Abstract
As a non-invasive strategy, sonodynamic therapy (SDT) which utilizes sonosensitizers to generate reactive oxygen species (ROS) has received significant interest over recent years due to its ability to break depth barrier. However, intrinsic limitations of traditional sonosensitizers hinder the widespread application of SDT. With the development of nanotechnology, various nanoparticles (NPs) have been designed and used to assist sonosensitizers for SDT. This review first summarizes the possible mechanisms of SDT, then classifies the NPs-assisted sonosensitizers and discusses their biomedical applications in ultrasonography, drug delivery, high intensity focused ultrasound and SDT-based combination treatment. Finally, some challenges and future perspectives of NPs-assisted SDT has also been discussed.
Introduction
Non-invasive therapies have received increasing attention because of improved therapeutic efficacy, decreased side effects and excellent spatial/temporal resolution.Citation1,Citation2 As a typical representative, photodynamic therapy (PDT), which utilizes photosensitizers to generate reactive oxygen species (ROS) for tumor cell apoptosis or necrosis, has been developed as a clinically therapeutic modality for superficial skin carcinoma.Citation3 However, the major challenge for PDT is the low penetration of light, which limits the application of PDT in deep tumor.Citation3
Ultrasound (US), as a mechanical wave with a frequency higher than human hearing (>20 kHz), has many characteristics including deep tissue penetration, non-invasion and non-ionization.Citation4 Therefore, US has been developed for many biomedical applications such as ultrasonography, sonodynamic therapy (SDT), drug delivery, high intensity focused ultrasound (HIFU) and so on.Citation5 As an emerging treatment mode, SDT utilizes US to activate sonosensitizers for ROS production, which could overcome the depth limitation.Citation6 However, traditional organic sonosensitizers suffer from poor pharmacokinetics and tumor accumulation, which limit the efficacy of SDT.Citation7 Promoted by the rapid development of nanomedicine, various NPs have been developed to assist sonosensitizers for improving the efficacy of SDT.Citation8–Citation10
In this review, we have classified and discussed the NPs-assisted sonosensitizers, summarized their mechanisms and biomedical applications in SDT, imaging, drug delivery and HIFU. Furthermore, we highlighted the recent advances of SDT-based combination therapy. Finally, we discussed the challenges and future perspectives of NPs-assisted SDT.
Mechanisms of Sonodynamic Therapy
Although extensive evidencess have demonstrated the therapeutic efficiency of SDT, the exact mechanism still remains unclear because of the complicated SDT processes.Citation8 Possible mechanisms could be summed up as generation of ROS, mechanical effects, and thermal effects.Citation8,Citation11–Citation13
Generation of Reactive Oxygen Species
Up to now, the most recognized mechanism is the production of ROS induced by sonosensitizers during the US.Citation8 After absorbing the energy from US, the sonosensitizers could be activated to produce ROS including singlet oxygen (1O2), hydroxyl radicals (•OH), superoxide anion (O2•−) or hydrogen peroxide (H2O2).Citation5,Citation14 Different kinds of ROS are generated with the selection of different sonosensitizers. For example, hematoporphyrin could produce singlet oxygen to kill the tumor cells.Citation15 Meanwhile, titanium oxide TiO2 NPs and bimetallic oxide MnWOx NPs are reported to generate both singlet oxygen and hydroxyl radicals for enhanced SDT efficiency.Citation16,Citation17
Mechanical Effects
Mechanical effects mainly include the cavitation effect and sonoporation effect.Citation11,Citation13 Cavitation could be divided into stable cavitation and inertial cavitation according to how the bubbles collapse during US.Citation18 Under low sound pressure (<0.1 MPa), bubbles usually display stable cavitation (SC), which is a periodic shrink and expansion of gas bubbles.Citation11,Citation13 Once the sound pressure is sufficiently high, the bubbles will collapse instantly, which is called as inertial cavitation (IC).Citation11 IC could produce acoustic emissions,Citation19 microstreaming,Citation19 jetting,Citation20 and shockwaves,Citation19 which lead to mechanical damage.Citation21 In addition, through thermal dissociation of water, the cavitation effect would also produce ROS.Citation8
Sonoporation effect refers to formation of pores in the cell membrane under US irradiation.Citation13 These formed pores allow for the transfer of molecules and NPs into cells.Citation22 Thereby, sonoporation effect could be utilized to increase the uptake and accumulation of drug molecules, genes or NPs.Citation13
Thermal Effects
Thermal effects are the rise of tissue temperature due to the absorption of US energy and converted into thermal energy.Citation23 The thermal conversion efficiency is connected with hemoperfusion and protein level.Citation11 For example, Barnett et al reported that the thermal conversion efficiency is positively related to protein level.Citation24 In addition, tissues with poor hemoperfusion could be dramatically heated under US irradiation due to the slow heat dissipation.Citation24
Nanoparticles Used for Sonosensitizers
During the US process, ROS could be generated. However, the amount of produced ROS is not enough to exert treatment effect due to the limited generating rate and nonspecific distribution.Citation5 Hence, the addition of sonosensitizers is necessary to ensure adequate production of ROS in tumor tissues. Benefiting from the development of nanotechnology, a mass of sonosensitizers-based nanocarriers have been constructed to improve the therapeutic effect of SDT.Citation7 Those NPs could be broadly divided into organic NPs and inorganic NPs.
Organic Nanoparticles
The early used sonosensitizers in SDT are organic small molecules, which are inspired from photosensitizers for photodynamic therapy.Citation25 Similar to photosensitizers, the first generation of sonosensitizers are based on porphyrin derivatives, including hematoporphyrin monomethyl ether (HMME), protoporphyrin IX (PpIX) hematoporphyrin (Hp), photofrin and so on.Citation26 Furthermore, many other organic molecules have also been identified as sonosensitizers, such as chlorophyll, hypocrellin B (HB), curcumin and their derivatives.Citation27–Citation34 However, the nonspecific distribution and poor pharmacokinetic property of organic small molecules have hindered their further clinical translation. These problems could be solved in a certain extent through loading the small molecules into organic NPs. Common organic NPs are including liposomes and self-assembled organic NPs.
Consisting of multiple phospholipids, liposomes are widely used for the sonosensitizer delivery because of their good biocompatibility and biodegradability. For example, porphyrin analogue (purpurin 18), as a sonosensitizer, was loaded into liposomes for the SDT of bacterial infections ().Citation35 Moreover, self-assembled organic NPs are also developed for the sonosensitizer encapsulation. For example, Zhai’s group constructed chondroitin sulfate-adipic dihydrazide-chlorin e6-lipoic acid (CS-ADH-Ce6-LA) self-assemble NPs to load with docetaxel (DTX), named as DTX/X-NPs ().Citation36 Under low-intensity US irradiation, chemo-sonodynamic combination therapy can be achieved through these DTX/X-NPs.
Figure 1 (A) Scheme illustration of purpurin 18 loaded liposomes for diagnosis and therapy of bacterial infection. Reprinted with permission from ACS Nano. Pang X, Xiao Q, Cheng Y et al. Bacteria-responsive nanoliposomes as smart sonotheranostics for multidrug resistant bacterial infections, pages 2427–2438. Copyright 2019 American Chemical Society.Citation35 (B) Illustration of the preparation, tumor accumulation, drug release and immune response mechanism of DTX/X-NPs. Reprinted from Journal of Controlled Release. Liu M, Khan A. R, Ji J et al. Crosslinked self-assembled nanoparticles for chemo-sonodynamic combination therapy favoring antitumor, antimetastasis management and immune responses, pages 150–164. Copyright 2018, with permission from Elsevier.Citation36
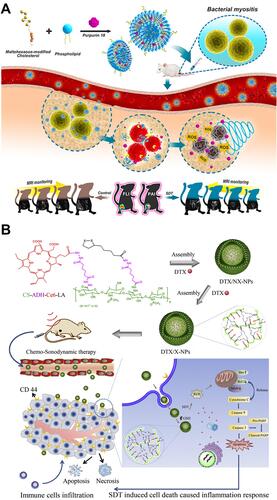
Inorganic Nanoparticles
Inorganic sonosensitizers are also used to improve the efficacy of SDT, as they are usually made up of inorganic NPs. Compared with organic NPs, inorganic NPs exhibit superior chemical/physiological properties and multifunctionality.Citation7 Common inorganic sonosensitizers are including titanium dioxide (TiO2) NPs, noble metal NPs, carbon-based and silica-based NPs.
TiO2 Nanoparticles
Under US irradiation, TiO2 NPs can produce electron−hole pairs for ROS generation.Citation37 However, the limited ROS generating rate due to the rapid electron−hole recombination and low stability in physiological conditions has hindered the application of TiO2 NPs in SDT.Citation38 Through surface functionalization, these two challenges could be alleviated.
In order to improve the ROS-generating efficiency, functional materials could be integrated to the surface of TiO2 NPs. For example, Deepagan et al reported hydrophilized Au-TiO2 nano-composites (HAu-TiO2 NCs) as sonosensitizers to increase the yield of ROS ().Citation38 Compared with HTiO2 NPs, HAu-TiO2 NCs were able to produce more ROS, which enhanced the efficacy of SDT.
Figure 2 (A) Schematic illustration of passive accumulation and ROS generation by HAu-TiO2 NCs. Reprinted with permission from Nano letters. Deepagan VG, You DG, Um W et al. Long-circulating Au-TiO2 nanocomposite as a sonosensitizer for ROS-mediated eradication of cancer, 6257–6264. Copyright 2016, American Chemical Society.Citation38 (B) Schematic illustration of SDT induced by HTiO2 NPs. Reprinted from Scientific Reports. You DG, Deepagan VG, Um W et al. ROS-generating TiO2 nanoparticles for non-invasive sonodynamic therapy of cancer, article number 23200. Copyright 2016, with permission from Nature Publishing Group.Citation39
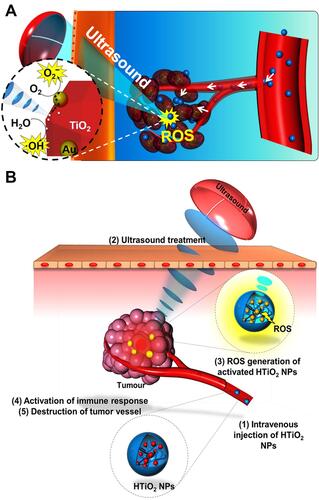
For improving the stability, some molecules could be anchored on the surface of TiO2 NPs. As a typical paradigm, You et al used hydrophilic carboxymethyl dextran (CMD) to modify the surface of TiO2 NPs ().Citation39 After CMD coating, the stability and blood circulation time of TiO2 NPs were increased.
Noble Metal Nanoparticles
Through preventing electron−hole recombination and increasing ROS generation, some noble metal materials like Au, Ag and Cu can be used to improve the efficacy of SDT.Citation8 Au NPs are eminently suitable for sonosensitizers due to the high biocompatibility and easy surface modification.Citation40,Citation41 For example, Courrol et al reported gold NPs functionalized with polyethylene glycol (PEG) as sonosensitizers for SDT.Citation42 In addition, some other noble metal NPs could also enhance SDT. As a typical paradigm, Bernard et al developed AgCu bimetallic NPs to combine with US.Citation43 In vitro experimental results demonstrated that these bimetallic NPs could improve the SDT and significantly decrease the cell viability of human ovarian carcinoma cell A2780.
Carbon-Based and Silica-Based Nanoparticles
Carbon-based NPs such as fullerene and graphene have been developed for sonosensitizers because they can separate electrons and holes.Citation8 For example, Yumita et al investigated the US-induced antitumor effect of polyhydroxy fullerene (PHF) and proved that PHF is a potential sonosensitizer for SDT.Citation44
Moreover, because the ability of absorbing US energy and inducing hyperthermia, silica-based NPs are also suitable for sonosensitizers.Citation45,Citation46 For example, Osminkina et al prepared porous silicon NPs covered by dextran.Citation45 Upon US irradiation (1–3 MHz, 1–2 W/cm2), these silicon NPs could inhibit the tumor growth both in vitro and in vivo through inducing hyperthermia.
It is worth noting that the collapse of bubbles (namely inertial cavitation) can also generate ROS, even without the assistance of sonosensitizers. Therefore, thorough constructing gas-generating NPs and producing specific gas bubbles (such as O2, CO2, NO), the efficacy of SDT can also be improved.Citation47
Biomedical Applications of Nanoparticles Assisted Sonosensitizers
Characterized by non-invasive and deep penetrative nature, US has been widely used in tumor diagnosis and treatment,Citation5,Citation8 such as imaging,Citation48 drug delivery,Citation4,Citation49 HIFUCitation50 and SDT-based synergistic therapy.Citation7–Citation9
Ultrasound Imaging
Effective ultrasonic imaging needs the particles size in the micron range, which are too large to penetrate through the intervals of vascular endothelial cells to cancer cells.Citation51,Citation52 To solve this size challenge, many phase-changeable NPs have been designed, which could accumulate in tumor site through enhanced permeability and retention effect (EPR) and then generate micrometer-sized bubbles by phase-change.Citation53
The most common phase-changeable NPs are using NPs to load with liquid fluorocarbons.Citation11 Under US irradiation, the liquid fluorocarbon changes to gas phase and microbubbles are formed, leading to enhanced ultrasonography. As a typical paradigm, Kim et al fabricated echogenic glycol chitosan NPs (named as Echo-CNPs) to encapsulate perfluoropentane (PFP) and anticancer drug ().Citation54 Through US irradiation (10 MHz, 0.0676 W/cm2), liquid-phase PFP transformed to microbubbles and anticancer drugs were also released, which resulted in cancer theranostics.
Figure 3 (A) Schematic illustration of Echo-CNPs. Reprinted from Theranostics. Min HS, You DG, Son S et al. Echogenic glycol chitosan nanoparticles for ultrasound-triggered cancer. Theranostics. 2015;5:1402–1418. Copyright 2015, with permission from Ivyspring International Publisher.Citation54 (B) Schematic illustration of GNR@SiO2-PFP for US/PA dual-modality imaging. Reprinted from Advanced Materials. Li C, Zhang Y, Li Z et al. Light-responsive biodegradable nanorattles for cancer theranostics. © 2017 WILEY-VCH Verlag GmbH & Co. KGaA, Weinheim.Citation55 (C) Schematic illustration of PFH/DOX@PLGA/Fe3O4-FA NPs for US/MR dual-modality imaging and chemotherapy/HIFU synergistic therapy. Reprinted with permission from ACS Applied Materials and Interfaces. Tang H, Guo Y, Peng L et al. In vivo targeted, responsive, and synergistic cancer nanotheranostics by magnetic resonance imaging-guided synergistic high-intensity focused ultrasound ablation and chemotherapy, pages 15428–15441. Copyright 2018 American Chemical Society.Citation56
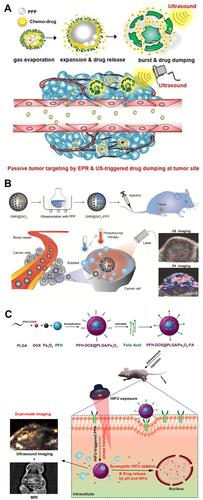
Furthermore, US imaging can be used to combine with other imaging modalities, such as photoacoustic imaging (PAI) and magnetic resonance imaging (MRI). For example, Huang et al designed a mesoporous silica coated gold nanorod to fill with PFP (GNR@SiO2-PFP), which could enhance US/PA dual-modality imaging ().Citation55 In another work, Chen’s group reported targeted theranostic NPs consisting of folic acid (FA), poly(lactic-co-glycolic acid) (PLGA), Fe3O4 NPs, perfluorohexane (PFH) and doxorubicin (DOX) (PFH/DOX@PLGA/Fe3O4-FA), which were able to achieve US/MR dual-modality imaging and chemotherapy/HIFU synergistic therapy ().Citation56
Ultrasound-Triggered Drug Delivery
Because of the sonoporation effect which can form pores in the cell membrane thus increased the drug transport, US has been widely used for drug delivery,Citation49 such as small molecules,Citation57 DNA,Citation58 small interfering RNA (siRNA)Citation59 and proteins.Citation60 For example, Shuai et al prepared a polymersome-based nanoprobe consisting of PFP, pentafluorobutane (PFB) and DOX for effective drug delivery into deep tissue through US ().Citation61 Once reaching the tumor site, the nanoprobes swelled and caused the reduction of the vaporization threshold of PFP/PFB, which resulted in the generation of nano/micro-bubbles for enhanced imaging and drug delivery. Moreover, under low-frequency US irradiation, the released DOX from nanoprobes could realize deep penetration.
Figure 4 (A) Schematic illustration of theranostical nanoprobes showing tunable size and performance in vivo derived from multi-stimulation sensitivity. Reprinted with permission from ACS Nano. Zhang L, Yin T, Li B et al. Size-modulable nanoprobe for high-performance ultrasound imaging and drug delivery against cancer, pages 3449–3460. Copyright 2018 American Chemical Society.Citation61 (B) Schematic diagram of disrupting the BBB by magnetically guidable theranostic nanobubbles. Reprinted from Advanced Materials. Huang H, Liu H, Hsu P et al. A multitheragnostic nanobubble system to induce blood–brain barrier disruption with magnetically guided focused ultrasound. © 2014 WILEY-VCH Verlag GmbH & Co. KGaA, Weinheim.Citation64
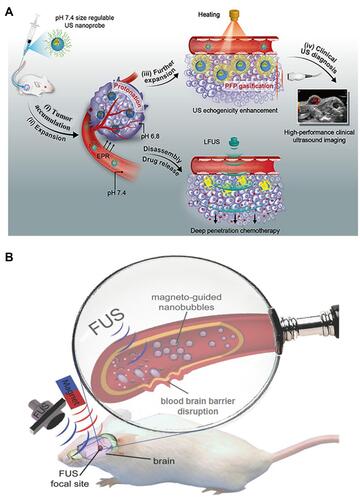
In addition, US can be used to facilitate drug delivery across the blood-brain barrier (BBB),Citation9,Citation62 which is comprised of endothelial cells and protects the brain from harmful substances.Citation63 As a typical paradigm, Chen’s group designed a silica shell consisting of super-paramagnetic iron oxide and nanobubbles for magnetically guidable focused US-induced BBB disruption ().Citation64
High Intensity Focused Ultrasound
HIFU, which uses the thermal effect for achieving ablation based on the high intensity, has been utilized for the cancer treatment.Citation50 However, the relatively low curative effect has limited its further extensive application.Citation65 With the help of NPs, the therapeutic efficacy of HIFU can be efficiently enhanced. Most recently, Chen et al designed silica-coated PLGA NPs encapsulating perfluorocarbon (PFOB), superparamagnetic Fe3O4 and antitumor ruthenium complex (RuPOP) ().Citation66 Upon HIFU irradiation, the PFOB gasified and caused the collapse of silicon shell, which resulted in improved HIFU therapeutic effect. Furthermore, the blasting behavior also triggered the burst release of RuPOP in tumor tissue, which was contributed to maximize the synergistic effect of HIFU and chemotherapy. Furthermore, the addition of superparamagnetic Fe3O4 can realize US/MR dual mode imaging.
Figure 5 Schematic illustration of nano-bomb with site-specific drug burst release to achieve HIFU/chemotherapy synergistic therapy under the US/MR-guided imaging. Reprinted from Journal of Controlled Release. Mai X, Chang Y, You Y et al. Designing intelligent nano-bomb with on-demand site-specific drug burst release to synergize with high-intensity focused ultrasound cancer ablation, pages 270–281. Copyright 2020, with permission from Elsevier.Citation66
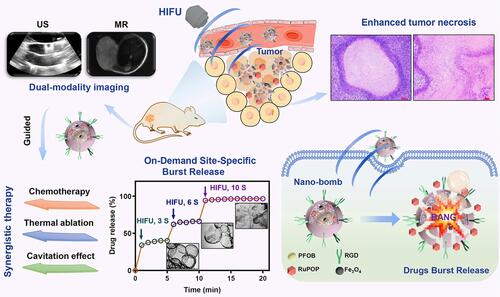
Sonodynamic Therapy-Based Combination Therapy
Based on NPs, synergistic antitumor effect could be realized through combining SDT with other therapeutic strategies, such as chemotherapy, chemodynamic therapy (CDT), immunotherapy, photothermal therapy (PTT), photodynamic therapy (PDT) and so on.Citation9,Citation10 In this section, we have listed and discussed several combined therapies based on SDT ().
Table 1 Sonodynamic Therapy-Based Combination Therapy
Sonodynamic Therapy Combined with Chemotherapy
Chemotherapy is the most common and highly effective method for cancer therapy.Citation67 However, the emergence of drug resistance always results in poor clinical prognosis.Citation10,Citation67 SDT is able to activate the caspase signaling pathway and downregulate the adenosine triphosphate-binding cassette (ABC) transporters, which is contributed to overcome the drug resistance.Citation68 Meanwhile, SDT can trigger the drug release in tumor site. Taken together, synergistic effect could be achieved through combining SDT and chemotherapy.Citation36,Citation69–Citation78 Recently, Chen et al fabricated a self-assemble sonosensitizer composed of hydrophobic fluorescent dye chlorin e6 (Ce6), hydrophobic antitumor drug paclitaxel (PTX) and hydrophilic cyanine dye IR783 (named as Ce6-PTX@IR783, ).Citation69 Among these three parts, Ce6 can improve the efficacy of SDT, PTX was used for chemotherapy, and IR783 was applied for tumor-targeting and PAI. Upon US irradiation (1.0 MHz, 1.0–1.5 W/cm2), Ce6-PTX@IR783 can realize synergistic effect of SDT and chemotherapy.
Figure 6 Schematic illustration of SDT-chemo combination therapy based on Ce6-PTX@IR783, including transportation in blood vessels, tumor accumulation, PA image, drug release and US-triggered synergistic effect. Reprinted from Nanoscale. Dong C, Jiang Q, Qian X et al. A self-assembled carrier-free nanosonosensitizer for photoacoustic imaging-guided synergistic chemo-sonodynamic cancer therapy, pages 5587–5600. Copyright 2020, with permission from Royal Society of Chemistry.Citation69
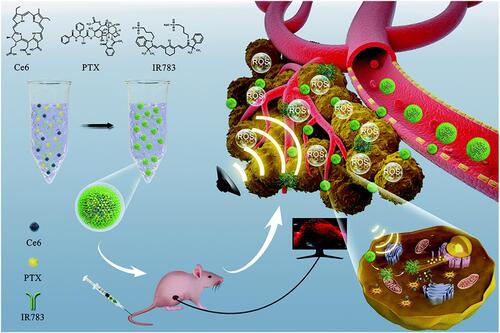
Sonodynamic Therapy Combined with Chemodynamic Therapy
Chemodynamic therapy (CDT) is an ROS-based therapeutic modality, which utilize the acidity and overexpressed H2O2 of tumor microenvironment. Through Fenton or Fenton-like reactions, highly toxic •OH could be in situ produced by catalyzing H2O2 without external stimulation, thus avoiding the challenges of limited penetration and side effects.Citation79 Investigators have doped the elements of CDT (such as Fe3+/Fe2+, Cu2+/Cu+, Mn2+) into SDT to achieve the synergistic treatment, which leads to encouraging results.Citation80–Citation83 Most recently, Yang et al reported PtCu3 nanocages as sonosensitizer with high ROS production under US irradiation (35 kHz, 3 W/cm2, ).Citation80 Meanwhile, these nanocages can act as Fenton-like nanozymes to catalyze the decomposition of H2O2 into •OH under the acidic tumor microenvironment for CDT. Moreover, PtCu3 nanocages were able to mimic glutathione peroxidase to accelerate glutathione (GSH) depletion. Taken together, PtCu3 nanocages can realize effective CDT-enhanced SDT.
Figure 7 Schematic image of photoacoustic (PA)/computed tomography (CT) dual-modal imaging guided CDT/SDT combination therapy by PtCu3 nanocages. The PtCu3 nanocages can generate 1O2 and •OH upon the US irradiation. Furthermore, PtCu3 nanocages as horseradish peroxidase (HRP) mimic could also generate •OH via Fenton-like reaction for CDT. Interestingly, PtCu3 nanocages as another glutathione peroxidase (GSH-Px)-like nanozyme can accelerate the depletion of GSH. Reprinted from Advanced Functional Materials. Zhong X, Wang X, Cheng L et al. GSH-depleted PtCu3 nanocages for chemodynamic-enhanced sonodynamic cancer therapy. © 2019 WILEY-VCH Verlag GmbH & Co. KGaA, Weinheim.Citation80
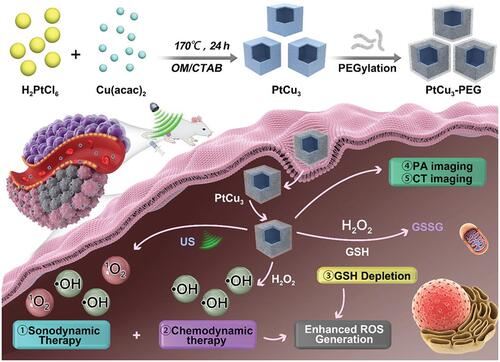
Sonodynamic Therapy Combined with Immunotherapy
Immunotherapy aims to active the immune system to search and destroy tumor cells.Citation84 An activated immune response is able to facilitate systemic immune surveillance, eliminate local and metastatic tumor. Moreover, immunotherapy could generate long-term immune memory to prevent cancer recurrence. Meanwhile, SDT has been confirmed to produce tumor cell fragments, which can act as tumor antigens for inducing the antitumor immune effect.Citation85 Therefore, the combination of SDT and immunotherapy has been successively developed.Citation86–Citation89 For example, Liu’s group fabricated two-dimensional (2D) nanosheets consisting of Zn2+, sonosensitizer tetrakis(4-carboxyphenyl) porphyrin (TCPP) and immune adjuvant cytosine–phosphorothioate–guanine (CpG) (Zn-TCPP/CpG nanosheets, ).Citation86 Under US irradiation (40 kHz, 2 W/cm2), tumor-associated antigens were released through SDT; then, tumor-specific immune responses were triggered. Thereby, through the assist of Zn-TCPP/CpG nanosheets, SDT can not only destruct primary tumors, but also induce effective immune responses and memory to inhibit distant tumors and cancer recurrence.
Figure 8 Schematic illustration of the immune response mechanism induced by Zn-TCPP/CpG nanosheets-based SDT. First, SDT could induce immunogenic cell death (ICD) and release tumor-associated antigens (TAAs), which could be presented by dendritic cells (DCs). Then, the immune system could be activated to inhibit tumor metastasis due to the antigen presentation by matured DCs. In addition, US itself can enhance anti-tumor immune responses through improving T cells infiltration and decreasing regulatory T cells in the tumor microenvironment. Reprinted with permission from Springer Nature Customer Service Centre GmbH: Springer Nature, Nano Research https://www.springer.com/journal/12274. Zhu W, Chen Q, Jin Q et al. Sonodynamic therapy with immune modulatable two-dimensional coordination nanosheets for enhanced anti-tumor immunotherapy. Copyright 2020.Citation86
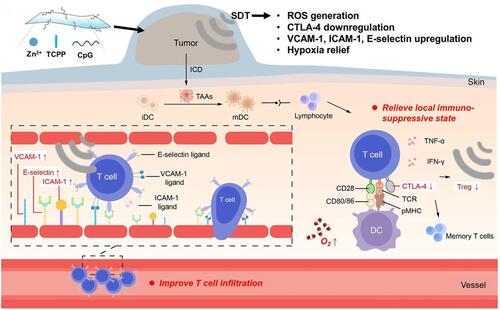
Sonodynamic Therapy Combined with Photothermal Therapy
Photothermal therapy (PTT), providing thermal energy under light irradiation for tumor damage, has become a promising antitumor approach.Citation90 Recently, the combination of SDT and PTT has also been reported.Citation91–Citation96 For example, Lin and co-workers synthesized a novel Pt-CuS Janus consisting of hollow semiconductor CuS, sonosensitizer tetra-(4-aminophenyl) porphyrin (TAPP) and noble metallic Pt ().Citation91 The deposition of Pt not only improved the photothermal performance, but also simulated nanozyme for catalyzing the decomposition of H2O2 to generate O2 that could overcome tumor hypoxia and enhance the ROS production. Under US (1 MHz,1.0 W/cm2) and laser (808 nm) irradiation, the synergistic antitumor effect of SDT and PTT can be realized.
Figure 9 Schematic diagram of preparation and synergistic mechanism of Pt-CuS-P-TAPP. Reprinted with permission from Nano Letters. Liang S, Deng X, Chang Y et al. Intelligent hollow Pt-CuS janus architecture for synergistic catalysis-enhanced sonodynamic and photothermal cancer therapy, pages 4134–4145. Copyright 2019 American Chemical Society.Citation91
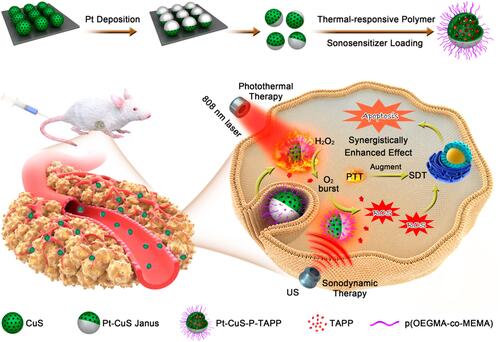
Sonodynamic Therapy Combined with Photodynamic Therapy
Photodynamic therapy (PDT), which utilizes photosensitizers to generate ROS for directly or indirectly perishing cancer cells, has served as an effective modality for cancer therapy.Citation97 However, the limited tissue penetration of light has hindered the widespread application of PDT. SDT can provide deeper penetration depth; meanwhile, most of sonosensitizers are from photosensitizers, which makes these sensitizers could be activated by both US and light irradiation.Citation25 Thereby, the combination of SDT and PDT is able to generate more ROS, which will increase the antitumor efficacy and decrease the sensitizer dose.Citation98–Citation102 For example, Liu et al developed core-shell up-conversion NPs (UCNPs), which loading HMME into up-conversion core and covalently linking rose bengal (RB) on silica (SiO2) shell, for SDT and PDT synergistic antibacterial treatment ().Citation98
Figure 10 Schematic illustration of the construction of UCNP@SiO2-RB/HMME NPs. Reprinted from Biomaterials Science. Xu F, Hu M, Liu C et al. Yolk-structured multifunctional up-conversion nanoparticles for synergistic photodynamic-sonodynamic antibacterial resistance therapy, pages 678–685. Copyright 2017, with permission from Royal Society of Chemistry.Citation98
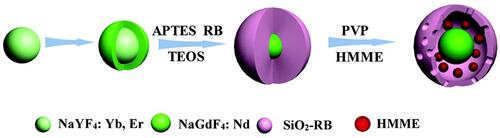
It is worth noting that both SDT and PDT are heavily dependent on O2; hence, O2 could be a breakthrough point for improving the synergistic efficacy. Furthermore, SDT can also combine with some other therapy modalities such as gas therapy, starvation therapy, HIFU and so on.
Challenges and Future Perspectives
NPs-based sonosensitizers have been successfully assisted US applications, especially in SDT. Although clinical trials are still awaited, the pre-clinical data have evidently demonstrated the efficacy of NPs-assisted sonosensitizers in SDT. In order to realize the clinical translation of SDT, there are still several challenges which need to be addressed.
First, the exact mechanism of SDT still remains unclear so far. Future studies ought to confirm the SDT mechanism, including the role of NPs-assisted sonosensitizers and the process of synergistic treatment.
Second, current sonosensitizers suffer from skin sensitivity, low specificity and poor pharmacokinetics, which limit the therapeutic effect of SDT. Further research should focus on exploring novel sonosensitizers with high efficacy and less toxicity through optimizing the structural and acoustic capabilities.
Third, although plenty of studies have proved the short-term safety of involved NPs, long-term toxicity studies are needed to ensure the biosafety of NPs-assisted sonosensitizers. In next step research, NPs with improved biocompatibility and biodegradability need to be developed.
Fourth, the US parameters used in different studies lack of consistency. Hence, further research is needed to optimize US parameters such as frequency, intensity, treatment duration and mechanical index.
So far, NPs-assisted sonosensitizers in biomedical applications are mostly focused on tumor therapy. Future potential prospect could include BBB opening for central nervous system therapy, anti-microbial and bacterial therapy. Although there remain many obstacles to overcome, the development of NPs-assisted SDT will provide more benefits for patients.
Acknowledgments
This work was supported by Natural Science Foundation of China grants (No. 81371581).
Disclosure
The authors disclose no conflicts.
References
- Trendowski M. The promise of sonodynamic therapy. Cancer Metastasis Rev. 2014;33:143–160.
- Costley D, Mc Ewan C, Fowley C, et al. Treating cancer with sonodynamic therapy: a review. Int J Hyperthermia. 2015;31:107–117. doi:10.3109/02656736.2014.992484
- Fan W, Huang P, Chen X. Overcoming the achilles’ heel of photodynamic therapy. Chem Soc Rev. 2016;45:6488–6519. doi:10.1039/C6CS00616G
- Mitragotri S. Healing sound: the use of ultrasound in drug delivery and other therapeutic applications. Nat Rev Drug Discov. 2005;4:255–260. doi:10.1038/nrd1662
- Yang B, Chen Y, Shi J. Reactive Oxygen Species (ROS)-Based Nanomedicine. Chem Rev. 2019;119:4881–4985. doi:10.1021/acs.chemrev.8b00626
- Yumita N, Nishigaki R, Umemura K, Umemura S. Hematoporphyrin as a Sensitizer of Cell-Damaging Effect of Ultrasound. Japan J Cancer Res. 1989;80:219–222. doi:10.1111/j.1349-7006.1989.tb02295.x
- Qian X, Zheng Y, Chen Y. Micro/Nanoparticle-Augmented Sonodynamic Therapy (SDT): breaking the Depth Shallow of Photoactivation. Adv Mater. 2016;28:8097–8129.
- Lin X, Song J, Chen X, Yang H. Ultrasound Activated Sensitizers and Applications. Angewandte Chemie Int Edition. 2020;59:14212–14233. doi:10.1002/anie.201906823
- Liang S, Deng X, Ma P, Cheng Z, Lin J. Recent Advances in Nanomaterial-Assisted Combinational Sonodynamic Cancer Therapy. Adv Mater. 2020;32:2003214. doi:10.1002/adma.202003214
- Son S, Kim JH, Wang X, et al. Multifunctional Sonosensitizers in Sonodynamic Cancer Therapy. Chem Soc Rev. 2020;49:3244–3261. doi:10.1039/C9CS00648F
- Duan L, Yang L, Jin J, Yang F, Gu N. Micro/Nano-Bubble-Assisted Ultrasound to Enhance the EPR Effect and Potential Theranostic Applications. Theranostics. 2020;10:462–483. doi:10.7150/thno.37593
- Rosenthal I, Sostaric JZ, Riesz P. Sonodynamic Therapy-a Review of the Synergistic Effects of Drugs and Ultrasound. Ultrason Sonochem. 2004;11:349–363. doi:10.1016/j.ultsonch.2004.03.004
- Tharkar P, Varanasi R, Wong WSF, Jin CT, Chrzanowski W. Nano-enhanced drug delivery and therapeutic ultrasound for cancer treatment and beyond. Front Bioeng Biotechnol. 2019;7:324. doi:10.3389/fbioe.2019.00324
- Li H, Shi W, Huang W, et al. Carbon Quantum Dots/TiOx Electron Transport Layer Boosts Efficiency of Planar Heterojunction Perovskite Solar Cells to 19%. Nano Lett. 2017;17:2328–2335. doi:10.1021/acs.nanolett.6b05177
- Zhang H, Chen J, Zhu X, et al. Ultrasound induced phase-transition and invisible nanobomb for imaging-guided tumor sonodynamic therapy. J Mater Chem B. 2018;6:6108–6121. doi:10.1039/C8TB01788C
- Gong F, Cheng L, Yang N, et al. Ultrasmall Oxygen-Deficient Bimetallic Oxide MnWOX Nanoparticles for Depletion of Endogenous GSH and Enhanced Sonodynamic Cancer Therapy. Adv Mater. 2019;31:1900730. doi:10.1002/adma.201900730
- Han X, Huang J, Jing X, et al. Oxygen-deficient black titania for synergistic/enhanced sonodynamic and photoinduced cancer therapy at near infrared-ii biowindow. Acs Nano. 2018;12:4545. doi:10.1021/acsnano.8b00899
- Greillier P, Bawiec C, Bessière F, Lafon C. Therapeutic Ultrasound for the Heart: state of the Art. IRBM. 2018;39:227–235. doi:10.1016/j.irbm.2017.11.004
- Coussios CC, Roy RA. Applications of Acoustics and Cavitation to Noninvasive Therapy and Drug Delivery. Annu Rev Fluid Mech. 2008;40:395–420. doi:10.1146/annurev.fluid.40.111406.102116
- Sankin GN, Simmons WN, Zhu SL, Zhong P. Shock wave interaction with laser-generated single bubbles. Phys Rev Lett. 2005;95:034051. doi:10.1103/PhysRevLett.95.034501
- Pang X, Xiao Q, Cheng Y, et al. Bacteria-responsive nanoliposomes as smart sonotheranostics for multidrug resistant bacterial infections. ACS Nano. 2019;13:2427–2438. doi:10.1021/acsnano.8b09336
- Feng Y, Tian Z, Wan M. Bioeffects of Low-Intensity Ultrasound in vitro: apoptosis, Protein Profile Alteration, and Potential Molecular Mechanism. J Ultrasound Med. 2010;29:963–974. doi:10.7863/jum.2010.29.6.963
- Sirsi SR, Borden MA. State-of-the-Art Materials for Ultrasound-Triggered Drug Delivery. Adv Drug Deliv Rev. 2014;72:3–14. doi:10.1016/j.addr.2013.12.010
- Barnett SB, Rott HD, Haar GRT, Ziskin MC, Maeda K. The sensitivity of biological tissue to ultrasound. Ultrasound Med Biol. 1997;23:805–812. doi:10.1016/S0301-5629(97)00027-6
- Umemura S, Kawabata K, Sasaki K. Recent advances in sonodynamic approach to cancer therapy. Ultrason Sonochem. 1996;3:187–191. doi:10.1016/S1350-4177(96)00024-7
- Chen H, Zhou X. Recent Progress in Development of New Sonosensitizers for Sonodynamic Cancer Therapy. Drug Discov Today. 2014;19:502–509. doi:10.1016/j.drudis.2014.01.010
- Umemura K, Yumita N, Nishigaki R, Umemura S. Sonodynamically Induced Antitumor Effect of Pheophorbide a. Cancer Lett. 1996;102(1–2):151–157. doi:10.1016/0304-3835(96)04174-2
- Shi H, Liu Q, Qin X, Wang P, Wang X. Pharmacokinetic Study of a Novel Sonosensitizer Chlorin-e6 and its Sonodynamic Anti-Cancer Activity in Hepatoma-22 Tumor-Bearing Mice. Biopharm Drug Dispos. 2011;32:319–332. doi:10.1002/bdd.761
- Wang H, Liu Q, Zhang K, et al. Comparison Between Sonodynamic and Photodynamic Effect on MDA-MB-231 Cells. J Photochem Photobiol B. 2013;127:182–191. doi:10.1016/j.jphotobiol.2013.08.015
- Wang P, Xu CS, Xu J, Wang X, Leung AW. Hypocrellin B Enhances Ultrasound-Induced Cell Death of Nasopharyngeal Carcinoma Cells. Ultrasound Med Biol. 2010;36:336–342. doi:10.1016/j.ultrasmedbio.2009.09.007
- El-Sikhry HE, Miller GG, Madiyalakan MR, Seubert JM. Sonodynamic and Photodynamic Mechanisms of Action of The Novel Hypocrellin Sonosensitizer, SL017: mitochondrial Cell Death is Attenuated by 11, 12-Epoxyeicosatrienoic Acid. Invest New Drugs. 2011;29:1328–1336. doi:10.1007/s10637-010-9495-2
- Ai X, Lyu L, Zhang Y, et al. Remote Regulation of Membrane Channel Activity by Site-Specific Localization of Lanthanide-Doped Upconversion Nanocrystals. Angewandte Chemie Int Edition. 2017;56:3031–3035. doi:10.1002/anie.201612142
- Qian J, Gao Q. Sonodynamic Therapy Mediated by Emodin Induces the Oxidation of Microtubules to Facilitate the Sonodynamic Effect. Ultrasound Med Biol. 2018;44:853–860. doi:10.1016/j.ultrasmedbio.2017.12.016
- Wang F, Gao Q, Guo S, et al. The Sonodynamic Effect of Curcumin on THP-1 Cell-Derived Macrophages. Biomed Res Int. 2013;2013:737264.
- Pang X, Xiao Q, Cheng Y, et al. Bacteria-Responsive Nanoliposomes as Smart Sonotheranostics for Multidrug Resistant Bacterial Infections. ACS Nano. 2019;13:2438.
- Liu M, Khan AR, Ji J, Lin G, Zhao X, Zhai G. Crosslinked Self-Assembled Nanoparticles for Chemo-Sonodynamic Combination Therapy Favoring Antitumor, Antimetastasis Management and Immune Responses. J Controlled Release. 2018;290:150–164. doi:10.1016/j.jconrel.2018.10.007
- Qian X, Han X, Chen Y. Insights into The Unique Functionality of Inorganic Micro/Nanoparticles for Versatile Ultrasound Theranostics. Biomaterials. 2017;142:13–30. doi:10.1016/j.biomaterials.2017.07.016
- Deepagan VG, You DG, Um W, et al. Long-Circulating Au-TiO2 Nanocomposite as a Sonosensitizer for ROS-Mediated Eradication of Cancer. Nano Lett. 2016;16:6257–6264. doi:10.1021/acs.nanolett.6b02547
- You DG, Deepagan VG, Um W, et al. ROS-Generating TiO2 Nanoparticles for Non-Invasive Sonodynamic Therapy of Cancer. Sci Rep. 2016;6:23200. doi:10.1038/srep23200
- Chen H, Shao L, Li Q, Wang J. Gold Nanorods and Their Plasmonic Properties. Chem Soc Rev. 2013;42:2679–2724. doi:10.1039/C2CS35367A
- Zhou LQ, Li P, Cui XW, Dietrich CF. Ultrasound Nanotheranostics in Fighting Cancer: advances and Prospects. Cancer Lett. 2020;470:204–219. doi:10.1016/j.canlet.2019.11.034
- Karina DOGA, Vieira DP, Courrol LC. Synthesis and Characterization of Aminolevulinic Acid Gold Nanoparticles: photo and Sonosensitizer Agent for Atherosclerosis. J Lumin. 2018;197:317–323. doi:10.1016/j.jlumin.2018.01.057
- Bernard V, Zobac O, Sopousek J, Mornstein V. AgCu Bimetallic Nanoparticles under Effect of Low Intensity Ultrasound: the Cell Viability Study In Vitro. J Cancer Res. 2014;2014:971769. doi:10.1155/2014/971769
- Yumita N, Iwase Y, Imaizumi T, et al. Sonodynamically-Induced Anticancer Effects by Functionalized Fullerenes. Anticancer Res. 2013;33:3145–3151.
- Osminkina LA, Nikolaev AL, Sviridov AP, Andronova NV, Tamarov KP. Porous Silicon Nanoparticles as Efficient Sensitizers for Sonodynamic Therapy of Cancer. Microporous Mesoporous Materials. 2015;210:169–175. doi:10.1016/j.micromeso.2015.02.037
- Sviridov AP, Andreev VG, Ivanova EM, Osminkina LA, Tamarov KP, Timoshenko VY. Porous Silicon Nanoparticles as Sensitizers for Ultrasonic Hyperthermia. Appl Phys Lett. 2013;103:2873. doi:10.1063/1.4829148
- Yu L, Hu P, Chen Y. Gas-Generating Nanoplatforms: material Chemistry, Multifunctionality, and Gas Therapy. Adv Mater. 2018;30:1801964.
- Park SM, Aalipour A, Vermesh O, Yu JH, Gambhir SS. Towards Clinically Translatable in vivo Nanodiagnostics. Nature Rev Materials. 2017;2:17014. doi:10.1038/natrevmats.2017.14
- Sirsi SR, Borden MA. State-of-the-Art Materials for Ultrasound-Triggered Drug Delivery. Adv Drug Deliv Rev. 2014;72:14.
- Kennedy JE. High-Intensity Focused Ultrasound in the Treatment of Solid Tumours. Nat Rev Cancer. 2005;5:321–327. doi:10.1038/nrc1591
- Oeffinger BE, Wheatley MA. Development and Characterization of a Nano-Scale Contrast Agent. Ultrasonics. 2004;42:343–347. doi:10.1016/j.ultras.2003.11.011
- Brigger I, Dubernet C, Couvreur P. Nanoparticles in Cancer Therapy and Diagnosis. Adv Drug Deliv Rev. 2002;54:631–651. doi:10.1016/S0169-409X(02)00044-3
- Mu J, Lin J, Huang P, Chen X. Development of endogenous enzyme-responsive nanomaterials for theranostics. Chem Soc Rev. 2018;47:5554–5573. doi:10.1039/C7CS00663B
- Min HS, You DG, Son S, et al. Echogenic glycol chitosan nanoparticles for ultrasound-triggered cancer theranostics. Theranostics. 2015;5:1402–1418. doi:10.7150/thno.13099
- Li C, Zhang Y, Li Z, et al. Light-Responsive Biodegradable Nanorattles for Cancer Theranostics. Adv Mater. 2018;30:1706150. doi:10.1002/adma.201706150
- Tang H, Guo Y, Peng L, et al. In Vivo Targeted, Responsive, and Synergistic Cancer Nanotheranostics by Magnetic Resonance Imaging-Guided Synergistic High-Intensity Focused Ultrasound Ablation and Chemotherapy. ACS Appl Mater Interfaces. 2018;10:15428–15441. doi:10.1021/acsami.8b01967
- Schroeder A, Honen R, Turjeman K, Gabizon A, Kost J, Barenholz Y. Ultrasound Triggered Release of Cisplatin from Liposomes in Murine tumors. J Controlled Release. 2009;137:63–68. doi:10.1016/j.jconrel.2009.03.007
- Yoon CS, Park JH. Ultrasound-Mediated Gene Delivery. Expert Opin Drug Deliv. 2010;7:321–330. doi:10.1517/17425241003596329
- Negishi Y, Endo Y, Fukuyama T, et al. Delivery of siRNA into the Cytoplasm by Liposomal Bubbles and Ultrasound. J Controlled Release. 2008;132:124–130. doi:10.1016/j.jconrel.2008.08.019
- Lu CT, Zhao YZ, Gao HS, et al. Comparing encapsulation efficiency and ultrasound-triggered release for protein between phospholipid-based microbubbles and liposomes. J Microencapsul. 2010;27:115–121. doi:10.3109/02652040903051996
- Zhang L, Yin T, Li B, et al. Size-modulable nanoprobe for high-performance ultrasound imaging and drug delivery against cancer. ACS Nano. 2018;12:3449–3460. doi:10.1021/acsnano.8b00076
- Zhao YZ, Du LN, Lu CT, Jin YG, Ge SP. Potential and Problems in Ultrasound-Responsive Drug Delivery Systems. Int J Nanomedicine. 2013;8:1621–1633. doi:10.2147/IJN.S43589
- Armulik A, Genové G, Mäe M, et al. Pericytes Regulate the Blood-Brain Barrier. Nature. 2010;468:557–561. doi:10.1038/nature09522
- Huang HY, Liu HL, Hsu PH, et al. System to Induce Blood-Brain Barrier Disruption with Magnetically Guided Focused Ultrasound. Adv Mater. 2015;27:655–661. doi:10.1002/adma.201403889
- Yoshizawa S, Matsuura K, Takagi R, Yamamoto M, Umemura S. Detection of Tissue Coagulation by Decorrelation of Ultrasonic Echo Signals in Cavitation-Enhanced High-Intensity Focused Ultrasound Treatment. J Therapeutic Ultrasound. 2016;4:15. doi:10.1186/s40349-016-0060-0
- Mai X, Chang Y, You Y, He L, Chen T. Designing intelligent nano-bomb with on-demand site-specific drug burst release to synergize with high-intensity focused ultrasound cancer ablation. J Controlled Release. 2020;331:270–281. doi:10.1016/j.jconrel.2020.09.051
- DeVita VT, Chu E. A History of Cancer Chemotherapy. Cancer Res. 2008;68:8643–8653. doi:10.1158/0008-5472.CAN-07-6611
- Wan GY, Liu Y, Chen BW, Liu YY, Wang YS, Zhang N. Recent Advances of Sonodynamic Therapy in Cancer Treatment. Cancer Biol Med. 2016;13:325–338. doi:10.20892/j.issn.2095-3941.2016.0068
- Dong C, Jiang Q, Qian X, et al. Nanosonosensitizer for Photoacoustic Imaging-Guided Synergistic Chemo-Sonodynamic Cancer Therapy. Nanoscale. 2020;12:5587–5600. doi:10.1039/C9NR10735E
- Liu Y, Wan G, Guo H, et al. System combines sonodynamic therapy and chemotherapy to treat hepatocellular carcinoma. Nano Res. 2017;10:834–855. doi:10.1007/s12274-016-1339-8
- Shen S, Wu L, Liu J, et al. Core–Shell Structured Fe3O4@TiO2-Doxorubicin Nanoparticles for Targeted Chemo-Sonodynamic Therapy of Cancer. Int J Pharm. 2015;486:380–388. doi:10.1016/j.ijpharm.2015.03.070
- Shen S, Guo X, Wu L, et al. Dual-Core@Shell-Structured Fe3O4-NaYF4@TiO2 Nanocomposites as a Magnetic Targeting Drug Carrier for Bioimaging and Combined Chemo-Sonodynamic Therapy. J Mater Chem B. 2014;2:5775–5784. doi:10.1039/C4TB00841C
- Zhao H, Zhao B, Li L, et al. Biomimetic decoy inhibits tumor growth and lung metastasis by reversing the drawbacks of sonodynamic therapy. Adv Healthcare Mater. 2020;9:1901335. doi:10.1002/adhm.201901335
- Malekmohammadi S, Hadadzadeh H, Rezakhani S, Amirghofran Z. Design and synthesis of gatekeeper coated dendritic silica/titania mesoporous nanoparticles with sustained and controlled drug release properties for targeted synergetic chemo-sonodynamic therapy. ACS Biomaterials Sci Eng. 2019;5:4405–4415. doi:10.1021/acsbiomaterials.9b00237
- Wu P, Sun Y, Dong W, et al. Enhanced Anti-Tumor Efficacy of Hyaluronic Acid Modified Nanocomposites Combined with Sonochemotherapy Against Subcutaneous and Metastatic Breast Tumors. Nanoscale. 2019;11:11470–11483. doi:10.1039/C9NR01691K
- Li Z, Han J, Yu L, et al. Synergistic Sonodynamic/Chemotherapeutic Suppression of Hepatocellular Carcinoma by Targeted Biodegradable Mesoporous Nanosonosensitizers. Adv Funct Mater. 2018;28:1800145. doi:10.1002/adfm.201800145
- Shi J, Chen Z, Wang B, Wang L, Lu T, Zhang Z. Reactive oxygen species-manipulated drug release from a smart envelope-type mesoporous titanium nanovehicle for tumor sonodynamic-chemotherapy. ACS Appl Mater Interfaces. 2015;7:28554–28565. doi:10.1021/acsami.5b09937
- Wang J, Jiao Y, Shao Y. Mesoporous silica nanoparticles for dual-mode chemo-sonodynamic therapy by low-energy ultrasound. Materials. 2018;11:2041. doi:10.3390/ma11102041
- Tang Z, Liu Y, He M, Bu W. Chemodynamic therapy: tumour microenvironment-mediated fenton and fenton-like reactions. Angewandte Chemie Int Edition. 2019;58:946–956. doi:10.1002/anie.201805664
- Zhong X, Wang X, Cheng L, Tang Y. GSH-Depleted PtCu3 Nanocages for chemodynamic-enhanced sonodynamic cancer therapy. Adv Funct Mater. 2020;30:1907954. doi:10.1002/adfm.201907954
- Li WP, Su CH, Chang YC, Lin YJ, Yeh CS. Ultrasound-Induced Reactive Oxygen Species Mediated Therapy and Imaging Using a Fenton Reaction Activable Polymersome. ACS Nano. 2016;10:2017–2027. doi:10.1021/acsnano.5b06175
- Wang X, Zhong X, Bai L, et al. Ultrafine Titanium Monoxide (TiO1+x) Nanorods for Enhanced Sonodynamic Therapy. J Am Chem Soc. 2020;142:6527–6537. doi:10.1021/jacs.9b10228
- Lin X, Liu S, Zhang X, et al. An Ultrasound Activated Vesicle of Janus Au-MnO Nanoparticles for Promoted Tumor Penetration and Sono-Chemodynamic Therapy of Orthotopic Liver Cancer. Angewandte Chemie Int Edition. 2020;59:1682–1688. doi:10.1002/anie.201912768
- Nam J, Son S, Park KS, Zou W, Shea LD, Moon JJ. Cancer Nanomedicine for Combination Cancer Immunotherapy. Nature Reviews Materials. 2019;4:398–414. doi:10.1038/s41578-019-0108-1
- Liu M, Khan AR, Ji J, Lin G, Zhao X, Zhai G. Crosslinked self-assembled nanoparticles for chemo-sonodynamic combination therapy favoring antitumor, antimetastasis management and immune responses. J Controlled Release. 2018;290:150.
- Zhu W, Chen Q, Jin Q, et al. Sonodynamic therapy with immune modulatable two-dimensional coordination nanosheets for enhanced anti-tumor immunotherapy. Nano Res. 2020;14:1–10.
- Yue W, Chen L, Yu L, et al. Checkpoint Blockade and Nanosonosensitizer-Augmented Noninvasive Sonodynamic Therapy Combination Reduces Tumour Growth and Metastases in Mice. Nat Commun. 2019;10:2025. doi:10.1038/s41467-019-09760-3
- Pang X, Liu X, Cheng Y, et al. Sono-Immunotherapeutic Nanocapturer to Combat Multidrug-Resistant Bacterial Infections. Adv Mater. 2019;31:1902530. doi:10.1002/adma.201902530
- Um W, Ko H, You DG, et al. Necroptosis-inducible polymeric nanobubbles for enhanced cancer sonoimmunotherapy. Adv Mater. 2020;32:1907953. doi:10.1002/adma.201907953
- Huang X, El-Sayed IH, Qian W, El-Sayed MA. Cancer cell imaging and photothermal therapy in the near-infrared region by using gold nanorods. J Am Chem Soc. 2006;128:2115–2120. doi:10.1021/ja057254a
- Liang S, Deng X, Chang Y, et al. Intelligent hollow pt-Cus janus architecture for synergistic catalysis-enhanced sonodynamic and photothermal cancer therapy. Nano Lett. 2019;19:4134–4145. doi:10.1021/acs.nanolett.9b01595
- Han X, Huang J, Jing X, et al. Oxygen-deficient black titania for synergistic/enhanced sonodynamic and photoinduced cancer therapy at near infrared-II biowindow. ACS Nano. 2018;12:4545–4555.
- Dai C, Zhang S, Liu Z, Wu R, Chen Y. Two-dimensional graphene augments nanosonosensitized sonocatalytic tumor eradication. ACS Nano. 2017;11:9467–9480. doi:10.1021/acsnano.7b05215
- Gao F, He G, Yin H, et al. Titania-Coated 2D gold nanoplates as nanoagents for synergistic photothermal/sonodynamic therapy in the second near-infrared window. Nanoscale. 2019;11:2374–2384. doi:10.1039/C8NR07188H
- Su K, Tan L, Liu X, et al. Rapid photo-sonotherapy for clinical treatment of bacterial infected bone implants by creating oxygen deficiency using sulfur doping. ACS Nano. 2020;14:2077–2089. doi:10.1021/acsnano.9b08686
- Liu Y, Wang Y, Zhen W, et al. Defect modified zinc oxide with augmenting sonodynamic reactive oxygen species generation. Biomaterials. 2020;251:120075. doi:10.1016/j.biomaterials.2020.120075
- Dolmans DE, Fukumura D, Jain RK. Photodynamic Therapy for Cancer. Nat Rev Cancer. 2003;3:380–387. doi:10.1038/nrc1071
- Xu F, Hu M, Liu C, Choi SK. Yolk-structured multifunctional up-conversion nanoparticles for synergistic photodynamic-sonodynamic antibacterial resistance therapy. Biomater Sci. 2017;5:678–685. doi:10.1039/C7BM00030H
- Abd El-Kaream SA, Abd Elsamie GH, Abd-Alkareem AS. Sono-photodynamic modality for cancer treatment using bio-degradable bio-conjugated sonnelux nanocomposite in tumor-bearing mice: activated cancer therapy using light and ultrasound. Biochem Biophys Res Commun. 2018;503:1075–1086. doi:10.1016/j.bbrc.2018.06.119
- Wang Z, Liu C, Zhao Y, et al. Photomagnetic nanoparticles in dual-modality imaging and photo-sonodynamic activity against bacteria. Chem Eng J. 2019;356:811–818. doi:10.1016/j.cej.2018.09.077
- Nomikou N, Curtis K, McEwan C, et al. Stimulus-responsive nanoparticle-based platform for use in both sonodynamic and photodynamic cancer therapy. Acta Biomaterialia. 2017;49:414–421. doi:10.1016/j.actbio.2016.11.031
- Liu Z, Li J, Jiang Y, Wang D. Multifunctional nanocapsules on a seesaw balancing sonodynamic and photodynamic therapies against superficial malignant tumors by effective immune-enhancement. Biomaterials. 2019;218:119251. doi:10.1016/j.biomaterials.2019.119251