares AragoCFBarreto GomesAPApplication of thermal analysis in the characterization of anti-hypertensive drugsJ Therm Anal Calorim2000593657661
- ChengXHuangXWangXSunDInfluence of calcination on the adsorptive removal of phosphate by Zn-Al layered double hydroxides from excess sludge liquorJ Hazard Mater20101771–351652320060217
- AjatMMohdMYusoffKHusseinMZSynthesis of glutamate-zinc-aluminium-layered double hydroxide nanobiocomposites and cell viability studyCurr Nanosci200844391396
- VicenteRCharacterisation of layered double hydroxides and their decomposition productsMater Chem Phys2002751–31925
- PierottiRARouquerolJReporting physisorption data for gas/solid systems with special reference to the determination of surface area and porosityPure Appl Chem1985574603619
- RakiLBeaudoinJJMitchellLLayered double hydroxide-like materials: nanocomposites for use in concreteCem Concr Res200434917171724
- HuangXBrazelCSOn the importance and mechanisms of burst release in matrix-controlled drug delivery systemsJ Control Release2001732–312113611516493
- Bin HusseinMZZainalZYahayaAHFooDWVControlled release of a plant growth regulator, [alpha]-naphthaleneacetate from the lamella of Zn-Al-layered double hydroxide nanocompositeJ Control Release2002822–341742712175754
- AmbrogiVFardellaGGrandoliniGPerioliLTiraltiMCIntercalation compounds of hydrotalcite-like anionic clays with anti-inflammatory agents, II: uptake of diclofenac for a controlled release formulationAAPS Pharm Sci Tech2002337782
- DongLYanLHouW-GLiuSJSynthesis and release behavior of composites of camptothecin and layered double hydroxideJ Solid State Chem2010183818111816
- HouWGJinZLSynthesis and characterization of naproxen intercalated Zn-Al layered double hydroxidesColloid Polym Sci20072851314491454
- WeiMPuMGuoJIntercalation of L-dopa into layered double hydroxides: enhancement of both chemical and stereochemical stabilities of a drug through host-guest interactionsChem Mater2008201651695180
- KodamaTHaradaYUedaMShimizuK-IShutoKKomarneniSSelective exchange and fixation of strontium ions with ultrafine Na- 4-micaLangmuir2001171648814886
- PosatiTBellezzaFTarpaniLSelective internalization of ZnAl-HTlc nanoparticles in normal and tumor cells. A study of their potential use in cellular deliveryAppl Clay Sci20125506269
- ChrysantSGMcDonaldRHWrightJTBardenPLWeissRJPerindopril as monotherapy in hypertension: a multicenter comparison of two dosing regimensClin Pharmacol Ther19935344794848477565
- RahmanNAnwarNKashifMOptimized and validated initial-rate method for the determination of perindopril erbumine in tabletsChem Pharm Bull2006541333616394545
- SicaDADosage considerations with perindopril for systemic hypertensionAm J Cardiol20018871318