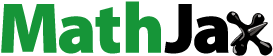
Abstract
Background
Oleanolic acid is a pentacyclic triterpene present in many fruits and vegetables, and has received much attention on account of its biological properties. However, its poor solubility and low bioavailability limit its use. The objective of this study was to encapsulate oleanolic acid into nanoliposomes using the modified ethanol injection method.
Methods
The liposomes contain a hydrophobic oleanolic acid core, an amphiphilic soybean lecithin monolayer, and a protective hydrophilic polyethylene glycol (PEG) coating. During the preparation process, the formulations described were investigated by designing 34 orthogonal experiments as well as considering the effects of different physical characteristics. The four factors were the ratios of drug to soybean phosphatidylcholine (w/w), cholesterol (w/w), PEG-2000 (w/w), and temperature of phosphate-buffered saline at three different levels. We identified the optimized formulation which showed the most satisfactory lipid stability and particle formation. The morphology of the liposomes obtained was determined by transmission electron microscopy and atomic force microscopy. The existence of PEG in the liposome component was validated by Fourier transform infrared spectrum analysis.
Results
The PEGylated liposomes dispersed individually and had diameters of around 110–200 nm. Encapsulation efficiency was more than 85%, as calculated by high-performance liquid chromatography and Sephadex® gel filtration. Furthermore, when compared with native oleanolic acid, the liposomal formulations showed better stability in vitro. Finally, the cytotoxicity of the oleanolic acid liposomes was evaluated using a microtiter tetrazolium assay.
Conclusion
These results suggest that PEGylated liposomes would serve as a potent delivery vehicle for oleanolic acid in future cancer therapy.
Video abstract
Point your SmartPhone at the code above. If you have a QR code reader the video abstract will appear. Or use:
Introduction
Oleanolic acid is a naturally occuring triterpenoid, widely distributed in the free or glucoside state in nearly 200 types of plant, including Mile swertia, Ligustrum lucidum, and Vitis vinifera. Oleanolic acid has many important biological actions, including anti-inflammatory, antioxidant, and antidiabetic properties.Citation1–Citation3 Recently, this compound has been noted for its antitumor effect.Citation4 However, being a poorly water-soluble drug (20°C, 4.61 mg/L), the solubility and dissolution rate of oleanolic acid in the gastrointestinal tract is poor, which limits its absorption and bioavailability.Citation5
To avoid these adverse effects and the inconvenience of native oleanolic acid, alternative formulations have been exploited for oral delivery of oleanolic acid. Polymeric microparticles and nanoparticles,Citation6 lipid nanoparticles, and liposomes have been investigated,Citation7,Citation8 and all of these formulations have improved the solubility of oleanolic acid. According to the Ostward-Freundlich and Noyes-Whitney equations, the saturation, solubility, and dissolution rate of a drug can be increased by reducing the particle size to increase the interfacial surface area.Citation9 Many approaches have been attempted to produce submicron drug powders. The common way of reducing particle size is to disrupt previously formed larger particles using milling methods. In addition, some new techniques, including high-pressure homogenization, have also been developed. However, these techniques show some disadvantages in practice, such as electrostatic effects and broad particle size distribution. In the past decade, nanoparticulate systems have shown the potential to modify drugs by decreasing their toxicity, sustaining their release, and increasing their efficacy, stability, and solubility. Citation10 Several nutraceuticals, including curcumin, coenzyme Q, and thymoquinone, have been packaged into nanoparticles and shown to be useful in “nanochemoprevention” and “nanochemotherapy”.Citation11 Among the most promising drug delivery systems, liposomes are an attractive option for advantageous drug transport.Citation8 They are self-assembled nanoparticles and have been used to encapsulate hydrophobic and hydrophilic drugs.Citation12 Liposomes have many advantages, including good biocompatibility, biodegradability, low toxicity, and controlled release of the entrapped drug.Citation13 In particular, small unilamellar vesicles enter into cells easily, and are beneficial for drug uptake. Liposomes with a diameter of less than 150 nm have been reported to be suitable for efficient drug delivery.Citation14,Citation15 Generally, small unilamellar vesicles can be produced by sonication, extrusion through membranes, and French press extrusion.Citation16
Despite the advantages of liposomes, they have some important drawbacks, including batch-to-batch variation in manufacturing, low drug loading efficiency, and poor stability.Citation17 Stability is one of the essential aspects of a liposomal drug, which has been the reason for developing formulations with specific steric stabilization. Therefore, various strategies have been developed, including modification of the surface of nanoparticles with phosphatidylinositol or polyethylene glycol (PEG).Citation18,Citation19 The latter, known as PEGylation, is one of the most successful approaches for prolonging nanoparticle circulation time in the blood, which leads to better targeting of the encapsulated drug, whilst exploiting the enhanced permeability and retention effect in tumors.Citation20 Recently, PEG-lipid conjugates have been incorporated into lipid bilayers,Citation21–Citation23 and it has been demonstrated that PEG-modified liposomes have significantly increased stability.Citation24
Lately, nanoscale formulations of oleanolic acid have been reported, and oleanolic acid has been successfully encapsulated into liposomes. Most of the work to date has focused on plain oleanolic acid liposomes, and there has been no report of preparation of PEG-modified liposomes. In our study, oleanolic acid was encapsulated in a nanoscale carrier to improve its bioavailability and take advantage of its small size and surface effects. Good solubility and diffusion permeability of oleanolic acid were obtained, along with a reduction in the effective drug dose and a dramatic increase in drug uptake. A modified ethanol injection method combined with sonication was used to prepare oleanolic acid liposomes modified by PEG-2000. Transmission electron microscopy and atomic force microscopy were used to observe the morphology of the oleanolic acid liposome formulation. The anticancer effects of native oleanolic acid and liposomal oleanolic acid on HeLa cells were demonstrated by MTT assay.
Materials and methods
Materials
Oleanolic acid and soybean phosphatidylcholine were purchased from Shenyang Tianfeng Biological Pharmaceutical Co, Ltd (Shenyang, China). Cholesterol, anhydrous ethanol, surfactant Tween-80, and PEG-2000 were purchased from Tianjin Guangfu Fine Chemical Research Institute (Tianjin, China). Sephadex G-75® and phosphate-buffered saline were obtained from Sigma Chemical Company (Henan, China). All reagents used were of at least analytical grade.
Preparation of PEGylated liposomal oleanolic acid
In this investigation, liposomes were prepared using the ethanol injection technique. Natural phospholipids showed high stability and biocompatibility, enabling production of lipid vesicles which were more resistant to oxidation.Citation25 Moreover, the cost of liposomal formulations based on natural phospholipids is usually lower than that of preparations containing synthetic phospholipids. Cholesterol was used as one of the membrane materials in the preparation of the liposomes in order to improve lipid packaging and membrane permeability characteristics.
Liposomes were prepared according to the ethanol injection method described by Justo and Moraes.Citation26 The method used for production of up to 10 mL of a liposome dispersion is as follows. In brief, oleanolic acid was dissolved in anhydrous ethanol to produce an ethanolic solution of oleanolic acid at a concentration of 0.5 mg/mL. For lipid injection, 9% (w/v) soybean phosphatidylcholine, 1.25% (w/v) cholesterol, and 0.1% (w/v) oleanolic acid were solubilized in 2 mL of anhydrous ethanol as the lipid phase, which was continuously stirred at 43°C. Tween-80 (0.2% [v/v] in the aqueous phase) and PEG-2000 were dissolved in 10 mL of phosphate-buffered saline (pH 6.5) in a water bath at 43°C as the aqueous phase. When the soybean phosphatidylcholine and cholesterol were completely dissolved in the ethanolic solution of oleanolic acid, the lipid phase was dripped into the aqueous phase under magnetic stirring, using a 4 μm diameter stainless steel needle attached to a 1 mL glass syringe. Residual ethanol in the primary emulsion was removed by a rotary evaporator at 45°C. The liposomal dispersion obtained was then subjected to probe ultrasonic testing using an LTD JY92-II device (Scientz Biotechnology Co, Zhejiang, China) in an ice-bath with a one-second pulse-on period and a one-second pulse-off period for 7 minutes. The probe ultrasonic frequency used in the experiment was around 100 W. Homogeneous and slightly opalescent suspensions were produced, and non-PEGylated liposomes were prepared using the same method. During the study, the ratios of drug to soybean phosphatidylcholine (w/w), cholesterol (w/w), and PEG-2000 (w/w), along with temperature of the phosphate-buffered saline were considered to be important factors for efficacy of the formulation. Furthermore, orthogonal experiments were designed at three levels ( and ). Of note is that the final concentration of ethanol in phosphate-buffered saline was kept constant at 2.5% (v/v). In addition, in order to improve the solubility of the preparations, the lipids suspended in ethanol were heated to 43, 48, and 53°C before injection. All the liposome suspensions were kept at 4°C until further testing.
Table 1 Levels of experimental factors
Table 2 Orthogonal experiment design and drug entrapment efficiency (%)
Determination of entrapment efficiency and drug content
Gel chromatography separation
A gel chromatographic column (1.0 cm × 25 cm) filled with Sephadex G-75 was used to separate the liposomal nanoparticle systems. A 1 mL sample of liposomal oleanolic acid suspension was added to the column, followed by elution using double-distilled water. Free drug remained in the gel. Liposomes travelling in the gel were collected from the first 6 mL, and the following 50 mL elution volume was the free oleanolic acid.
HPLC analysis
The original liposomes and the eluted liposomes were ruptured using an adequate volume of methanol, and the total drug content as well as drug content in the liposomal dispersion were analyzed respectively on an Agilent 1200 series system (Agilent, Santa Clara, CA). The chromatographic apparatus consisted of an Agilent Zorba × 300 SB-C18 column (5 μm, 250 mm × 4.6 mm) used at room temperature. The mobile phase, consisting of methanol and 0.1% acetic acid (88:12) (v/v), was maintained at a flow rate of 1.0 mL/minute. The ultraviolet detector wavelength was 215 nm and the injection volume was 20 μL. The peak area of response to the concentration of oleanolic acid was linear over the range 10–120 μg/mL (r = 0.9999). Drug entrapment efficiency (EE) and drug loading (DL) were calculated using Equationequations (1)(1) and Equation(2)
(2)
where Wentrapped is the analyzed weight of the amount of drug entrapped in the liposomes, Wtotal is the analyzed weight of drug in the liposomal dispersion, and Wlipids is the total weight of the lipid content.
Characterization of PEGylated oleanolic acid
Transmission electron microscopy
Morphological observation of PEGylated liposomal oleanolic acid was done using an transmission electron microscope (JEM-100CX/II, JEOL Ltd, Tokyo, Japan). Samples were diluted with double-distilled water and placed on a copper grid coated with carbon film and air-dried. They were then stained with 2% (w/v) tungstophosphoric acid. Finally, the samples were air-dried prior to placing them in the transmission electron microscope at room temperature.
Atomic force microscopy
Atomic force microscopy was also exploited to investigate the surface morphology of the liposomes in greater detail. Imaging and force measurement were conducted using a Nanoscope IIIa atomic force microscope from Digital Instruments (Bruker AXS Inc, Madison, WI) equipped with an E scanner, having a maximum scan area of 10 μm2, with scanning performed at room temperature. The scanner was calibrated following the standard procedures recommended by Digital Instruments. A Scan Asyst mode was used to obtain images of the liposomes. Just before analysis, the samples were appropriately diluted in double-distilled water, and 20 μL of the dispersion was deposited on mica. The mica was then air-dried on a clean bench. Freshly cleaved mica was mounted onto a stainless steel disc using a sticky tab (Latham, NY) and measurements were performed.
Fourier transform infrared spectrum analysis
The oleanolic acid liposomes were first freeze-dried to powder, and mannitol and sucrose at a mass ratio of 1:1 were used as lyoprotectants. For preparation, a small quantity of the sample was mixed with dry potassium bromide (KBr), and the mixture was then ground into fine powder using an agate mortar before pressing into a thin KBr pellet under a hydraulic press at 10,000 psi. Infrared spectra of liposomal oleanolic acid and PEG-2000 samples were obtained in transmission mode using a Fourier transform infrared (FTIR) Per kin-Elmer spectrometer model Spectrum GX from discs containing samples of the liposomes and KBr in the range of 4000 cm−1 to 400 cm−1. Characteristic peaks were recorded for the different samples.
Stability of PEGylated liposomal oleanolic acid
Liposomes have many advantages, but also have their limitations. In particular, they tend to adhere to each other and fuse to form larger particles in suspension. In our study, two methods were used to determine and evaluate their stability. First, according to their drug content, PEGylated liposomal oleanolic acid samples were placed in an electric blast-drying oven at 30, 40, and 50°C. Thereafter, they were kept for 5, 10, and 30 days, with their drug contents determined at these respective time points. Entrapment efficiency was evaluated for the PEGylated oleanolic acid liposomes at 20°C and 4°C on days 1 and 7, at which times leakage rates for the liposomes were also obtained.
In vitro drug release study
The rate of release of oleanolic acid liposomes in vitro was determined using a dynamic dialysis method in phosphate-buffered saline (pH 7.4). Briefly, the dialysis bags were pretreated in the usual way, and 1 mL of the oleanolic acid liposome solution was then placed into the dialysis bag (molecular weight cutoff 12–14 kDa). The liposome solution was then dialyzed against 150 mL of phosphate-buffered saline solution at 37°C ± 0.2°C using a rotational speed of 100 rpm. At scheduled time intervals, one of the dialysis bags was removed. Meanwhile, the same amount of release medium was added. Finally, the residual oleanolic acid content of the liposomes in the dialysis bags was determined by high-pressure liquid chromatography, as described earlier. Native oleanolic acid and non-PEGylated oleanolic acid liposomes were evaluated as controls. All experiments were performed in triplicate.
In vitro MTT cytotoxicity assay
The cytotoxic activity of the liposomes was evaluated using the MTT method with HeLa cells.Citation27,Citation28 Briefly, HeLa cells were seeded at a density of 5 × 104/mL in a 96-well flat-bottomed plate and incubated for 24 hours at 37°C in a 5% CO2 atmosphere. Oleanolic acid liposomes were dissolved and diluted in phosphate-buffered saline (pH 7.4), then filtered using a 0.22 μm sterile filter and injector. Various drug concentrations of 500, 250, 125, 62.5, and 31.25 μg/mL were obtained. Non-PEGylated oleanolic acid liposomes dissolved in phosphate-buffered saline at the same dilution were used as a control. Meanwhile, oleanolic acid was dissolved in dimethyl sulfoxide and diluted similarly with phosphate-buffered saline. It is noteworthy that the final concentration of dimethyl sulfoxide in the culture medium was controlled at less than 0.5% (v/v). The cells were cultured for 24 hours under the conditions mentioned above. The drugs were added into the appropriate wells, and cell viability was then analyzed using the MTT agent. Briefly, 10 μL of MTT solution was added to each well, and the cells were incubated for a further 4 hours. Then, 100 μL of formazan lysis buffer was added to dissolve any formazan crystals formed. Absorbance was then measured at 595 nm on a microplate reader. All experiments were performed in triplicate. Absorbance of untreated cells was considered to be 100% cell viability, and treated cell viability was calculated.
Results
Encapsulation efficiency of PEGylated oleanolic acid liposomes
PEGylated oleanolic acid liposomes prepared using the ethanol injection method were found to be a mildly translucent liposomal suspension. For all experiments using the orthogonal design, entrapment efficiency was 30%–98%. Consequently, we chose the optimized formulation to prepare blank and oleanolic acid-loaded liposomes. There were many factors to take into account in the preparation process, including prescription and technology factors. In this study, the ratios of drug to soybean phosphatidylcholine (w/w), cholesterol (w/w), and PEG-2000 (w/w), along with the temperature of the phosphate-buffered saline were identified to be the most influential factors (labeled as A, B, C, and D in ). Whilst considering the encapsulation efficiency as an important index, the four factors were investigated at three different levels. The L9 (3Citation4) orthogonal design is shown in and . The results of this experiment show that the ranking of the four factors in this experiment was B > C > A > D, the most effective factor being the ratio of drug to cholesterol, with the reactive temperature also having a slight influence. With regard to the individual factors, the appropriate ratio of drug to soybean phosphatidylcholine (w/w) was 1:15, the optimum ratio of drug to cholesterol (w/w) was 1:2, and the appropriate ratio of drug to PEG (w/w) was 1:1. Likewise, the optimal reactive temperature was 43°C. According to the analytical results, the optimal formulation should be A1B2C1D1.
Drug content
As shown in , the oleanolic acid content in the liposomal formulations was relatively high. A content of more than 98% was clearly observed at the three levels. In particular, the oleanolic acid content at level 3 was 102.37, which could be explained by the fact that, during the preparation procedure, part of the aqueous medium used to hydrate the oil phase was evaporated, resulting in an increased oleanolic acid concentration in the samples. The results indicate that drug loss in the preparation was not significant.
Table 3 Drug content of three batches
Characterization of PEGylated oleanolic acid liposomes
Morphological examination and particle size measurement of the PEGylated oleanolic acid liposomes were performed using a transmission electron microscope. shows the structures of PEGylated oleanolic acid liposomes with and without surfactant Tween-80. Mean particle size was approximately 100 nm on representative transmission electron microscopic images. Spherical particles with an obvious double-layer structure were observed. shows that the liposomes with Tween-80 had a characteristic liposomal dispersion, with few particles adhered to each other.
Figure 1 Transmission electronic microscopic images of PEGylated OA liposomes. (A) Formulation without Tween-80. (B) Tween-80 0.2% (v/v) added to preparation.
Abbreviations: OA, oleanolic acid; PEG, polyethylene glycol.
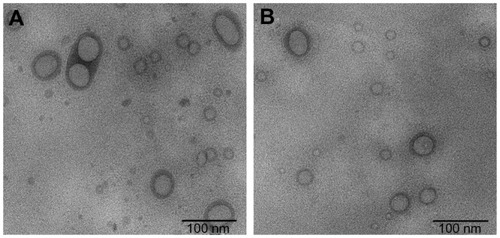
Atomic force micrographs () demonstrated that the size of the PEGylated liposomal oleanolic acid formulations was below 200 nm. Mean particle diameters without Tween-80 surfactant obtained by atomic force microscopy were around 168 nm (). The atomic force micrographs () also showed that only some of the particles adhered to each other when using soybean phosphatidylcholine, and some even aggregated together in bulk. Some particles seemed to be liposomes, judging from their relatively flat shapes, which may be the result of deformation during atomic force microscopy. The mean diameter of the oleanolic acid liposomes prepared from the formulation containing Tween-80 was 110 nm (), and it appeared that particle aggregation occurred infrequently among PEGylated oleanolic acid liposomes containing Tween-80.
Figure 2 Scan Asyst mode atomic force microscopic images of PEGylated OA liposomes. (A and B) Without Tween-80 and (C and D) with 0.2% (v/v) Tween-80 as a surfactant.
Abbreviations: OA, oleanolic acid; PEG, polyethylene glycol.
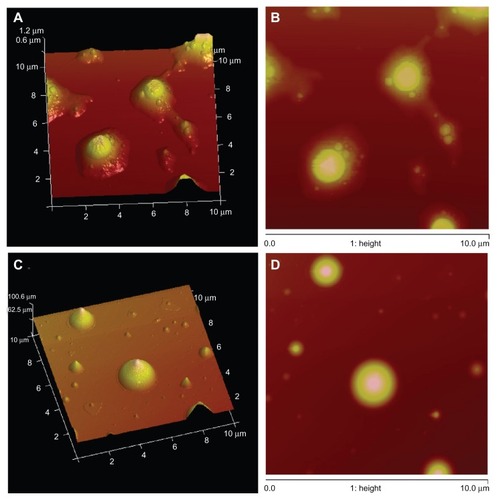
To confirm attachment of PEG to the oleanolic acid liposomes, the liposome formulations were investigated by FTIR spectrometry (). Broad strong bands between 3340 cm−1 and 3424 cm−1 were seen in the FTIR spectrum of PEG, which corresponds to the stretching vibrations of the intramolecular hydrogen bonds (υO–H) of PEG. Moreover, the typical absorption band at approximately 1120 cm−1 is attributable to the characteristic C–O–C stretching vibration of the repeated –OCH2CH2– units of the PEG backbone (). A similar result has been reported by Kassim et alCitation29 and Petrova et al.Citation30 Pure PEG also showed distinct peaks at about 1220, 1120, and 842 cm−1. Characteristic peaks of PEG were also evident in the PEG-coated liposomes, suggesting that PEG was successfully coated onto the liposomes.
Stability of PEGylated oleanolic acid liposomes
Stability of oleanolic acid liposomes is essential from the nanomedicine point of view. When liposomes are kept for a long time, some leakage of the encapsulated drug can occur. The stability of our PEGylated oleanolic acid liposomes was investigated in two ways. The results obtained using the first method are shown in . Our experience was that temperature was an important factor in the rate of leakage. The encapsulation efficiency of PEGylated oleanolic acid liposomes decreased as the temperature increased, and after 10 days, the encapsulation efficiency had decreased from 98.2% to 94.8%. Furthermore, when the liposomes were kept for 5–30 days at 50°C, their drug content showed a marked decrease.
Table 4 Drug contents of PEGylated OA liposomes in different temperatures after several days
The second method was based on the encapsulation efficiency of the nanoparticles. Their physicochemical stability is shown in . After 14 days, the encapsulation efficiency of non-PEGylated oleanolic acid liposomes had decreased to 70.2% at room temperature (20°C). However, the encapsulation efficiency of PEGylated oleanolic acid liposomes had decreased to 76.8%. Oxidation and hydrolysis of the lipids resulted in decomposition and aggregation of the liposomes, which also induced drug leakage. PEGylated oleanolic acid liposomes were stable at low temperature, although their encapsulation efficiency decreased slightly at 4°C, but their stability was significantly improved.
Table 5 Entrapment efficiency of PEGylated and non-PEGylated OA liposomes in different conditions
In vitro drug release study
The liposomal formulations as well as the native oleanolic acid showed faster release initially and slow release thereafter (). Moreover, it was observed that both PEGylated and non-PEGylated oleanolic acid liposomes had a slower release profile than pure oleanolic acid. From 2 to 10 hours, the leakage rate of pure oleanolic acid was markedly higher than for PEGylated oleanolic acid liposomes, and the non-PEGylated formulations showed an intermediate leakage rate. After 10 hours, the leakage rate of PEGylated oleanolic acid liposomes increased significantly, whereas that of pure oleanolic acid remained fairly steady. However, leakage from PEGylated and non-PEGylated preparations was consistently lower than that from pure oleanolic acid. These results indicate that oleanolic acid is released more slowly from the PEGylated liposomal formulation than from the pure oleanolic acid formulation, which could reduce drug toxicity.
MTT assay of antitumor activity
The in vitro antitumor effect of pure oleanolic acid and that of PEGylated and non-PEGylated oleanolic acid liposomes were studied by MTT assay (), using oleanolic acid as a model antitumor drug. With regard to viability, the HeLa cell retained different sensitivity to native oleanolic acid and the different kinds of oleanolic acid liposomes. With pure oleanolic acid, the cell viability was higher than for oleanolic acid-loaded liposomes, and had somewhat low antitumor activity. However, this activity increased significantly when cells were treated with oleanolic acid liposomes and PEGylated formulations. Cell numbers were reduced in a concentration-dependent manner. Moreover, it was demonstrated that antitumor activity was enhanced when oleanolic acid was prepared as a nanoscale medicine. In particular, surface-modified liposomes showed better inhibition of HeLa cells.
Discussion
Liposomes have been considered to be promising and valuable antitumor drug carriers due to their many advantages. Controlled drug delivery systems using liposomes can improve the biodistribution of existing anticancer drugs, and prolong drug accumulation in both tumor tissue and in the blood stream. The design of liposomes is important because their hydrophobic core should be capable of carrying highly insoluble anticancer drugs with high loading capacity (most chemotherapeutic drugs are hydrophobic). However, a surface-modified hydrophilic moiety provides steric protection and reduces macrophage uptake in vivo. One of the most widely used methods for enhancing the circulation properties of liposomes is incorporation of PEG within the surface membrane of liposomes.Citation19,Citation31,Citation32 The possible mechanism is that a hydrated PEG shell disturbs the interaction of liposomes with serum proteins, and significantly reduces opsonization and uptake by macrophages.Citation33
The water solubility and bioavailability of oleanolic acid is very low.Citation34 Presently, various new oleanolic acid formulations are being developed to overcome the problem of low oral bioavailability.Citation35 Oleanolic acid drop pills have been successfully obtained by Tang et al, through mixing homogeneously with PEG of different molecular weights.Citation36 However, there have been a few reports of exploiting oleanolic acid liposomes modified by PEG. Consequently, our hypothesis was that a hydrophilic polymeric coating (ie, PEG) could improve the longevity of oleanolic acid in the circulation and have slow-release properties due to stability of the drug-loaded liposomes.
In our study, drug content was determined after the liposomes were fabricated. The results provide some groundwork in the examination of entrapment efficiency. Morphological analysis of the formulated liposomes by transmission electron microscopy provided further evidence of successful incorporation of PEG into nanoparticles. Atomic force microscopy showed that the particle size was larger than that determined by transmission electron microscopy. There were two possible explanations for this difference. One reason is that the structure of the liposomes could be changed when atomic force microscopy was performed. In the process of observing the atomic force microscopic images of the formulations, the force of the probe was not always uniform; in addition, the dried liposome film was very soft. Tapping of the probe resulted in deformation of some liposomes and a larger particle size. Second, the mica plate used to support the samples was not completely flat, which might have induced a morphological change in the liposomes. The incorporation of PEG improves biocompatibility, and decreases the positive surface charge significantly, as reported by Quellec et al.Citation37 It has been considered generally that PEGylation forms a fixed aqueous layer on the surface of nanoparticles via interaction between PEG and water molecules, leading to reduced amounts of associated serum opsonins on the surface, thereby prolonging the circulation time of the nanoparticles.Citation38–Citation41 Because the Tween-80 used as a surfactant has a polyoxyethylene chain in its structure, molecules of Tween-80 located at the interface between the water and oil phase of the emulsion may have a similar function to that of PEG molecules. However, the detailed mechanisms behind these observations for a PEG emulsion, including the conformational and functional properties of Tween-80 on the surface of the emulsion, remain to be elucidated and will be the subject of our future studies.
Aggregation of liposomes may lead to leakage of inclusions.Citation42,Citation43 Therefore, stability is an essential problem for lipid vesicles.Citation44 After preliminary studies to find the best formulation for PEG liposomes, an in vitro release study was carried out to evaluate the rate of leakage from the preparations. The results indicate that PEG-modified liposomes had slow release and were stable compared with pure oleanolic acid. The configuration of PEG molecules on the surface of the liposome was important for steric repulsion in vitro because it determined the shielding efficiency. Moreover, PEGylated formulations showed advantages on cytotoxicity assay. The viability of cells treated with oleanolic acid liposomes performed better than oleanolic acid alone, but the pattern approximately resembled that of pure oleanolic acid.
Some earlier studies have been done on oleanolic acid liposomes and PEG-coated liposomes.Citation45,Citation46 However, there has not been any detailed investigation of PEGylated oleanolic acid liposomes, which have an important role in evading uptake by macrophages.Citation47,Citation48 It was found that high molecular weight PEG (2000 Da and higher) covalently attached to the lipid head group undergoes steric exclusion from the surface of the liposome. By dehydrating the lipid bilayer, high molecular weight PEG plays a significant role in the thermodynamic stability of liposomes.Citation49–Citation51 Also, hydrophilic PEG chains on the surface of the bilayers can adsorb more water molecules than those entrapped in the core.Citation52,Citation53 Thereby, the addition of PEG could enhance the encapsulating percentage and loading efficiency. PEG-2000 has been selected by many research groups as a surface-modifying polymer to develop novel liposome formulations.Citation54,Citation55 Liposomes modified using PEG-2000 showed feasibility and stable potential for loading drugs. Furthermore, it was demonstrated that introduction of PEG-2000 introduced concentricity of size distribution. In addition to protecting the vesicles against peritoneal macrophages, the presence of PEG-2000 reduces repulsion between the negative surfaces of the vesicles and the peritoneal mesothelium.Citation56
In our study, PEG-2000 was used to modify conventional liposomes. Formulations with steric stability were successfully obtained. We have confirmed the appropriate concentration of PEG to maximize its antitumor potential as well as optimizing the configuration of formulations. Therefore, we propose to have a mass ratio of oleanolic acid to PEG of 1:1 in our fabricated liposomes, which may be used as a very effective drug delivery system for antitumor drugs. The increased cytotoxicity of PEG-modified oleanolic acid liposomes may open a new pathway for the targeting of anticancer drugs, but there is still a pressing need for further in vivo studies to realize their full potential.
Acknowledgments
This work was financially supported by a research grant from the Chinese Ministry of Education Doctor Degree (20101333120011), grants from the Hebei Province Natural Science Fund (C2011203137, 11965152D), and a Chinese postdoctoral grant (480013).
Disclosure
The authors report no conflicts of interest in this work.
References
- TsurugaTChunYTEbizukaYSankawaUBiologically active constituents of Melaleuca leucadendron: inhibitors of induced histamine release from rat mast cellsChem Pharm Bull199139327632781726076
- BalanehruSNagarajanBProtective effect of oleanolic acid and ursolic acid against lipid peroxidationBiochem Int1991249819901776961
- ZhangYNZhangWHongDOleanolic acid and its derivatives: new inhibitor of protein tyrosine phosphatase 1B wth cellular activitiesBioorg Med Chem2008168697870518707891
- ShibataSChemistry and cancer preventing activities of ginseng saponins and some related triterpenoid compoundsJ Korean Med Sci200116SupplS283711748374
- LiuJPharmacology of oleanolic acid and ursolic acidJ Ethnopharmacol19954957688847885
- BilensoyEGurkaynakODoganALHincalAASafety and efficacy of amphiphilic beta-cyclodextrin nanoparticles for paclitaxel deliveryInt J Pharm200834716317017689901
- Arica YeginBBenoitJPLamprechtAPaclitaxel-loaded lipid nanoparticles prepared by solvent injection or ultrasound emulsificationDrug Dev Ind Pharm2006321089109417012121
- TorchilinVPRecent advances with liposomes as pharmaceutical carriersNat Rev Drug Discov2005414516015688077
- MosharrafMNystromCThe effect of particle size and shape on the surface specific dissolution rate of microsized practically insoluble drugsInt J Pharm19951223547
- ElaineMMGaryGLDrug nanoparticles: formulating poorly water-soluble compoundsToxicol Pathol200836434818337220
- NairHBSungBYadavVRKannappanRChaturvediMMAggarwalBBDelivery of anti-inflammatory nutraceuticals by nanoparticles for the prevention and treatment of cancerBiochem Pharmacol2010801833184320654584
- NaikSPatelDChuttaniKMishraAKMisraAIn vitro mechanistic study of cell death and in vivo performance evaluation of RGD grafted PEGylated docetaxel liposomes in breast cancerNanomedicine1122201110.1016/j.nano.2011.11.008
- PapahadjopoulosDAllenTMGabizonASterically stabilized liposomes – improvements in pharmacokinetics and antitumor therapeutic efficacyProc Natl Acad Sci U S A19918811460114641763060
- DrummondDCMeyerOHongKKirpotinDBPapahadjopoulosDOptimizing liposomes for delivery of chemotherapeutic agents to solid tumorsPharmacol Rev19995169174310581328
- TakeuchiHKojimaHYamamotoHKawashimaYPassive targeting of doxorubicin with polymer coated liposomes in tumor bearing ratsBiol Pharm Bull20012479579911456120
- SzokaFPapahadjopoulosDComparative properties and methods of preparation of lipid vesicles (liposomes)Annu Rev Biophys Bioeng198094675086994593
- MishraBPatelBBTiwariSColloidal nanocarriers: a review on formulation technology, types and applications toward targeted drug deliveryNanomedicine2010692419447208
- AllenTMHansenCPharmacokinetics of stealth versus conventional liposomes: effect of doseBiochim Biophys Acta199110681331411911826
- AllenTMHansenCMartinFRedemannCYau-YoungALiposomes containing synthetic lipid derivatives of poly(ethylene glycol) show prolonged circulation half-lives in vivoBiochim Biophys Acta1991106629362065067
- GabizonAShmeedaHBarenholzYPharmacokinetics of pegylated liposomal doxorubicin: review of animal and human studiesClin Pharmacokinet20034241943612739982
- BistaKBruchRFCovingtonAMSorgerAGerstmannTOttoAInvestigations of thermotropic phase behavior of newly developed synthetic PEGylated lipids using Raman spectro-microscopyBiopolymers2008891012102018615661
- SadzukaYKishiKHirotaSSonobeTEffect of polyethyleneglycol (PEG) chain on cell uptake of PEG-modified liposomesJ Liposome Res20031315717212855110
- ShimadaKMatsuoSSadzukaYDetermination of incorporated amounts of poly(ethylene glycol)-derivatized lipids in liposomes for the physicochemical characterization of stealth liposomesInt J Pharmacol2000203255263
- IshidaTHarashimaHKiwadaHLiposome clearanceBiosci Rep20022219722412428901
- JustoORMoraesAMAnalysis of process parameters on the characteristics of liposomes prepared by ethanol injection with a view to process scale-up: effect of temperature and batch volumeChem Eng Res Des201189785792 (Chemical engineering research and design. ISSN:0263-8762)
- JustoORMoraesAMKanamycin incorporation in lipid vesicles prepared by ethanol injection designed for tuberculosis treatmentJ Pharm Pharmacol200557233015638989
- KrollAPillukatMHHahnDSchnekenburgerJCurrent in vitro methods in nanoparticle risk assessment: limitations and challengesEur J Pharm Biopharm20097237037718775492
- PlumbJACell sensitivity assays: the MTT assayMethods Mol Med199928253021374024
- KassimAEkarmul MahmudHNMYeeLMHanipahNElectrochemical preparation and characterization of polypyrrole-polyethylene glycol conducting polymer composite filmsPac J Sci Technol20067103107 (pdf)
- PetrovaSMiloshevSMatevaRIlievISynthesis of amphiphilic PEG-PCL-PEG triblock copolymersJ Univ Chem Technol Metall200843199204 (pdf)
- BlimeGCevcGLiposomes for the sustained drug release in vivoBiochim Biophys Acta1990102991972223816
- KlibanovALMaruyamaKTorchilinVPHuangLAmphipathic poly(ethylene glycol) effectively prolong the circulation time of liposomesFEBS Lett19902682352372384160
- FurumotoKYokoeJOgawaraKEffect of coupling of albumin onto surface of PEG liposome on its in vivo dispositionInt J Pharm200732911011617000067
- ChenYJYangXLZhaoXLPreparation of oleanolic acid nanosuspensionJ Pharm Pharmacol200641924927
- YangRSuLQHuangXPreparation and quality evaluation of oleanolic acid self-microemulsionFood Drug2008104042 Chinese literature
- TangFLiHDDispersion of oleanolic acid drop pills and its evaluation in vitroChin Pharm J20084311671169 Chinese literature
- QuellecPGrefRPerrinLProtein encapsulation within polyethylene glycol-coated nanospheres. I. Physicochemical characterizationJ Biomed Mater Res19984245549740006
- ShimadaKMiyagishimaASadzukaYDetermination of the thickness of the fixed aqueous layer around polyethyleneglycol-coated liposomesJ Drug Target199532832898821002
- SadzukaYNakadeAHiramaREffect of mixed polyethyleneglycol modification on fixed aqueous layer thickness and antitumor activity of doxorubicin containing liposomeInt J Pharm200223817118011996821
- ShehataTOgawaraKHigakiKKimuraTProlongation of residence time of liposome by surface-modification with mixture of hydrophilic polymersInt J Pharm200835927227918486370
- YokoeJSakuragiSYamamotoKAlbumin-conjugated PEG liposome enhances tumor distribution of liposomal doxorubicin in ratsInt J Pharm2008353283418082345
- MatteucciMLThrallDEThe role of liposomes in drug delivery and diagnostic imaging: a reviewVet Radiol Ultrasound20004110010710779068
- Pedroso de LimaMCNevesSFilipeADuzgunesNSimoesSCationic liposomes for gene delivery: from biophysics to biological applicationsCurr Med Chem2003101221123112678796
- Bakker WoudenbergIAStormGWoodleMCLiposomes in the treatment of infectionsJ Drug Target199423633717704480
- ShengYLiuCYuanYLong-circulating polymeric nanoparticles bearing a combinatorial coating of PEG and water-soluble chitosanBiomaterials2009302340234819150737
- ZahrASDavisCAPishkoMVMacrophage uptake of core-shell nanoparticles surface modified with poly(ethylene glycol)Langmuir2006228178818516952259
- GbadamosiJKHunterACMoghimiSMPEGylation of microspheres generates a heterogeneous population of particles with differential surface characteristics and biological performanceFEBS Lett200253233834412482589
- PapisovMITheoretical considerations of RES-avoiding liposomes: molecular mechanics and chemistry of liposome interactionsAdv Drug Deliv Rev19983211913810837639
- PrievASamuniATiroshOBarenholzYThe role of hydration in stabilization of liposomes: resistance to oxidative damage of PEG-grafted liposomesJ Target Drug19986147167
- TiroshOBarenholzYKatzhendlerJPrievAHydration of polyethylene glycol-grafted liposomesJ Biophys19987413711379
- ZalipskySHarrisMJChemistry and biological applications of poly(ethylene glycol)Presented at ACS Symposium Series 680April 13–17, 1997San Francisco, CA
- ZuckerDAndriyanovAVSteinerARavivUBarenholzYCharacterization of PEGylated nanoliposomes co-remotely loaded with topotecan and vincristine: relating structure and pharmacokinetics to therapeutic efficacyJ Control Release1012201110.1016/j.jconrel.2011.10.003
- KaminskasLMMcLeodVMKellyBDA comparison of changes to doxorubicin pharmacokinetics, antitumor activity, and toxicity mediated by PEGylated dendrimerNanomedicine2012810311121704192
- BrusaPArpiccoSStellaBDosioFCattelLImmordinoMLPreparation, characterization, cytotoxicity and pharmacokinetics of liposomes containing docetaxelJ Control Release20039141742912932719
- NaikSPatelDSurtiNMisraAPreparation of PEGylated liposomes of docetaxel using supercritical fluid technologyJ Supercrit Fluids201054110119
- DadashzadehSMirahmadiNBabaeiMHValiAMPeritoneal retention of liposomes: effects of lipid composition, PEG coating and liposome chargeJ Control Release201014817718620800629