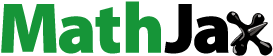
Abstract
Background
Alpha-momorcharin (α-MMC) and momordica anti-HIV protein (MAP30) derived from Momordica charantia L. have been confirmed to possess antitumor and antivirus activities due to their RNA-N-glycosidase activity. However, strong immunogenicity and short plasma half-life limit their clinical application. To solve this problem, the two proteins were modified with (mPEG)2-Lys-NHS (20 kDa).
Methodology/principal findings
In this article, a novel purification strategy for the two main type I ribosome-inactivating proteins (RIPs), α-MMC and MAP30, was successfully developed for laboratory-scale preparation. Using this dramatic method, 200 mg of α-MMC and about 120 mg of MAP30 was obtained in only one purification process from 200 g of Momordica charantia seeds. The homogeneity and some other properties of the two proteins were assessed by gradient SDS-PAGE, electrospray ionization quadruple mass spectrometry, and N-terminal sequence analysis as well as Western blot. Two polyethylene glycol (PEG)ylated proteins were synthesized and purified. Homogeneous mono-, di-, or tri-PEGylated proteins were characterized by matrix-assisted laser desorption ionization-time of flight mass spectrometry. The analysis of antitumor and antivirus activities indicated that the serial PEGylated RIPs preserved moderate activities on JAR choriocarcinoma cells and herpes simplex virus-1. Furthermore, both PEGylated proteins showed about 60%–70% antitumor and antivirus activities, and at the same time decreased 50%–70% immunogenicity when compared with their unmodified counterparts.
Conclusion/significance
α-MMC and MAP30 obtained from this novel purification strategy can meet the requirement of a large amount of samples for research. Their chemical modification can solve the problem of strong immunogenicity and meanwhile preserve moderate activities. All these findings suggest the potential application of PEGylated α-MMC and PEGylated MAP30 as antitumor and antivirus agents. According to these results, PEGylated RIPs can be constructed with nanomaterials to be a targeting drug that can further decrease immunogenicity and side effects. Through nanotechnology we can make them low-release drugs, which can further prolong their half-life period in the human body.
Video abstract
Point your SmartPhone at the code above. If you have a QR code reader the video abstract will appear. Or use:
Introduction
Momordica charantia L. (MC), a Momordica Linn. genus of the family Cucurbitaceae and commonly known as bitter melon, is a traditional medicine plant indigenous to China.Citation1 The fruit and seed extracts from MC have been used in China for centuries for antivirus, antitumor, and immunopotentiating agent purposes.Citation2 In recent years, several ribosome-inactivating proteins (RIPs), including momordica anti-HIV protein (MAP30) and α-momorcharin (MMC), β-MMC, and γ-MMC, a group of which belong to the family of single-chain RIPs, were found to have the ability to inhibit protein biosynthesis in tumor cells by catalytic inactivation of the 60S ribosomal subunit.Citation3,Citation4 These proteins were also found to be able to inhibit the multiplication of herpes simplex virus-1 (HSV-1),Citation4,Citation5 poliovirus I in Hep2 cells,Citation6 and acquired human immunodeficiency virus type-l (HIV-l).Citation7 However, the strong immunogenicity, allergic reaction, and short half-life of these proteins have been considered the major barriers for their application as therapeutic agents in vivo.Citation8,Citation9 In recent years, researchers have shifted their focus to other technologies. An established technology, polyethylene glycol (PEG) conjugation (PEGylation), can bestow on proteins several benefits, such as increasing plasma half-life, decreasing toxicity, and reducing immunogenicity and antigenicity.Citation9,Citation10 The Food and Drug Administration has approved the PEGylated forms of the therapeutic proteins such as uricase, erythropoietin, granulocyte-colony stimulating factor, interferon, adenosine deaminase, asparaginase, and a growth hormone antagonist. Another technology is nanotechnology, which uses nanomaterials for packing potential therapeutic proteins to extend the half-life period or to make them targeting drugs.
α-MMC and MAP30 as potential therapeutic proteins possess biological activities such as inhibiting protein biosynthesis (ribosome inactivation),Citation11 antitumor, antivirus, and, especially, anti-HIV replication.Citation7,Citation12,Citation13 However, as foreign proteins are like other potential therapeutic proteins, poor biocompatibility limits their further development and application. To overcome these problems, in this study we first purified the two main proteins from bitter melon seeds and carried out their PEGylation using a branched 20 kDa (mPEG)2-Lys-NHS directed specifically to lysil ɛ-amino groups. Homogeneous one-mer, two-mer, and three-mer PEG-RIPs were then identified by matrix-assisted laser desorption ionization-time of flight mass spectrometry (MALDI-TOF-MS). Not only was their immunogenicity in vivo remarkably decreased but also, importantly, their antitumor and antivirus activities in vitro were moderately influenced when compared with the un-PEGylated counterparts. This work is just the beginning step towards them being used clinically. The application of PEGylation and nanotechnolgy may indicate that the potential application of both α-MMC and MAP30 can be developed for antitumor and antivirus agents in the future.
Materials and methods
Materials
Bitter melon seeds were obtained from the Institute of Agricultural Science and Technique of Sichuan Province, China. Matrices for electrophoresis were products of Sigma-Aldrich (St Louis, MO) and Bio-Rad Laboratories (Hercules, CA). SP-Sepharose FF, Sephacryl S-100, Macro-Cap-SP, and ampholyte were purchased from Amersham Pharmacia Biotech (Piscataway, NJ). (mPEG)2-Lys-NHS (20 kDa) was obtained from Shearwater Polymers (Huntsville, AL). Dulbecco’s Modified Eagle’s Medium (DMEM) and fetal bovine serum used in cell culture were from Gibco BRL (Grand Island, NE). pUC18 DNA was purchased from TAKARA (Dalian, China). Nitrocellulose (NC) membrane was obtained from Bio-Rad Laboratories. Sheep-antimouse Ab-linked to alkaline phosphatase was purchased from Sigma-Aldrich. JAR choriocarcinoma cells were purchased from the Cell Bank of Shanghai Institute of Cell Biology (Shanghai, China).
Purification of α-MMC and MAP30
All steps tried either alone or in combination within the process of purification were carried out at 4–6°C unless specifically stated. Firstly, the powder from fresh bitter melon seeds was extracted in 0.15 M NaCl solution and then the pH of the solution was adjusted to 4.0. After simple centrifugation, the supernatant was neutralized and fractionated by 30%–65% ammonium sulfate. The precipitate was dialyzed against the pH 6.3, 0.05 M phosphate buffer. Secondly, the sample was applied onto a SP-Sepharose FF column and eluted with pH 6.3, 0.05 M phosphate buffer containing 0.15 M NaCl. The elution peak containing 30 kDa protein was collected. Thirdly, the portion was loaded onto a Sephacryl S-100 column and the elution peak with 30 kDa protein was pooled. Finally, the sample was applied onto a Macro-Cap-SP column. A linear gradient of 0–0.15 M NaCl in pH 7.0, 20 mM sodium phosphate buffer eluted the column and two peaks with 30 kDa proteins were respectively collected.
Determination of protein concentration
Protein concentration was determined by the method of Lowry et alCitation14 or ultraviolet spectrometry at 280 nm using bovine serum albumin (BSA) as standard.
Identification of α-MMC and MAP30
SDS-PAGE
SDS-PAGE was performed according to Laemmli.Citation15
Assay of the amino-terminal sequence ESI-QUAD-MS and MALDI-TOF-MS
Both the amino-terminal sequences MALDI-TOF-MS and electrospray ionization quadruple mass spectrometry (ESI-QUAD-MS) were consigned to the Beijing Proteome Research Center of China to complete the analysis.
Western blotting analysis
Briefly, α-MMC was separated on SDS-PAGE and then transferred to an NC membrane. Nonspecific binding was blocked and washed by placing the membrane in a solution of BSA. NC membrane was incubated with a mouse anti-α-MMC McAb and was exposed to a sheep-antimouse Ab linked to alkaline phosphatase. After adding BCIP (5-bromo-4-chloro-3-indolyl phosphate), the colored bands were visualized and photographed.Citation16 In the analysis of MAP30, NC membrane was incubated with a diluted solution of rat anti-MAP30 PcAb under gentle agitation. Other conditions were the same with α-MMC.
Chemical synthesis and identification of PEGylated α-MMC and PEGylated MAP30
In general, PEGylated α-MMC and PEGylated MAP30 can be obtained in the following optimal conditions: 10 mg/mL of α-MMC or MAP30 reacted with (mPEG)2-Lys-NHS (mass ratio of PEG:RIP was 2:1) in pH 8.5,100 mM borate buffer at room temperature for 30 minutes. The reaction mixture was applied with Sephacryl S-100 column. The PEGylated conjugates can be collected and assessed by gradient SDS-PAGE and MALDI-TOF-MS.
Topological inactivation activity
Topological inactivation activity was according to a previously described method.Citation17
Antitumor activity in vitro
JAR choriocarcinoma cells were maintained in an incubator supplied with a humidified atmosphere of 5% CO2 at 37°C. The culture medium was DMEM containing 20 mM HEPES and 10% fetal bovine serum. The cell viability and proliferation were determined by quantitative 3-(4,5-dimethylthiozol-2-yl)-2,5- diphenyltetrazolium bromide (MTT). A total of 3 × 104 cells/mL were applied into 96-well microtiter plates at 100 μL per well and exposed to PEGylated α-MMC, PEGylated MAP30, and un-PEGylated counterparts of 0.012, 0.06, 0.3, and 1.5 mg/mL at 24, 48, and 72 hours. Cells without drugs were used as controls. Each concentration was tested in quadruplicate. The determination procedure was carried out according to the previous description.Citation18 The optical density (OD) at 570 nm was measured using an ELISA plate reader (EL × 800, BIO-TEK, Atlanta, GA). Cell viability and proliferation were observed together with controls.
The inhibitory effect of PEGylated RIPs on herpes simplex virus-1
VERO cells were cultured to logarithmic growth phase in 10 mL DMEM containing 10% (v/v) fetal bovine serum, 100 μg/mL penicillin, and 100 U/mL streptomycin. After removing medium, cells were substituted to 5 mL of the above medium and infected with 100 μL of herpes simplex virus-1 (HSV-1) for 1.5 hours. Then, 5 mL maintenance medium containing 2% (v/v) fetal bovine serum, 100 μg/mL penicillin, and 100 U/mL streptomycin was added and cells were continually cultured for about 1–2 days when over 80% cells appeared with cytopathic effect (CPE). The infected supernatant was collected for testing of virus titers.
Determination of virus titer
VERO cells of 1 × 105 cells/mL were applied 100 μL per well to 96-well microtiter plates and cultured for 24 hours. After discarding medium, 100 μL of infected supernatant, which was 10−1 to 10−10 diluted with medium, was added to each well (quadruplicate) until CPE did not increase. TCID50 (50 percent tissue culture infective dose) was calculated according to Reed and Muench.Citation19,Citation20
Measurement of cytotoxicity
The cytotoxicity of PEGylated α-MMC and PEGylated MAP30 was evaluated by the quantitative MTT test.Citation21 A total of 1 × 105 VERO cells/mL was applied to 96-well microtiter plates at 100 μL per well and was exposed to un-PEGylated proteins and PEGylated-proteins at 3.3 μmol · L−1~3.3 nmol · L−1 (100 mg · L−1~0.1 mg · L−1) and acyclovir (ACV) at 10 μmol · L−1~0.01 μmol · L−1 for 48 hours as well as cells without drugs as control. Each concentration was tested in quadruplicate. The determination procedure was carried out according to the previous description.Citation18 The OD at 490 nm was measured using ELISA:
Inhibition of proteins on HSV-1 antigen secretion
A total of 1 × 105 VERO cells/mL were seeded into 24-well plates at 1 mL/well and then incubated with 5% CO2 at 37°C for 24 hours. After abandoning the supernatant, 300 μL HSV-1 stock solution with 100 TCID50/mL was added to each well and incubated for 1.5 hours. Then, 100 μL of culture medium containing proteins (concentration was mentioned previously in the cytotoxity method) was added to each well and negative and positive cell control established. After incubation for 48 hours, HSV-1 antigen in culture supernatant was tested by human HSV-1 antigen 1 (HSV-1 AG1) enzyme-linked immunosorbent assay kit with indirect ELISA:
The analysis of virus inactivation
VERO cells infected with HSV-1 were added with 20 μL (5 mg/mL) of MTT to each well and incubated at 37°C for 4 hours. Then, 150 μL of DMSO was added to each well. The OD490 was read by a microplate spectrophotometer. The percentage of inhibition was calculated by the formula described under the heading “Measurement of cytotoxicity.”
Immunogenicity of PEGylated α-MMC and PEGylated MAP30
Fifty Sprague Dawley rats were randomly classified into control, α-MMC, PEGylated α-MMC, MAP30, and PEGylated MAP30 groups of ten each. The rats in the control group were subcutaneously injected with saline solution, and the RIP and PEGylated RIP groups were emulsified in Freund’s complete adjuvant at a dose of 1.42 mg/kg at the infection frequency of 3 days for 17 days. Blood samples were collected from the capillary vessel in rats’ eyes and separated sera were stored at −20°C. Antigen-specific serum IgG levels were measured by ELISA. Briefly, 96-well plates were coated with 100 μL of 30 μg/mL purified antigen (RIPs and PEG-RIPs) in 0.05 M carbonate-coating buffer, pH 9.6, and incubated at 4°C. After washing, 300 μL of PBS containing 3% BSA, pH 7.4, was added to each well, followed by 2.5 hours of incubation at 37°C. Diluted sera (1:16) were added (100 μL/well) and followed by 2.5 hours of incubation at 37°C. Wells were then washed in PBS washing buffer, pH 7.4, containing 0.05% Tween-20, and incubated with peroxidase-conjugated AffiniPure goat antimouse IgG (1:16000) at 37°C for 2.5 hours. Then, the plate was washed five times in PBS followed by the addition of 100 μL/well tetramethyl benzidine (Sigma-Aldrich) as a substrate for horseradish peroxidase. The reactions were allowed to develop at 37°C for 15 minutes. Finally, 50 μL of 2 M sulfuric acid was added to stop the reaction. The absorbance was measured with a plate reader (Model 680, Bio-Rad Laboratories) at 450 nm.
Statistical analysis
SPSS statistical software (SPSS, Inc, Chicago, IL) was used for analysis. P < 0.05 was considered statistically significant.
Results and discussion
Purification and identification of α-MMC and MAP30
Purification of α-MMC and MAP30 was performed by applying 30%–65% ammonium sulfate precipitation, acidification, SP-Sepharose FF, Sephacryl S-100, and Macro-Cap-SP chromatography. In conclusion, 200 mg of α-MMC and 120 mg of MAP30 with the recoveries of 1.7% and 1.0%, respectively, was obtained from 200 g starting material seeds. The procedure of purification is summarized in . Both α-MMC and MAP30 were assessed by SDS-PAGE () and Western blotting (). By using the Compute pI/Mw program from ExPASy Proteomics Server (http://www.expasy.org), the theoretical pI of α-MMC is 9.32 and MAP30 is 8.86. And this is coincident with the result of IEF analyses of these two proteins. ESI-QUAD-MS was used to further identify the two proteins, and sequences were analyzed using the BLAST program (http://www.expasy.org/tools/blast/), which demonstrated that they are α-MMC (Swiss Prot accession number: P16094, peptides are YLLMHLFNYDGK) and MAP30 (Swiss-Prot accession number: P24817, peptides are ESPPEAYNILFK). MALDI-TOF-MS was used to determine the accurate molecular weight of α-MMC and MAP30. The results showed that the molecular weights were 28551.6 Da and 29072.0 Da, respectively (), while the theoretical molecular weight was 27368.36 Da and 29600.74 Da (http://www.expasy.org). Edman degradation indicated that the N-terminal sequence of the purified proteins, N-Asp-Val-Ser-Phe-Arg and N-Asp-Val-Asn-Phe-Asp, was consistent with theoretical sequences of both α-MMC and MAP30.
Table 1 Summarization of purification of alpha-momorcharin (α-MMC) and momordica anti-HIV protein (MAP30) from 200 g of bitter melon seeds
Figure 1 SDS-PAGE of extracts and eluates from different purification steps. Lane 1: crude extract; Lane 2: dialytic sample after AS precipitation; Lane 3: eluate from SP-Sepharose FF chromatography; Lane 4: eluate from gel filtration chromatography; Lane 5: α-MMC from Macro-Cap-SP chromatography; Lane 6: MAP30 from Macro-Cap-SP chromatography; Lane7: LMW calibration kit.
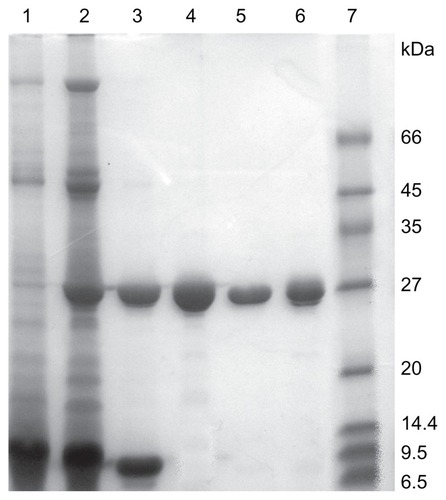
Purification and identification of PEGylated α-MMC and PEGylated MAP30
Through optimized PEGylation reaction, a high total PEGylation ratio can be obtained (about 60%–70%). The gradient SDS-PAGE monitoring () showed that the un-PEGylated counterparts from the reaction mixture were successfully removed by Sephacryl S-100 chromatography or Superdex 75 chromatography. In the MALDI-TOF-MS analysis (), one-mer, two-mer, and three-mer PEGylated isomers with 49899.4 Da, 70430.9 Da, and 91057.6 Da (the molar ratios of 1:1, 2:1, and 3:1 of (mPEG)2-Lys-NHS:α-MMC) were detected from α-MMC-PEG conjugates. Meanwhile, one-mer and two-mer PEGylated isomers with 50335.6 Da and 70614.2 Da (the molar ratios of 1:1 and 2:1 of (mPEG)2-Lys-NHS: MAP30) were found from MAP30-PEG conjugates.
Figure 4 Gradient SDS-PAGE of both PEGylated α-MMC and PEGylated MAP30 conjugates. (A) Lane 1: α-MMC as a control; Lane 2: mixture of α-MMC and α-MMC-PEG conjugate; Lane 3: purified α-MMC-PEG conjugate; Lane 4: unmodified α-MMC; Lane 5: HMW calibration kit. (B) Lane 1: MAP30 as a control; Lane 2: mixture of MAP30 and MAP30-PEG conjugate; Lane 3: purified MAP30-PEG conjugate; Lane 4: unmodified MAP30; Lane 5: HMW calibration kit.
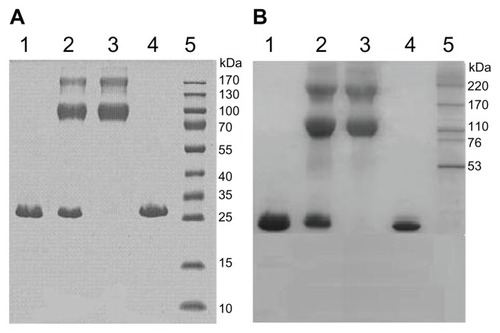
Figure 5 MALDI-TOF-MS analysis of both PEGylated α-MMC and PEGylated MAP30 conjugates. (A) The (mPEG)2-Lys-NHS was around 20 kDa and the PEG conjugates were observed at 49899.4 Da, 70430.9 Da and 91057.6 Da, reflecting 1, 2 or 3 PEG-bound chains per α-MMC molecule. (B) The (mPEG)2-Lys-NHS was around 20 kDa and the PEG conjugates were observed at 50335.6 Da and 70614.2 Da, reflecting 1 or 2 PEG-bound chains per MAP30 molecule.
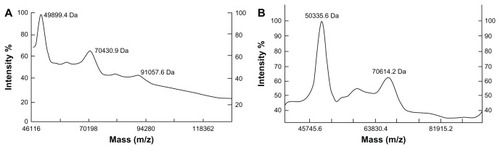
Topological inactivation activity
To demonstrate their topological inactivation activity, supercoiled DNA (pUC18) was incubated with PEGylated RIPs and un-PEGylated counterparts. In suitable enzymatic digestion conditions, all of the experiment samples cleaved the supercoiled double-stranded DNA to produce nicked circular or linear DNA. As shown in , all of them exhibited DNase-like activity.
Effects of PEGylated α-MMC and PEGylated MAP30 on proliferation of JAR cells
To investigate the effect of PEGylated α-MMC and PEGylated MAP30 on cell viability and proliferation, JAR cells were seeded on 96-well plates and were treated with increasing concentrations of PEGylated RIPs and un-PEGylated counterparts for 72 hours () and for different times at 1.5 mg/mL (). Statistical analysis revealed that the concentrations of 1.5, 0.3, and 0.06 mg/mL started to significantly reduce the proliferation of cells after 48 and 72 hours of incubation. The analyses with the result of exhibited that native α-MMC allowed an approximately 21% decrease compared with the PEGylated α-MMC and 25% of MAP30 with the PEGylated MAP30 at the highest tested concentration. This can indirectly demonstrate a dose- and time-dependent inhibition to the growth of JAR choriocarcinoma cells. The result in the analysis of displayed that the growth of cells was not prominent after 24 hours of treatment, but continued incubation for 48 or 72 hours with the proteins enhanced the cytotoxicity on cells.
Figure 7 Inhibitory effects of α-MMC/MAP30 and PEG-α-MMC/MAP30 on the proliferation of human choriocarcinoma JAR cells. JAR cells were treated with increasing concentrations for 72 hours. (A) and for various time points at 1.5 mg/mL. (B) of native or PEGylated α-MMC/MAP30 as indicated.
Note: Each data point represents the average of three independent experiments performed in quadruplicate.

α-MMC and PEGylated α-MMC induced alterations in the morphology of JAR cells (). After processing for 72 hours, untreated JAR cells extended and flattened (), while the treated groups showed fewer cells and abnormal shapes such as shrinkage, blebbing, and loss of membrane asymmetry, indicating the cytotoxic effect of α-MMC and its modifier on JAR cells. In particular, α-MMC-treated groups showed extremely obvious morphological changes (). MAP30 and PEGylated MAP30 also showed a similar cytotoxic effect to JAR cells.
The inhibitory effect of PEGylated α-MMC and PEGylated MAP30 on HSV-1
TCID50 was calculated using the method of Reed and Muench,Citation19 and the emergence of CPE was used as a positive sign. The CPE of each dilution of HSV-1 cytopathic was tabulated as in . Measurement of cytotoxicity was designed to establish appropriate dose range for the research of inhibitory effect of proteins on HSV-1 to VERO cells. The result () reflected the effects of different concentrations of test proteins on cell viability of VERO cells and showed that α-MMC/MAP30, PEG-α-MMC/MAP30, and ACV had no significant inhibition on VERO cells in the tested concentration range. The cell viability maintained above 97% from 0.003–0.03 μmol · L−1 and 85% from 0.3–3.3 μmol · L−1. Therefore, the dose range of tested proteins inhibiting VERO cell infection with virus was below 3.3 μmol · L−1. The inhibitory effect of α-MMC/MAP30, PEGylated α-MMC/MAP30, and ACV on HSV-1 glycoprotein antigen secretion glycoprotein was tested through the determination of HSV-1 glycoprotein antigen (). The results showed a dose-dependent inhibitory effect. IC50 was 0.67 μmol · L−1 with α-MMC, 2.94 μmol · L−1 with PEG-α-MMC, 0.47 μmol · L−1 with MAP30, 2.39 μmol · L−1 with PEG-MAP30, and 3.55 μmol · L−1 with ACV, respectively. Furthermore, IC50 of α-MMC/MAP30 and PEG-α-MMC/MAP30 was lower than the general drug of ACV. At the same dose, the inhibitory effect on HSV-1 glycoprotein antigen secretion, glycoprotein of MAP30 was higher than that of α-MMC and PEG-MAP30 was higher than PEG-α-MMC. The MTT results showed that the ratio of cell viability can be increased on a dose-dependent feature compared with the control group when protein concentration was higher than 0.03 μmol · L−1. But ACV did not display effects on HSV-1 inactivation. At the same dose, the ratio of cell viability with MAP30 was higher than α-MMC and PEG-MAP30 was higher than PEG-α-MMC. This indicated that both α-MMC/MAP30 and PEG-α-MMC/MAP30 had the ability of direct HSV-1 inactivation in a certain range of concentration. Additionally, the effect of inactivation was obvious with the increase of protein concentration. VERO cells infected with HSV-1 after 48 hours displayed alterations in the morphology. The inhibitory effect can be observed in groups of α-MMC/MAP30, PEG-α-MMC/MAP30, and ACV (). The extent of cytopathy was gradually reduced with the increase of the protein concentration. In groups of α-MMC and MAP30, most cells did not appear cytopathic caused by virus at a concentration of 3.3 μmol · L−1. This indicated that proteins played an important role in the inhibition on HSV-1.
Table 2 Herpes simplex virus-1-induced cytopathic effect (CPE) of VERO cell (50% tissue culture infective dose (TCID50) = antilog [(55.6–50)/55.6–20) × (−1) + (lg10−5)] = 10−5.2/0.1 mL = 10−6.2/mL)
Table 3 Inhibitory effects of RIPs and PEGylated RIPs on herpes simplex virus-1 (HSV-1) antigen
Evaluation of immunogenicity of both PEGylated MAP30 and PEGylated α-MMC in Sprague Dawley rats
The antigenicity of native and two PEGylated RIPs was evaluated by measuring the specific IgG antibody titers of the serum of Sprague Dasley rats. The results showed that the PEGylated RIPs allowed to some extent decrease of immunogenicity while compared with un-PEGylated counterparts. The decrease of IgG generation was approximately 70% for PEGylated α-MMC compared with the un-PEGylated counterpart and 50% for PEGylated MAP30 compared with the un-PEGylated counterpart.
Conclusion
A novel preparative strategy of both α-MMC and MAP30 at one time was successfully established. Some properties of these proteins were assessed by SDS-PAGE, ESI-QUAD-MS, MALDI-TOF-MS, and N-terminal sequence as well as Western blotting. This fast, highly efficient methodology enables us to focus more energy on subsequent research. A branched (mPEG)2-Lys-NHS(20 kDa) was used to modify these proteins and the serial PEGylated ones were assessed by gradient SDS-PAGE and MALDI-TOF-MS. The α-MMC modifier possessed one-mer, two-mer, and three-mer PEG-bound chains, while MAP30 modifier obtained one-mer and two-mer PEG-bound chains. PEGylated conjugates preserved moderate activities on JAR choriocarcinoma cells and HSV-1. Furthermore, both PEGylated proteins showed about 60%–70% antitumor and antivirus activities as well as a 50%–70% immunogenicity decrease when compared with unmodified counterparts. To sum up, PEGylation of α-MMC and MAP30 may offer a possible way for their clinical application as potential therapeutic agents. Nevertheless, to fulfill the requirements of a useful drug, we should meet the challenges, including the reduction of immunogenicity to the greatest extent, the retention of sufficient activity, the extension of the half-life period, and development of various forms of PEG-RIPs. This work is just the beginning for them to be used clinically. After all, therapeutic proteins must fulfill the requirements described previously. PEGylation can reduce immunogenicity and retain certain biological activity of RIPs, but there is lots of work to do for further decreasing their immunogenicity and extending the half-life period. Future work concerns packing RIPs with nanomaterials for targeting drugs and low release.
Acknowledgments
This work was supported by the National Science Foundation of China (Grant No 30770232).
Disclosure
The authors report no conflicts of interest in this work.
References
- ReadBELiuJLiS1956Pen ts’ao kang mu [Chinese pharmaceutical compendium]Beijing, ChinaPeople’s Medical Publishing House1977
- StirpeFRibosome-inactivating proteinsToxicon200444437138315302521
- HausnerTPAtmadjaJNierhausKHEvidence that the G2661 region of 23S rRNA is located at the ribosomal binding sites of both elongation factorsBiochimie19876999119233126829
- BourinbaiarASLee-HuangSThe activity of plant-derived antiretroviral proteins MAP30 and GAP31 against herpes simplex virus in vitroBiochem Biophys Res Commun199621939239298645280
- Foà-TomasiLCampadelli-FiumeGBarbieriLStirpeFEffect of ribosome-inactivating proteins on virus-infected cells. Inhibition of virus multiplication and of protein synthesisArch Virol19827143233326284092
- McGrathMSHwangKMCaldwellSEGLQ223: an inhibitor of human immunodeficiency virus replication in acutely and chronically infected cells of lymphocyteand mononuclea phagocyte lineageProc Natl Acad Sci U S A1989868284428482704750
- HuangPLSunYChenHCKungHFLee-HuangSProteolytic fragments of anti-HIV and anti-tumor proteins MAP30 and GAP31 are biologically activeBiochem Biophys Res Commun1999262361562310471373
- KozlowskiAHarrisJMImprovements in protein PEGylation: pegylated interferons for treatment of hepatitis CJ Control Release2001721–321722411390000
- HarrisJMChessRBEffect of pegylation on pharmaceuticalsNat Rev Drug Discov20032321422112612647
- RobertsMJBentleyMDHarrisJMChemistry for peptide and protein PEGylationAdv Drug Deliv Rev200254445947612052709
- LordJMHartleyMRRobertsLMRibosome inactivating proteins of plantsSemin Cell Biol19912115221954339
- Lee-HuangSHuangPLChenHCAnti-HIV and anti-tumor activities of recombinant MAP30 from bitter melonGene199516121511567665070
- Lee-HuangSHuangPLNaraPLMAP30: a new inhibitor of HIV-1 infection and replicationFEBS Lett19902721–212181699801
- LowryOHRosebroughNJFarrALRandallRJProtein measurement with the Folin phenol reagentJ Biol Chem1951193126527514907713
- LaemmliUKCleavage of structural proteins during the assembly of the head of bacteriophage T4Nature197022752596806855432063
- NgTBShawPCYeungHWHoWKImmunological relatedness of ribosome-inactivating proteins from the Cucurbitaceae familyBiochem Mol Biol Int19933134474538118419
- Lee-HuangSHuangPLSunYLysozyme and RNases as anti-HIV components in beta-core preparations of human chorionic gonadotropinProc Natl Acad Sci U S A19999662678268110077570
- LiMChenYLiuZShenFBianXMengYAnti-tumor activity and immunological modification of ribosome-inactivating protein (RIP) from Momordica charantia bycovalent attachment of polyethylene glycolActa Biochim Biophys Sin (Shanghai)200941979279919727528
- ReedLMuenchHA simple method of estimating fifty per cent endpointsAm J Epidemiol1938273493
- YangZYKongWPHuangYA DNA vaccine induces SARS coronavirus neutralization and protective immunity in miceNature2004428698256156415024391
- MosmannTRapid colorimetric assay for cellular growth and survival: application to proliferation and cytotoxicity assaysJ Immunol Methods1983651–255636606682