Abstract
The aim of this study was to produce and characterize nanoparticles (NPs), combining chondroitin sulfate (CS) and fucoidan (FC) with chitosan for therapeutic purposes. These NPs were characterized by dynamic light scattering, zeta potential determination, and transmission electronic microscopy. The anticoagulant activity was determined for FC NPs and compared with FC solution at the same concentration. FC NPs showed regular shapes and better anticoagulant activity than free polysaccharide solution. FC solution did not affect coagulation compared to FC NPs, which increased up to two-fold, even at a lower concentration. Cytotoxicity and permeability tests were conducted using Caco-2 cell monolayer, exhibiting no toxic effect in this cell line and higher permeability for NP2 samples than FC solution at the same concentration.
Introduction
Sulfated polysaccharides are found in many animals (vertebrates and invertebrates) and plant tissues with different polyanionic structures. This feature is due to the presence of sulfate esters and/or hexuronic acid. These macromolecules are largely studied for their interesting biological profile, including anticoagulant activity.Citation1–Citation3 Sulfated polysaccharides occur naturally as acidic polysaccharides. Among them, chondroitin sulfate (CS) is an abundant glycosaminoglycan found in bone, cartilage, and connective mammalian tissue. It is composed of N-acetylgalactosamine and glucuronic acid residues linked by 1–3 generally sulfated on N-acetylgalactosamine at 4- or 6-position. Chondroitin sulfate is able to produce ionic complexes, but only with positively charged molecules, due to its acidic mucopolysaccharide structure.Citation4–Citation7 This polysaccharide has recognized anti-inflammatory and antitumor activities and can be used in combination with other polysaccharides in different hydrogel drug delivery systems.Citation8,Citation9 Another interesting sulfated polysaccharide is the sulfated fucan, which displays anticoagulant and antithrombotic activities.Citation1,Citation10,Citation11 Also known as fucoidan (FC),Citation12 this substance is found in brown algae (Fucus vesiculosus) and displays an ability to increase the inhibition of thrombin by antithrombin or heparin cofactor II.Citation1,Citation2 Biopolymers such as CT are considered natural complexing agents, with the ability to form physical gels or coacervates with sulfated polysaccharides. Chitosan is a linear polysaccharide, composed of units of D-glucosamine and N-acetyl-D-glucosamine, which in acidic pH is in a cationic form.Citation5,Citation13–Citation19 The properties of CT have been studied extensively for its mucoadhesion to the gastrointestinal mucosal epithelium and anti-inflammatory activity.Citation20,Citation21 CT produces insoluble ionic complexes with water-soluble polyanionic species in neutral or alkaline medium. Some negative polyelectrolytes, such as alginate, carboxymethylcellulose, xanthan gum, heparin, and CS, are able to complex with CT.Citation16,Citation22 CS and FC had never been used for the preparation of nanostructured systems with potential antithrombotic and anticoagulant activities. Nanostructured systems play an important role in future therapies because they are widely described in the literature as systems that cross the gastrointestinal epithelial absorption barrier. Moreover, NPs are able to control and direct the release of bioactive substances.Citation23,Citation24 In nanomedicine, a drug (synthetic or a biopolymer) can be dissolved, incorporated, encapsulated and/or adsorbed or attached to NPs in order to (1) improve its pharmacokinetic profile;Citation24–Citation27 (2) raise the effectiveness of treatment;Citation28 (3) reduce the adverse effects of preferential accumulation in specific sites, causing low concentrations in healthy tissues;Citation29 and (4) increase the chemical and conformational stability of a variety of therapeutic agents, such as small molecules, peptides, and oligonucleotides.
Particle size and zeta potential (ZP) are fundamental for colloidal system stabilization. Furthermore, particle absorption due to internalization/endocytosis is also driven by size (dimension) and surface chemistry (ZP), which allow an interaction with cells. In particular, surface chemistry critically affects how NPs interact with each other and the environment and, even more, with cells. Simply classifying the internalization of NPs in terms of their surface charge, neutral and anionic nanoparticles are internalized considerably less efficiently than cationic ones. Therefore, particle interaction with cell membranes has a drastic effect on the uptake process.Citation30
In fact, the attractive interactions lead to a clustering that effectively lowers the free energy threshold for wrapping and, therefore, shifts the lower cutoff radius. When these are repulsed, the minimum radius for endocytosis increases, therefore hindering endocytosis.Citation30
The ionic interaction between CT and some anionic polysaccharides, such as CS,Citation14,Citation31 FC,Citation32 and heparin,Citation33,Citation34 has recently been used to produce micro- (CS) or nanoparticles (heparin) with biological activity. The production of these micro- (CS) and nanostructured materials is feasible through ionic interactions among the polyelectrolytes, ie, the positive charges of CT at acid pH interacting with the negatively charged sulfated polysaccharides (interpolymer complexation). Many scientific reports, including those from Calvo et alCitation35 have pointed to NP production using biodegradable hydrophilic polymers. In these works, a CT NP production method using the ionic interaction with sodium tripolyphosphate for protein delivery was described. Thus, the positive charges of CT amino groups interact with negatively charged tripolyphosphate, forming particulate material in the nanometer range.
NP production through ionic interaction between polyelectrolytes, such as CT and TP, has been previously described.Citation36 The literature also includes the use of CT and dextran for the release of doxorubicin;Citation28 CT and heparinCitation33,Citation34 for gene therapy, and CT and alginateCitation37 and CT and gelatin for the controlled release of cyclosporine-A.Citation29
The aim of this work was to develop a new nanocoacervation method in order to obtain sulfated polysaccharide NPs with anticoagulant activity. The optimization of the anticoagulant activity of these polysaccharides in nanoparticulate systems, which restricts the conformation freedom of these macromolecules, was evaluated by different approaches. Furthermore, the feasibility of preparing CT NPs with different natural polyanions, as a promising oral anticoagulant formulation, was studied.
Materials and methods
Materials
Chitosan of low molar weight/deacetylation degree ≥90% (Sigma, St. Louis, USA), fucoidan from Fucus vesiculosus (Sigma, St. Louis, USA), chondroitin-4-sulfate (Sigma, St Louis, USA), sodium borate (Borax®) (Merck, Darmstadt, Germany), and acetic acid (Vetec, Rio de Janeiro, Brazil) were used in analytical grade. Milli-Q water (Millipore, USA) was used in the preparation of all buffers and solutions.
Methods
Preparation of polysaccharide nanoparticles by nanocoacervation
In this work, methods previously described in the literature were adapted for a regular coacervation process.Citation5,Citation14,Citation38–Citation40 Briefly, CT was used as the positive (cationic) and CS or FC as negative (anionic) polyelectrolytes. Then, 30 mL of a solution of the negative polyelectrolyte in borax buffer or water were dripped (30 mL/h), using a syringe-based droplet system, onto 30 mL of CT in acetic acid 1% (v/v), under magnetic stirring and sonication at 100% intensity in an ice bath for 60 minutes. The distance between the needle and the CT solution was 10 cm. The polyelectrolyte concentrations tested in this work are presented in . After complete dripping, dispersions were centrifuged at 548.51 × g for 30 minutes in a SIGMA 4–16 KH refrigerated centrifuge (Osterode, Germany), the pellet was separated, and the supernatant was centrifuged at 28435.21 × g for 30 minutes. After separating the supernatant, the pellet was resuspended in 1000 μL of water and used in the following measurements. All experiments were performed in triplicate.
Table 1 Combination of polysaccharide solutions to produce four different NP suspensions
Mean diameter and polydispersity index measurements
Mean diameter (MD) and polydispersity index (PI) measurements were conducted on a Horiba LB-550 DLS analyzer (Kyoto, Japan), with a detection angle of 90°, 100 scans over 2 minutes each for each sample, with a refraction index adjusted to 1.33 and a temperature of 22°C. These measurements were performed in triplicate for each NP sample, on different days for different solutions.
Determination of zeta potential
The ZP was measured using Malvern nanosizer software (Worcestershire, United Kingdom). A mean value was obtained from three readings for each sample. The parameter values – pH, refractive index, and average diameter – were imputed in the equipment before analyzing.
Transmission electron microscopy (TEM)
The samples were maintained for 5 minutes on copper grids of 400 mesh covered with formvar film, sprayed with carbon. The grids were dried with filter paper and contrasted in aqueous uranyl acetate 5% for 1 minute, and then observed under a FEI Morgagni (Hillsboro, OR) microscope operating at 80 kV.
Study of particle separation by centrifugation
The NP dispersions were centrifuged at increasing speeds, in order to observe size distribution obtained at each speed used and suitability of NP purification. The following speeds were tested: 87.76, 548.51, 2194.07, 4936.66, 19746.67, and 28435.21 × g. The six pellets were collected and resuspended in 500 μL of water by sonication for 30 minutes. The MD of the resuspended pellet and the supernatant were measured.
Sulfated polysaccharide assays
NP samples isolated by centrifugation were mixed with different acidic solutions (10%, 50%, and 100% acetic acid; 0.1 M and 1 M hydrochloric acid) at 25.0 μg/mL concentration in order to obtain the complete dissolution of polyelectrolyte complex. The dissolution was confirmed by DLS analysis. These samples were complexed with dimethylmethylene blue (DMB) and evaluated by spectrophotometry at 525 nm.Citation41 The concentration of sulfated polysaccharide in the NP samples was calculated using a calibration curve obtained with FC at 1.0, 5.0, 10.0, 20.0, 30.0, and 40.0 μg/mL, in triplicate.
Coagulation tests
The ability of FC NPs to potentiate inhibition of rat plasma coagulation was assessed by measuring NP2 recalcification time in an Amelung KC4 A coagulometer (Mount Holly, NJ). Citrated blood samples were centrifuged (2000 × g) for 10 minutes, and the platelet-poor plasma was stored at −20°C until use. Pre-warmed rat plasma (100 μL containing 3.8% sodium citrate; 1:9, v/v) was incubated with NP2 samples at various concentrations (suspension in saline solution) for 1 minute at 37°C. The addition of 100 μL of pre-warmed 50 mM CaCl2 started plasma clotting. The coagulation time was recorded in seconds.Citation42–Citation44
Additional assays were performed to verify the NP anticoagulant effects on activated partial thromboplastin time (aPTT) and prothrombin time (PT). For aPTT tests, cephalin plus kaolin (aPTT reagent) was incubated for 1 minute with 50 μL of pre-warmed plasma (37°C) and selected NP samples, at various concentrations. The reaction was started by the addition of 100 μL of pre-warmed CaCl2 (50 mM). For PT tests, 50 μL of pre-warmed rat plasma was incubated with NP samples, at various concentrations, for 2 minutes, at 37°C. The reaction was started by the addition of 100 μL of pre-warmed thromboplastin with calcium (PT reagent).Citation42–Citation44 Only FC NPs were tested and compared to free polysaccharide solution, as the effects of anticoagulation for CS are not reported in the literature.
Cytotoxicity test for evaluating polysaccharides Caco-2 cell toxicity
Caco-2 cells were seeded in 96-well plates (CELLSTAR®; Greiner Bio-One GmbH, Frickenhausen, Germany) at a density of 32,000 cells/well, distributed in a total volume of 200 μL/well. Then, the plates were taken to a Galaxy CO2 cell incubator (New Brunswick Scientific, Enfield, CT) at 37°C and 5% CO2 for 24 hours. After incubation, the cells were placed in contact with the following samples: FC solutions in HBSS pH = 6.8 (500, 1000, and 1500 mg/mL), physical mixtures of CT and FC in HBSS pH = 6.8 (250, 500, and 1000 mg/mL), and NPs of CT and FC resuspended in Hank’s Balanced Salt Solution (HBSS) pH = 6.8 (250, 500, and 1000 mg/mL).
HBSS pH = 6.8 was used as a positive control, and a negative solution 10% Tryton X-100 (Tryton® X-100 Merck, Darmstadt, Germany). The samples were kept in contact with the cells for 3 hours and then aspirated and treated with MTT [3-(4,5-Dimethylthiazol-2-yl)-2,5-diphenyl tetrazolium bromide] reagent (2.5 mg/mL) by adding 100 mL of HBSS and 25 mL of MTT solution per well. The plates containing the cells were incubated with MTT for 3 hours at 37°C and 5% CO2. At the end of this incubation time, MTT was aspirated, and the cells were washed with phosphate buffer solution (PBS) (pH = 7.4). The PBS was then aspirated, and 100 mL/well of dimethyl sulfoxide (DMSO) (Sigma Aldrich, Milano, Italy) was added, in order to break the cell membrane. That allowed the release of formazam crystals formed, generating a purple color more or less intense according to the degree of cell viability. The absorbance readings were performed in wells of Microplate Absorbance Reader iMARK™ (Bio-Rad Laboratories Srl, Segrate, Italy), with reference to 570 nm–690 nm, after shaking vigorously for 60 seconds.Citation45
Permeability studies performed by means of Caco-2 cell monolayer
The nanoparticle suspensions (NP2) prepared in pH 6.8 HBSS were subjected to permeability tests across Caco-2 cell monolayers. Caco-2 cells (passage 38) were seeded on tissue-culture-treated polycarbonate filters (area 113.1 mm2; inner diameter 13.85 mm) in 12-well plates (Greiner Bio-one, International PBI, Italy) at a seeding density of 1*105 cells/cm2. Dulbecco’s Modified Eagle’s Medium (DMEM, pH 7.40; Bio Industries, Israel), supplemented with 10% fetal bovine serum, benzylpenicillin G (160 U/mL), and streptomycin sulfate (100 μg/mL) (Bio Industries) as well as with 1% nonessential amino acids (Sigma, I), was used as culture medium. Cell cultures were kept at 37°C in an atmosphere of 95% air and 5% CO2 and 95% of relative humidity. Filters were used for transepithelial electrical resistance (TEER) measurements and transport experiments 21–23 days after seeding.Citation45
Five hundred microliters of FC solution or NP2 suspension, prepared as previously described in the “Preparation of polysaccharide nanoparticles by nanocoacervation” paragraph, at 1000 μg/mL in HBSS at pH 6.8, was used as the apical (donor) phase of the monolayers. HBSS at pH 7.4 (1.5 mL) was used as the basolateral (receptor) phase and added to the basolateral side of the monolayers. At 30, 60, 120, and 180 minutes, each filter and its mounting donor chamber, filled with the apical phase, was moved into a fresh basolateral (receptor) phase. All the receptor phases were collected, and the permeated sulfated polysaccharides were assayed by means of the spectrophotometric method previously described.
During the experiments and until 7 hours afterward, the integrity of the monolayers was assessed by means of TEER measurements at fixed times, using a Millicell ERS-meter (Millipore Corp., Bedford, MA).
Statistical data analysis
Statistical differences were determined using SigmaPlot 11.0 (Systat Software, Inc). Differences between groups were considered significant at P < 0.05.
Results and discussion
The NP preparation method was based on the ionization of CS and FC polysaccharides carboxyl and sulfate groups, which interact efficiently with the positively charged CT under controlled sonication. This method did not use surfactants or organic solvents, only magnetic stirring and sonication to break the millimetric drop and form nanometric spherical particles. Another positive aspect of the present method was the droplet control with a fixed value and controlled distance between the needle and the CT solution, which avoided the undesirable variation of these parameters, making the scale-up method more feasible. The developed method in the present work was less expensive and cleaner than other techniques in the literature.Citation5,Citation14,Citation38–Citation40 A similar procedure was proposed by CitationChen et al (2009);Citation33 however, the NPs were prepared exclusively by heparin solution drop, using a pipette, into a CT solution, without sonication. These results indicated a close relationship between the heparin structure and NP formation, indicating a limited applicability of this polysaccharide. The formulations presented in did not show agglomeration or follow the characterization. The pH measurements of each polysaccharide solution and the nanometric dispersions were carried out in order to optimize the preparation process and to evaluate the impact of pH on the anticoagulant and antithrombotic activity. The pH values of 0.05% and 0.1% CS or FC solutions in borax buffer were between 8.9 and 9.1; for sulfated polysaccharides in water, at the same concentration, the observed pH values were between 6.2 and 6.6. The CT solution 0.05% and 0.1% in acetic acid presented pH values between 2.8 and 3.1. NP dispersions prepared with borax buffer solutions presented pH values between 3.9 and 4.1, whereas those prepared with water presented pH values between 2.5 and 2.7. The results showed that the ionization of only one of the biopolymers using a buffer could lead to coacervate formation. This justifies the procedure described by CitationChen et al (2009),Citation33 wherein a coacervation was obtained by mixing ionized CT solution with heparin dissolved only in water. However, to ensure greater efficiency in the process studied herein, NPs were prepared using buffer in both polysaccharides.
The pH of the NP suspensions indicated the need for centrifugation and washing the material, followed by redispersion, to carry out the testing of in vitro pharmacological activity, to avoid any interference in the obtained results. The highest concentrations of polysaccharides used (0.1% w/v) were similar to those normally used for NP preparation on a laboratory scale. Despite the simplicity of the process, scaling up was not considered in this study, as the goal was to focus on evaluating the effect of the nanostructuring of polysaccharides in their pharmacological activity, and not in the industrialization of the product.
The MD, PI, and ZP values for proposed formulations are shown in . Np1 and Np7 showed the lowest and the highest MD, 154.2 ± 35.77 nm and 453.37 ± 369.48 nm, respectively. According to Gaumet et al,Citation46 smaller NPs are less susceptible to the mononuclear phagocytosis system and present higher circulation times in the organism; therefore, NP1 was selected as the best candidate for the CS carrier, compared to NP3, NP5 and NP7. Despite having sizes close to that of NP1 (P = 0.0034), other nanosystems containing CS showed higher PI and lower reproducibility. NP2 was selected as the best nanocarrier for FC, compared to NP4, NP6, and NP8, for the same reasons applied to NP1.
Table 2 Mean diameter, polydispersity index, and zeta potential values for different formulas
For FC, an increased variation in particle size distribution could be observed. This was probably due to the molecular weight distribution being narrower in CS compared to FC, which did not have the fractions of mass separated in order to simulate a more accurate use of the crude natural product as a new drug. The best experimental result was obtained with NP2, which showed an adequate mean and SD of size distribution, PI, and ZP (P = 0.0021). The lowest MD and PI were reached using 0.1% of polysaccharides solutions in borax buffer (ie, NP1 and NP2) (), indicating the possibility of obtaining a better result with an increased concentration of polysaccharide.
Similar results were observed for all samples using borax buffer solutions (NP1, NP2, NP5, and NP6), which presented lower MD and ZP values than those with water (NP3, NP4, NP7, and NP8). These profiles might be due to the alkaline medium solutions, as acidic polysaccharides are less ionized in neutral solvent, indicating the need for ionization of both polysaccharides to improve the preparation of NPs.
The literature has reported the production of CT NPs with tripolyphosphate by using coacervation with copolymer insertion, such as polyethylene. The MDs obtained for these NPs were between 263.8 and 745.5 nm.Citation35 Apparently, the dripping method performed by these authors presents a higher MD and also increases the cost, mainly due to the use of specific reagents, in comparison to our new methodology. The low polydispersity values obtained for NP1 (0.109 ± 0.015) and NP2 (0.139 ± 0.019) revealed that samples with 0.1% of CS or FC in alkaline medium are more homogenous for MD particles. However, systems obtained using 0.05% polysaccharide solutions did not show the same features. All systems showed positive superficial electrical density. NP samples using borax buffer solution had lower ZP values than those with water. NP1 showed a lower ZP value than NP5 and NP7, and almost the same value as NP3, suggesting that polysaccharide concentration is a non-interfering factor. However, FC NPs did not show the same ZP values for different concentrations, as can be seen in NP2 and NP6. All zeta potential values suggest MD stability for the NPs, as they had no neutral values, avoiding particle aggregation. Low-ZP NPs should produce the best pharmacological profiles, due to a reduction of opsonization.
The literature reports chitosan-heparin NP formation to produce a carrier for bovine serum albumin, using similar polysaccharide concentrations, low molecular weight chitosan, and a dropwise technique. The authors reported values of 204.4 nm, 0.189, and +29.39 mV for MD, PI, and ZP, respectively.Citation47 Sezer et alCitation32 produced fucospheres by using polyelectrolyte complexation between CT and FC. In this report, the smaller fucosphere showed a larger mean diameter than NP2, as well as a higher PI and lower ZP than produced in this study. Those authors did not evaluate the anticoagulant activity of their fucospheres, but rather, their efficacy in the treatment of dermal burns.Citation32 Compared with the previously described chitosan–heparin NP preparation with anticoagulant activity,Citation33 no similar results or reproducibility were obtained for our FC and CS NP preparation, with sulfated polysaccharide incorporation yield into the NPs ranging between 25% and 70%. Characteristic parameters for regression equation (y = 0.0096X + 0.0046) of the DMB sulfated polysaccharide determination method obtained by least squares treatment of the results confirmed the good linearity of the method developed (r = 0.9953). In that regard, five-sample replicates were consecutively tested, using the same equipment, at a concentration of 25 μg/mL, 100% of the normal analytical working value.
The NP1 TEM data presented in , show the presence of NPs with spherical or oval form. There are no significant particle aggregates, and the MD value is close to that observed in the DLS analysis. also shows particles in the 200 nm range, which reinforces the MD data measured by DLS analysis. The NP2 system was also submitted to TEM (), which showed NPs with spherical shape and MD in the same order as DLS analysis.
Figure 1 NP1 TEM images of two different points (A and C) of the same sample (22000×). Images (B and D) shows higher magnification (56000×) of (A and C), respectively.
Abbreviations: NP, nanoparticle; TEM, transmission electron microscopy.
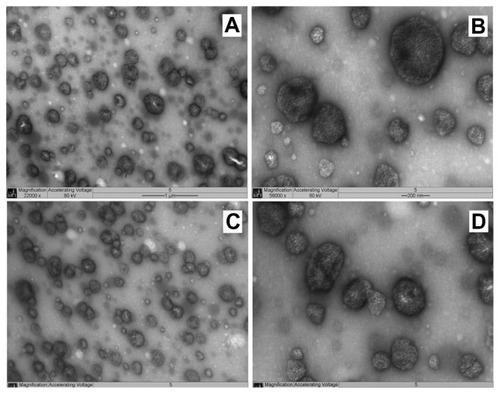
Figure 2 NP2 TEM image (36000× magnification).
Abbreviations: NP, nanoparticle; TEM, transmission electron microscopy.
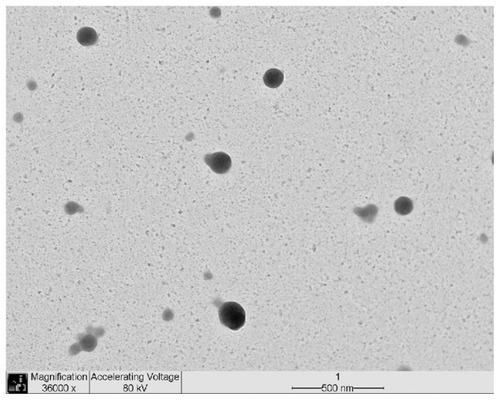
To analyze the effects of coacervation in the shapes of the NPs obtained and compare the sizes of the non-nanostructured material that could agglomerate in vivo, the physical mixture of the polysaccharides was evaluated by TEM analysis. The physical mixture of the polysaccharides (CT + CS and CT + FC) was submitted to common stirring and sonication. According to TEM presented in , the physical mixture morphology was not well defined in this experiment, in contrast to NP1 and NP2, produced by the nanocoacervation method ( and ), with MDs quite similar to those observed for NP1 and NP2. The morphology difference between NP structure and physical mixture arrangement showed that, without sonication, there is no nanosphere formation.
Figure 3 Physical mixtures TEM. (A) Chondroitin sulfate–chitosan mixture; (B) Fucoidan–chitosan mixture.
Abbreviation: TEM, transmission electron microscopy.

The particle analysis performed on NP1 after sequential centrifugation revealed particles presenting MD of 3545.8 nm (>5.0% of total mass) for NP1 at the first step. The supernatant was sequentially centrifuged; the decrease in MD in the NP1 particles is shown in . The NP1 MD decreased to 185.4 nm after centrifugation at 28435.21 × g. This result suggests that lower particles may be separated using a higher speed level and using as ideal conditions the previous centrifugation at 548.51 × g, followed by 28435.21 × g. The main purpose is to discard the major particles and test those with MDs between 100 and 400 nm. This was necessary to avoid high PI and to ensure that the results of anticoagulant activity are due to the NPs and not to the soluble biopolymer. Similar results were observed with NP2 particle separation (). However, the distribution was more heterogeneous than that of NP1, for the reasons already presented. Therefore, a separation procedure identical to that of NP2 was established for NP1. Centrifugation was a good choice, as it is faster than dialysis, less expensive than gel filtration, and less aggressive to polysaccharide NP structures than lyophilization.Citation48 However, the very similar results of PI measurements indicate that the use of unseparated NPs does not represent a problem for pharmacological performance of these new anticoagulant formulations. Therefore, unseparated FC–NP preparations and FC solution were selected and tested in the coagulation assays.
Thus, NP2 and NP1 were selected for the subsequent tests. DLS analysis showed that chloride acid 1 M solution was able to break NP2 containing sulfated polysaccharides, resulting in a solution with a 5.4 nm MD. Other acid solutions could not break the NPs with high MD values and similar diameters. Similar results were obtained for NP1. The soluble FC and CS were quantified by DMB – metachromatic assay. The FC content in resultant NP2 samples was 75.85 ± 0.919 μg/mL (n = 3), and CS content was 25.16 ± 0.817 μg/mL (n = 3). Fucoidan yield in NP2 was similar in these NPs, as reported by CitationChen et al (2009)Citation33 in chitosan–heparin NPs, which used a different polymer.
The in vitro coagulation tests confirmed the anticoagulation activity of the NPs compared to the control, using PBS for NP2 (FC NP), with no significant results for NP1 (CS NP); P = 0.00345.
For NP2, in the aPTT test, the coagulation time (13.3 μg/mL) was two times higher than that of the control (), whereas sulfated polysaccharide free solution increased only 1.6 times (40% lower). The NP dispersion pH was about 4.5, not indicating an effect in in vitro coagulation tests. FC solution (2.67 μg/mL) did not affect the coagulation time compared to the control. On the other hand, NP2 increased the coagulation time, even at a low concentration (0.53 μg/mL) compared to the control (1.6 x). The results of the recalcification tests () were similar to aPTT, with NP2 activity higher than FC solution. NP2 and FC solution (13.3 μg/mL) increased the coagulation time 1.51 and 1.24 times, respectively. In contrast, the prothrombin time (PT) showed no significant difference on coagulation time compared to the control (data not shown). The absence of an activity profile of NP2 and FC solution in PT suggested that the extrinsic pathway is not affected by these substances. Considering the aPTT data, the intrinsic coagulation pathway is the main target of both NP2 and FC solution. These aPTT and PTT results reinforced previous works that showed sulfated polysaccharides in free form affecting the coagulation intrinsic pathway.Citation44
Figure 6 aPTT test for NP2 and FC solution.
Notes: Mean values ± SD; n = 3.
Abbreviations: aPTT, activated partial thromboplastin time; FC, fucoidan; NP, nanoparticle.
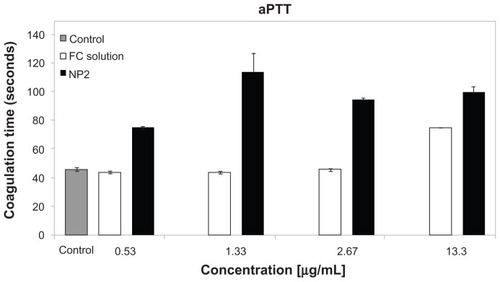
Figure 7 Recalcification test for NP2 and FC solution.
Notes: Mean values ± SD; n = 3.
Abbreviations: FC, fucoidan; NP, nanoparticle; SD, standard deviation.
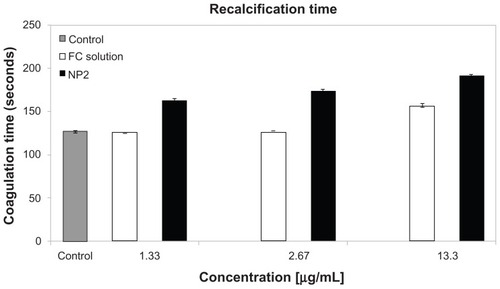
NP2 showed higher anticoagulant activity than FC solution, possibly due to the fact that polysaccharides in solution may assume a nonspecific conformation that leads to lower activity, contrasting with NP2, which has a fixed conformation, permitting a more effective receptor interaction and activity.Citation33
CS did not exhibit the same anticoagulant properties as FC, but there are some reports in the literature showing antinflamatoryCitation49 and anticancer activities.Citation50,Citation51 For these reasons, these CS NPs can be tested as a new anticancer system with carrier properties. In this work, NP1 was prepared only as a nanocoacervation model for NP2, for economic reasons.
It was necessary to conduct the cytotoxicity assay with MTT in order to verify that the NPs, physical mixtures, and polysaccharide solutions showed some toxicity for the Caco-2 cell monolayer, considering the possible oral administration of the nanostructured systems under study. Cell viability is determined by the capacity of the mitochondrial enzyme succinate dehydrogenase to reduce MTT (tetrazole salt) to formazam. The fucoidan–chitosan physical mixture showed a decrease of cell viability, increasing polymer concentrations from 250 ug/mL to 2000 ug/mL and suggesting a cytotoxic effect toward Caco-2 cells, probably due to the large average particle diameter, which was able to stop gas and nutritive substance exchanges from the cell substrate. The sulfated polysaccharide solutions in higher concentrations showed no toxicity by keeping the cells fully viable. In the test using fucoidan–chitosan NPs (NP2, 250, 500, and 1000 ug/mL) did not show any cytotoxic effect and were biocompatible ().
Figure 8 Cytotoxicity test for FC solution, NP2, and physical mixture between FC and CT.
Notes: Mean values ± SD; n = 3.
Abbreviations: CT, chitosan; FC, fucoidan; PM, physical mixture; NP, nanoparticle.
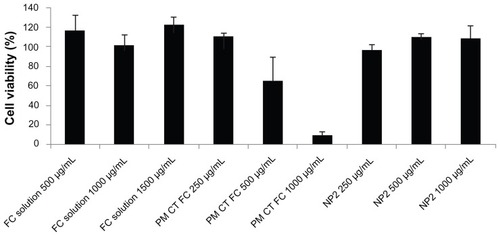
shows TEER% profiles for tested samples and the control (HBSS pH 6.8). At the beginning of the permeation experiments, the mean TEER value was 503.4 Ωcm2 (SD ± 47.92 Ω cm2), to indicate the junctional integrity of Caco-2 cell monolayers. For HBSS pH 6.8, the TEER% profile remains almost close to 100% during the experiment time, indicating that this medium did not impair the permeability barrier of the Caco-2 monolayer in vitro and the paracellular pathway.
Figure 9 TEER% vs time profiles observed for NP2, FC solution, and the control (HBSS pH 6.8).
Notes: Mean values ± SD; n = 3.
Abbreviations: FC, fucoidan; HBSS, Hank’s Balanced Salt Solution; NP, nanoparticle; SD, standard deviation; TEER, transepithelial electrical resistance.

The NP2 system showed TEER% profiles significantly lower than that of the control (P < 0.05) in the first 2 hours and not significantly (P > 0.05) lower from this time until the end of experiment (7 hours). This TEER profile indicates an opening between the cell junctions (TEER decreasing), increasing the Caco-2 monolayer permeability by paracellular pathway, followed by a gradual increase in TEER values (tight junctions closing). These observations indicated that this nanosystem could transiently and reversibly open the tight junctions between Caco-2 cells. This behavior was previously observed in the literature in different pH ranges, displaying more prominence in pH values under 6.8.Citation33 In contrast, the FC solution did not widen the tight junctions, as suggested by TEER values.
The results of sulfated polysaccharides permeation () showed different behaviors for NP2 and FC solution. NP2 presented a higher sulfated polysaccharide permeation percentage from the beginning of the experiment until 120 minutes (P < 0.05), compared to the FC solution. During the first hour, NP2 showed two-fold permeation, followed by a slight decrease of this ratio to 1.77 in the second hour. This result suggests higher permeability rates for NP2 than the corresponding sulfated polysaccharide solution. This could be due to different conformation and dimensions of FC as free polymer chains or in nanoparticulate form. Moreover, since nanoparticle zeta potential was positive due to the presence of chitosan, nanoparticle–cell membrane interactions should be more facilitated with respect to negatively charged free FC molecules. It is conceivable that the size and surface chemistry (zeta potential) of the NPs caused FC absorption via nanoparticle internalization, probably via endocytosis.
Figure 10 Percentage values of permeated sulfated polysaccharides, calculated for the permeability experiment performed using Caco-2 cell monolayers observed for NP2 and FC solution.
Notes: Mean values ± SD; n = 3.
Abbreviations: FC, fucoidan; NP, nanoparticle; SP, sulfated polysaccharides; SD, standard deviation.
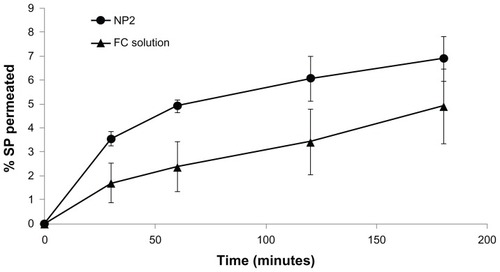
The particle size was not affect by ionic strength: no particle aggregation occurred in the presence of PBS or HBSS. This is a crucial point, because the particle internalization/endocytosis is strongly hindered by particle propensity to aggregation.
Conclusion
In this work, we have reported the preparation of sulfated polysaccharide NPs by using a new adapted technique, nanocoacervation, which produces NPs with lower MD and good PI, and uses CS or FC as a therapeutic substance in borax buffer.
The comparison of our systems with those described in the literature containing CT revealed that our system presents a lower MD, with an easier procedure, and that spent fewer reagents, with a cleaner process. The NPs containing CT and
FC presented higher activity than the FC free form at the same concentration in the coagulation tests. FC-based nanosystems were demonstrated to be biocompatible with Caco-2 cells (intestinal), in view of administration via oral route. Permeability studies across the Caco-2 monolayer showed a greater ability of the NPs to cross the intestinal epithelium when compared to the sulfated polysaccharide solution. This could be due to internalization via endocytosis, due to particle size and surface chemistry (zeta potential). This feature could suggest the possibility of oral administration of such systems.
Acknowledgments
This work was supported by Coordenação de Aperfeiçoamento de Pessoal de Nível Superior (CAPES) (6711/10-3). Edital CAPES Nanobiotecnologia 2008.
Disclosure
The authors report no conflicts of interest in this work.
References
- MouraoPAPereiraMSPavaoMSStructure and anticoagulant activity of a fucosylated chondroitin sulfate from echinoderm. Sulfated fucose branches on the polysaccharide account for its high anticoagulant actionJ Biol Chem19962713923973239848798631
- BeckerCFGuimaraesJAMouraoPAVerliHConformation of sulfated galactan and sulfated fucan in aqueous solutions: implications to their anticoagulant activitiesJ Mol Graph Model200726139139917307002
- MulloyBMouraoPAGrayEStructure/function studies of anticoagulant sulphated polysaccharides using NMRJ Biotechnol200077112313510674219
- OefnerPJChiesaCCapillary electrophoresis of carbohydratesGlycobiology1994443974127827401
- SuiWHuangLLWangJBoQBPreparation and properties of chitosan chondroitin sulfate complex microcapsulesColloids Surf B Biointerfaces2008651697318440788
- HondaSSeparation of neutral carbohydrates by capillary electrophoresisJ Chromatogr A19967201–23373518601200
- ParkWNaKPolyelectrolyte complex of chondroitin sulfate and peptide with lower pI value in poly(lactide-co-glycolide) microsphere for stability and controlled releaseColloids Surf B Biointerfaces200972219320019414243
- BaliJPCousseHNeuzilEBiochemical basis of the pharmacologic action of chondroitin sulfates on the osteoarticular systemSemin Arthritis Rheum2001311586811503140
- BarkalowFJSchwarzbauerJEInteractions between fibronectin and chondroitin sulfate are modulated by molecular contextJ Biol Chem19942696395739628307950
- DaceRMcBrideEBrooksKGanderJBuszkoMDoctorVMComparison of the anticoagulant action of sulfated and phosphorylated polysaccharidesThromb Res19978711131219253806
- MouraoPASPereiraMSSearching for alternatives to heparin: sulfated fucans from marine invertebratesTrends Cardiovasc Med19999822523211094330
- KariyaYMulloyBImaiKIsolation and partial characterization of fucan sulfates from the body wall of sea cucumber Stichopus japonicus and their ability to inhibit osteoclastogenesisCarbohydr Res200433971339134615113672
- DenuziereAFerrierDDomardAChitosan-chondroitin sulfate and chitosan-hyaluronate polyelectrolyte complexes. Physico-chemical aspectsCarbohydr Polym1996294317323
- Ganza-GonzalezAAnguiano-IgeaSOtero-EspinarFJBlanco MendezJBChitosan and chondroitin microspheres for oral-administration controlled release of metoclopramideEur J Pharm Biopharm199948214915510469933
- MuzzarelliRAAMuzzarelliCChitosan chemistry: relevance to the biomedical sciencesPolysaccharides 1: StructureCharacterization and Use2005186151209
- RinaudoMChitin and chitosan: properties and applicationsProg Polym Sci2006317603632
- SilvaHSRCSantosKSCRdFerreiraEIQuitosana: derivados hidrossolúveis, aplicações farmacêuticas e avançosQuímica Nova2006294776785
- LiuZHJiaoYPWangYFZhouCRZhangZYPolysaccharides-based nanoparticles as drug delivery systemsAdv Drug Deliv Rev200860151650166218848591
- OhJKLeeDIParkJMBiopolymer-based microgels/nanogels for drug delivery applicationsProg Polym Sci2009341212611282
- DudhaniARKosarajuSLBioadhesive chitosan nanoparticles: preparation and characterizationCarbohydr Polym2010812243251
- TakeuchiHYamamotoHKawashimaYMucoadhesive nanoparticulate systems for peptide drug deliveryAdv Drug Deliv Rev2001471395411251244
- VasiliuSPopaMRinaudoMPolyelectrolyte capsules made of two biocompatible natural polymersEur Polym J2005415923932
- KooOMRubinsteinIOnyukselHRole of nanotechnology in targeted drug delivery and imaging: a concise reviewNanomedicine20051319321217292079
- MohanrajVChenYNanoparticles – a reviewTrop J Pharm Res200651561573
- KreuterJNanoparticulate systems for brain delivery of drugsAdv Drug Deliv Rev2001471658111251246
- KreuterJNanoparticles – a historical perspectiveInt J Pharm2007331111017110063
- KumariAYadavSKYadavSCBiodegradable polymeric nanoparticles based drug delivery systemsColloids Surf B Biointerfaces201075111819782542
- MitraSGaurUGhoshPCMaitraANTumour targeted delivery of encapsulated dextran-doxorubicin conjugate using chitosan nanoparticles as carrierJ Control Release2001741–331732311489513
- El-ShabouriMHPositively charged nanoparticles for improving the oral bioavailability of cyclosporin-AInt J Pharm20022491–210110812433438
- CantonIBattagliaGEndocytosis at the nanoscaleChem Soc Rev20124172718273922389111
- HuangLLSuiWPWangYXJiaoQPreparation of chitosan/chondroitin sulfate complex microcapsules and application in controlled release of 5-fluorouracilCarbohydr Polym2010801168173
- SezerADCevherEHatipogluFOgurtanZBasALAkbugaJThe use of fucosphere in the treatment of dermal burns in rabbitsEur J Pharm Biopharm200869118919817951036
- ChenMCWongHSLinKJThe characteristics, biodistribution and bioavailability of a chitosan-based nanoparticulate system for the oral delivery of heparinBiomaterials200930346629663719767097
- BoddohiSAlmodovarJZhangHJohnsonPAKipperMJLayer-by- layer assembly of polysaccharide-based nanostructured surfaces containing polyelectrolyte complex nanoparticlesColloids Surf B Biointerfaces2010771606820137902
- CalvoPRemunan LopezCVila-JatoJLAlonsoMJChitosan and chitosan/ethylene oxide-propylene oxide block copolymer nanoparticles as novel carriers for proteins and vaccinesPharm Res19971410143114369358557
- JanesKAFresneauMPMarazuelaAFabraAAlonsoMJChitosan nanoparticles as delivery systems for doxorubicinJ Control Release2001732–325526711516503
- DouglasKLPiccirilloCATabrizianMEffects of alginate inclusion on the vector properties of chitosan-based nanoparticlesJ Control Release2006115335436117045691
- DeSJRobinsonDPolymer relationships during preparation of chitosan-alginate and poly-l-lysine-alginate nanospheresJ Control Release200389110111212695066
- SarmentoBRibeiroAVeigaFFerreiraDDevelopment and characterization of new insulin containing polysaccharide nanoparticlesColloids Surf B Biointerfaces200653219320217049214
- LeeHELeeMJParkCRPreparation and characterization of salmon calcitonin-sodium triphosphate ionic complex for oral deliveryJ Control Release2010143225125720026364
- LeeCTKungPHLeeYDPreparation of poly(vinyl alcohol)-chondroitin sulfate hydrogel as matrices in tissue engineeringCarbohydr Polym2005613348354
- CostaLSFidelisGPCordeiroSLBiological activities of sulfated polysaccharides from tropical seaweedsBiomed Pharmacother2010641212819766438
- Mendes-SilvaWAssafimMRutaBMonteiroRQGuimaraesJAZingaliRBAntithrombotic effect of Glycyrrhizin, a plant-derived thrombin inhibitorThromb Res20031121–2939815013279
- NishinoTNishiokaCUraHNagumoTIsolation and partial characterization of a novel amino sugar-containing fucan sulfate from commercial fucus vesiculosus fucoidanCarbohydr Res19942552132248181008
- SandriGBonferoniMCRossiSFerrariFBoselliCCaramellaCInsulin-loaded nanoparticles based on N-trimethyl chitosan: in vitro (Caco-2 model) and ex vivo (excised rat jejunum, duodenum, and ileum) evaluation of penetration enhancement propertiesAAPS Pharm Sci Tech.2010111362371
- GaumetMVargasAGurnyRDelieFNanoparticles for drug delivery: the need for precision in reporting particle size parametersEur J Pharm Biopharm20086911917826969
- LiuZGJiaoYPLiuFNZhangZYHeparin/chitosan nanoparticle carriers prepared by polyelectrolyte complexationJ Biomed Mater Res A200783380681217559117
- VauthierCCabaneBLabarreDHow to concentrate nanoparticles and avoid aggregation?Eur J Pharm Biopharm200869246647518396389
- IovuMDumaisGdu SouichPAnti-inflammatory activity of chondroitin sulfateOsteoarthritis Cartilage200816Suppl 3S141818667340
- AsimakopoulouAPTheocharisADTzanakakisGNKaramanosNKThe biological role of chondroitin sulfate in cancer and chondroitin-based anticancer agentsIn Vivo200822338538918610752
- YangHLiuSCaiHWChondroitin sulfate as a molecular portal that preferentially mediates the apoptotic killing of tumor cells by penetratin-directed mitochondria-disrupting peptidesJ Biol Chem201028533256662567620484051