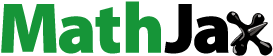
Abstract
Background
Recent interest in biocompatible polymeric microneedles for the delivery of biomolecules has propelled considerable interest in fabrication of microneedles. It is important that the fabrication process is feasible for drug encapsulation and compatible with the stability of the drug in question. Moreover, drug encapsulation may offer the advantage of higher drug loading compared with other technologies, such as drug coating.
Methods and results
In this study, we encapsulated a model protein drug, namely, bovine serum albumin, in polymeric microneedles by photolithography. Drug distribution within the microneedle array was found to be uniform. The encapsulated protein retained its primary, secondary, and tertiary structural characteristics. In vitro release of the encapsulated protein showed that almost all of the drug was released into phosphate buffered saline within 6 hours. The in vitro permeation profile of encapsulated bovine serum albumin through rat skin was also tested and shown to resemble the in vitro release profile, with an initial release burst followed by a slow release phase. The cytotoxicity of the microneedles without bovine serum albumin was tested in three different cell lines. High cell viabilities were observed, demonstrating the innocuous nature of the microneedles.
Conclusion
The microneedle array can potentially serve as a useful drug carrier for proteins, peptides, and vaccines.
Video abstract
Point your SmartPhone at the code above. If you have a QR code reader the video abstract will appear. Or use:
Introduction
Transdermal drug delivery has seen a rapid growth in the past three decades. Since the approval of a scopolamine transdermal patch in 1979, researchers have developed a variety of transdermal dosage forms, leading to 21 transdermal drugs being approved by the US Food and Drug Administration.Citation1 Simultaneously, the growth of biotechnology has produced a surge in the number of protein and peptide drugs available for human use.Citation2 Most of these protein drugs have been conventionally delivered via intravenous or subcutaneous routes, necessitating frequent injections, thus reducing patient compliance. Oral delivery of proteins, peptides, and vaccines is envisaged, but there are significant hurdles due to the adverse environment these biomolecules encounter in the gastrointestinal tract.Citation3 Transdermal delivery has evolved as an interesting alternative for protein drug therapy because it circumvents the inherent challenges associated with oral and parenteral routes. However, the skin’s natural barrier prevents these macromolecules from diffusing through the skin.
To enhance their permeation, active permeation methodologies have been used. Chemical penetration enhancers as well as physical techniques like electroporation, ultrasound, and iontophoresis have seen some success.Citation4,Citation5 Microneedles have been shown to disrupt the stratum corneum actively by creating micron-sized pores and hence enhancing the flux of macromolecules.Citation6–Citation8 They offer the advantage of delivering a drug without impinging on the underlying nerves.Citation9
Drugs have been delivered using microneedles by three different modes. Firstly, coating onto microneedle shafts,Citation10,Citation11 and secondly, pretreating the skin with microneedles to create pores, followed by application of conventional dosage forms.Citation12,Citation13 With the development of polymeric microneedles, a third approach was developed whereby drugs could be encapsulated in the polymeric matrix and released from the polymer upon application.Citation14 Encapsulation of drugs within the polymeric core offers the advantage of a higher drug loading in one convenient formulation, omitting multiple steps that would otherwise be required. Hence encapsulation of drugs within the microneedles has received most attention from transdermal drug delivery scientists in the past few years.Citation15–Citation17
Encapsulation of proteins inside microscale and nanoscale particles has been studied previously using various approaches, such as double emulsion,Citation18 electrospray,Citation19 self-assembly, Citation20 and microfabrication.Citation21 For example, lipid nanocarriers of the self-assembled type can be used to encapsulate proteins, peptides, and nucleic acids with tunable sizes and morphologies.Citation20,Citation22 These three-dimensional nanostructures can be obtained by homogenizing a mixture of lipids hydrated with protein solutions.
Similarly, therapeutic proteins such as insulin and vaccines can be encapsulated in microneedles, from which release of these proteins has been demonstrated upon insertion into animal skins in vivo.Citation14,Citation23,Citation24 A compilation of proteins and vaccines that can be potentially delivered through the skin has been reviewed by Prausnitz and Langer.Citation4 Drugs released from microneedles can form a depot from where they can be absorbed into the systemic circulation or lymphatic vessels. Drugs have been encapsulated in microneedles by various techniques, including micromolding the drug-containing polymer in prefabricated master structuresCitation17,Citation25 or dipping polypropylene tips in drug-laden polymer solutions.Citation24 Photo cross-linking of drug-laden polymer precursors filled in preformed molds using ultraviolet light has also been reported.Citation14,Citation23
However, the reported fabrication approaches used for these protein-laden microneedles involve the use of high temperatures, vacuum, or prolonged exposure to ultraviolet light which may be potentially harmful to biological drugs. High temperatures (140°C–160°C) used for micromolding of sugar microneedles have resulted in a significant loss of drug content.Citation26 Casting methods used by other groupsCitation25,Citation27 utilize polymers or sugar derivatives requiring a concentration of hydrogel using high temperatures and vacuum which may have deleterious effects on fragile protein molecules.Citation26 A newer process reported recently involves prefabrication of a female purple sand mold at high temperatures and subsequent fabrication of needles by vacuum suction and freeze-drying.Citation28 These challenging conditions may not be ideal for a drug delivery system aimed to encapsulate fragile molecules.
We have recently developed a photolithography-based method utilizing low exposure to ultraviolet light,Citation29 which can be potentially applied for protein encapsulation. We selected bovine serum albumin (BSA) as a model protein. To ensure that the encapsulated material retains its structure and activity, we tested the stability of the encapsulated BSA by analyzing its primary, secondary, and tertiary structural features. This is relevant for therapeutic proteins and peptide drugs like insulin, desmopressin, and vaccines, such as influenza vaccine. To the best of our knowledge, this is the first report detailing exhaustive stability testing for protein encapsulation in microneedles. The in vitro release of the BSA protein was tested in phosphate-buffered saline. In vitro permeation of the encapsulated BSA through rat skin was also tested. In addition, we report the in vitro biocompatibility of microneedles using three different cell lines to assess the toxicity of the polymeric microneedles for skin applications.
Materials and methods
Materials
Poly (ethylene glycol) diacrylate (PEGDA) (Mn = 258), 2-hydroxy-2-methyl propiophenone, and BSA were purchased from Sigma-Aldrich (St Louis, MO). 3-(4,5-dimethylthiazol-2-yl)-2,5-diphenyl tetrazolium bromide (MTT) and dimethyl sulfoxide were purchased from MP Biomedicals (Cleveland, OH). A CytoTox-ONE™ homogeneous membrane integrity assay kit was bought from Promega (Madison, WI). Sodium azide was purchased from Alfa Aesar (Lancaster, UK). BSA Texas red conjugate was bought from Molecular Probes, Invitrogen (Orlando, FL). All other chemicals were of analytical grade and used as received.
Fabrication and characterization of polymeric microneedles
The microneedles were fabricated by a photolithographic method we developed previously.Citation29 Briefly, PEGDA containing 0.5% v/v of 2-hydroxy-2-methyl propiophenone, hereinafter referred to as “prepolymer” solution, was exposed to a high intensity (20.9 W/cm2) ultraviolet light source (Exfo Omnicure, Quebec, Canada) to form the backing layer. In a similar step, the prepolymer solution was pipetted onto this backing layer and exposed to ultraviolet light through a specifically patterned photomask. The microstructures thus formed, due to preferential exposure of the prepolymer solution in transparent regions of the photomask, represented micron-sized rods, defined as “microneedles”. Excess unpolymerized prepolymer solution was washed away using purified water and the microneedles left to dry in air. The geometric characteristics of the microneedles (length, base, and tip diameter) were studied using an SMZ-1500 stereomicroscope (Nikon, Tokyo, Japan).
Incorporation and uniform drug distribution in microneedles
BSA Texas red conjugate was incorporated into the microneedle backing layer and shafts at a concentration of 0.045% w/w in the prepolymer solution to ascertain uniform distribution of drug in the polymerized microneedles. The fabricated microstructures were then imaged using a Nikon A-1R confocal microscope to observe the fluorescence intensity at various areas of the backing layer and various lengths of a microneedle shaft. The fluorescence intensity was calculated using Nikon NIS elements BR 3.1 analytical software. Microneedle arrays were also imaged with a Nikon SMZ-1500 stereomicroscope.
Stability tests for BSA in microneedles
Primary structure stability
Sodium dodecyl sulfate-polyacrylamide gel electrophoresis was carried out using Laemmli’s method to assess the effect of ultraviolet-initiated photopolymerization on the conformational stability of BSA.Citation30 It was performed by casting 10% running gel and 5% stacking gel. Each formulation, containing 10 μg of protein sample as determined by bicinchoninic acid protein assay (Pierce, Rockford, IL), was mixed with an equal quantity of Laemmli sample buffer and 5% of β-mercaptoethanol. The solutions were heated at 100°C for 2 minutes after which they were loaded onto a comb stacked on the gel cast in an electrophoresis cell. The gel was run at 100 volts for 2.5 hours. After removal from the electrophoresis cell, the gel was stained with Coomassie brilliant blue R-250 staining solution for 2 hours on an orbital shaker. The excess stain was removed by a destaining solution (20% methanol, 10% glacial acetic acid, 70% water) overnight and the gel was imaged using a Samsung ST-550 digital camera (Seoul, South Korea).
Secondary structure stability
Circular dichroism spectroscopy was performed on the samples to evaluate the secondary structural characteristics of BSA in the fabricated microneedles. The analysis was performed using a Jasco J-810 spectropolarimeter (Tokyo, Japan) with a 1 mm light path quartz cell (Hellma, Müllheim, Germany). Data were acquired at a bandwidth of 0.1 nm with a scan speed of 50 nm per minute and a response time of 8 seconds. The samples and standard BSA solution were scanned over the wavelength range of 240–200 nm. The microneedle release samples were first filtered using microcentrifugal concentrators, (30 kDa cutoff, Vivaspin 20, General Electric, Addlestone, Surrey, UK) to separate the protein from the polymer. The samples were compared with a standard solution of BSA, and BSA degraded using heat (75°C) and acidic conditions (pH 2) was used as a control.Citation31,Citation32 The average value of triplicate measurements was used to plot the curve of mean residue ellipticity (in degrees cm2 dmol−1) to wavelength. The experimental data acquired from the spectropolarimeter were analyzed using the DichroWeb browser and a deconvolution algorithm (K2d) to calculate the mean residue ellipticity and percentage of alpha helix.Citation33–Citation35
Tertiary structure stability
In order to evaluate tertiary structural changes in the protein conformation, their fluorescence spectra were analyzed. A standard BSA solution at a concentration of 0.026 mg/mL in purified water was prepared to compare the spectral data with the BSA released from the microneedle samples. The emission spectra were studied in the range of 300–400 nm at a fixed excitation wavelength of 280 nm using a Hitachi F-7000 fluorescence spectrophotometer (Hitachi, Japan). Similar to the circular dichroism experiments, heat-degraded and acid-degraded samples were used as a control. The fluorescence intensities were plotted against wavelength as an average of triplicate measurements.
In vitro release of BSA from microneedles
BSA was encapsulated in the microneedles at three different concentrations (0.5%, 0.8%, and 1.3% w/w BSA in prepolymer solution) to obtain microneedle arrays containing 0.4–1.6 mg of the protein. The in vitro release was determined by suspending the microneedle arrays in 15 mL of 1 × phosphate buffered saline at 37°C. Periodically, the release medium was withdrawn completely and replaced with 15 mL of fresh medium to maintain sink conditions. The collected samples were kept at 4°C until analysis. The protein concentration in the release samples was analyzed by a bicinchoninic acid protein assay kit. Each concentration was analyzed in triplicate and the mean value was used for analysis. The cumulative amount in mg and the percentage of BSA released were plotted against time.
In vitro permeation through rat skin
In vitro permeation studies were carried out in water jacketed horizontal diffusion cells (TK-6H1, Shanghai Kai Kai Science and Technology Co Ltd, Shanghai, China). The skin was hydrated in the receptor solution (1 × phosphate-buffered saline with 0.005% v/v sodium azide) overnight. The skin was placed stretched on ten layers of Kimwipes (Kimberly-Clark, Roswell, GA) to provide tissue-like mechanical support. Citation36 Microneedles containing 0.7%, 1.42%, and 1.85% w/w BSA were applied to abdominal rat skin after removing the subcutaneous fat. Microneedles containing no BSA were used to blank the inherent protein released from the skin. BSA dissolved in propylene glycol was used to compare the enhancement of BSA permeation by microneedles over passive diffusion. The microneedle array was secured on the skin using scotch tape and the skin was placed between the donor and receptor compartments. The receptor compartment was filled with 4.5 mL of receptor solution, which was continuously stirred at 250 rpm using a Teflon-coated magnetic stirrer. At each sampling point, 1 mL of receptor solution was withdrawn and replaced with fresh receptor solution. The collected samples were stored at 4°C until they were analyzed. All samples were centrifuged at 10,000 rpm for 5 minutes and the supernatant was collected for analysis. The concentration of permeated BSA was determined by the ultraviolet A215–A225 method.Citation37 Each sample was analyzed in triplicate. The cumulative amount of drug permeated per unit area was plotted against time.
In vitro biocompatibility of polymeric microneedles
Biocompatibility of the microneedle materials was assessed by the viability of three different cell lines using colorimetric determination of mitochondrial succinate dehydrogenase activity using the MTT assay.Citation38 Human dermal fibroblasts, human adult low calcium high temperature (HaCaT) keratinocytes, and human embryonic kidney (HEK293) cells were used to assess the toxicity of the polymer used in fabricating the microneedles. The cells were grown in Dulbecco’s modified Eagle’s medium supplemented by 10% fetal bovine serum and 1% penicillin-streptomycin solution. After the cells had achieved 80%–90% confluency, they were trypsinized and counted. Cells (1 × 104 cells/well) were plated into 96-well microtiter plates (Corning, NY) in 200 μL of growth medium. After 24 hours of plating, 20 μL of polymer extracts (prepared by extracting the polymer from fabricated microneedles in 1× phosphate-buffered saline at 37°C for 24 hours) were added to each well. Positive control consisted of wells containing 20 μL 1× phosphate-buffered saline. The plates were incubated at 37°C in humidified 5% CO2 for 24, 48, and 72 hours. The medium was aspirated at the respective analysis point, and 20 μL of MTT solution (5 mg/mL in phosphate-buffered saline) was added to each well, followed by 200 μL of growth medium. The plates were incubated for 4 hours at 37°C. After 4 hours, the medium was aspirated again and 150 μL of dimethyl sulfoxide was added into each well to dissolve the formazan crystals formed, with the aid of a plate shaker operated at 100 rpm. The colorimetric assay was carried out by measuring the absorbance at 595 nm using a Tecan 2000 microplate reader (Tecan, Germany). The cell viabilities were calculated as a percentage of the control.
The toxicity of the polymer was also assessed by analyzing the amount of lactate dehydrogenase released from the membranes of damaged cells.Citation39 The cells were plated in a similar manner as described above and treated with polymer extract and phosphate-buffered saline. Maximum lactate dehydrogenase release was achieved by treating the cells with the lysis solution (9% w/v Triton X-100) provided by the manufacturer. The assay was performed according to the manufacturer’s protocol. The percentage toxicity was calculated using the following equation, where PolymerLDH, VehicleLDH, and TritonLDH represent the respective fluorescence values obtained from wells treated with polymer, phosphate-buffered saline, and Triton X-100.
Statistical analysis
The graphs were plotted using Microsoft Excel 2007. All experiments were performed at least three times and the data are reported as the mean ± standard deviation. Statistical analysis of the data was performed using PASW Statistics 18 Software (SPSS Inc, Chicago, IL). Comparison amongst groups was made by analysis of variance. The difference was considered to be statistically significant at P < 0.05.
Results and discussion
Fabrication and characterization of microneedles
The microneedles were found to have an average length of 820 μm, a base diameter of 300 μm, a center to center spacing between the needles of 1500 μm, and an average tip diameter of 140 μm. The microneedle patch consisted of an array of 8 × 8 needles spread over an area of 1.44 cm2.
Incorporation and uniform drug distribution in microneedles
Drug incorporation in the polymeric matrix prior to microneedle formation allows for higher drug loading as opposed to coating the drug molecules on the fabricated microneedles. In this study, we could achieve up to 1.6 mg of BSA per microneedle array. Drug encapsulation in polymers has been used previously with other polymers, namely, polyvinyl pyrrolidone and its copolymer with methacrylic acid,Citation23 copolymer of methylvinylether and maleic anhydride,Citation40 and polyvinyl alcohol.Citation41 However, our method circumvents the long ultraviolet exposure and extensive preprocessing required for microneedle formation using the previous methods, which may impact protein stability. Moreover, our process does not involve any mold-based or template-based processing, potentially avoiding interactions between mold or template material and fragile protein molecules.
An important aspect of a drug delivery system is the uniform distribution of drug in the carrier matrices. To test for uniformity, we encapsulated BSA Texas red conjugate to visualize the distribution of the protein inside the backing and the shafts of the microneedle array. The BSA Texas red conjugate shows peak excitation (λex) and emission (λem) at 596 nm and 615 nm, respectively,Citation42 which can be quantified using fluorescence microscopy. We employed confocal imaging as a tool to image the microneedle sample to assess the fluorescence distribution at different areas and depths of the microstructure. As observed from , fluorescence is distributed across different areas of the backing layer in a uniform pattern (P > 0.05). However, as the microneedle length increased from the bottom of the array (length 10 μm) to the microneedle tip (length 700 μm), the fluorescence intensity was observed to decrease gradually from 377 to 3.1 (). This can be attributed to the inherent microneedle geometry because the microneedle shafts are broader at the base than at the tip, owing to less drug being encapsulated in the tip region of the microneedles. Drug distribution among different microneedles in an array was constant, with the majority of the microneedles showing a uniform fluorescence (). This is expected to ensure uniform drug delivery over the patch area of 1.44 cm2. shows a stereomicroscope image of a complete microneedle array, showing that the drug is evenly distributed.
Figure 1 Encapsulation of bovine serum albumin Texas red conjugate (TR-BSA) in polymeric microstructures shows uniform distribution. Uniformly distributed TR-BSA in (A) microneedle backing and (B) microneedle shaft (C) microneedle array. Quantitative estimation of fluorescence intensity shows uniform distribution over (D) different areas of the backing layer (n = 3), (E) different length on a microneedle shaft (n = 6) and (F) different microneedles of an array.
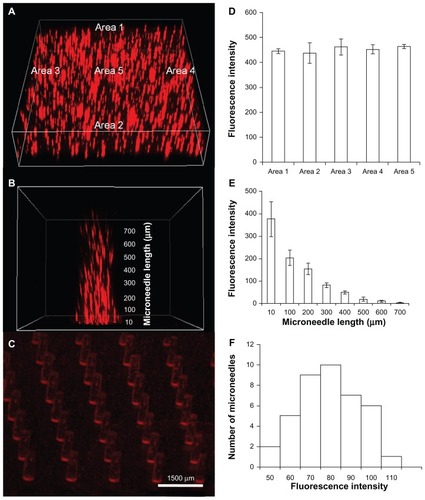
Stability tests for BSA in microneedles
Microneedles have been considered as a useful drug delivery system to deliver therapeutic peptides, proteins, and vaccines.Citation4 Because the biological function of a protein is dependent on its conformation, it is imperative to design a dosage form which does not adversely affect the stability of these fragile molecules. In our fabrication process, we used an ultraviolet light-based photo cross-linking method for creating polymeric microstructures. Ultraviolet light has been previously reported to cause protein denaturation and structural changes in the primary, secondary, and tertiary structure of proteins.Citation43 We studied these three structural features by investigating sodium dodecyl sulfate polyacrylamide gel electrophoresis, circular dichroism spectra, and fluorescence intensity measurements of BSA encapsulated in the microneedles. While the results here demonstrate that the conformation of BSA was maintained throughout the fabrication process and the subsequent release experiments, the structural and biological properties of proteins other than BSA will depend on individual protein characteristics.
Sodium dodecyl sulfate polyacrylamide gel electrophoresis has been the most common method used for the separation of proteins and determination of protein molecular weight.Citation44,Citation45 It has been used previously to determine the structural integrity of BSA in microspheres.Citation46 In this study, we used sodium dodecyl sulfate polyacrylamide gel electrophoresis to determine the amino acid sequence of BSA and analyze any deleterious effect of ultraviolet radiation on the protein (). BSA released from the formulation was compared with a freshly prepared solution and a protein standard marker. The single line of bands appearing at 66 kDa suggests that the protein was stable during the fabrication process and remained stable in the dosage form for a period of 72 hours. There were no other bands observed during the electrophoretic separation, providing evidence against any protein aggregation to form dimer or multimer or fragmentation to smaller subunits, indicating the primary structure of BSA remained intact during the fabrication process.
Figure 2 Sodium dodecyl sulphate-polyacrylamide gel electrophoresis images of protein standard marker, bovine serum albumin standard and bovine serum albumin released from microneedles after 24, 48, and 72 hours.
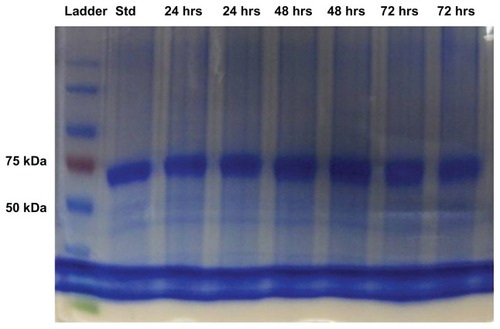
The secondary structure of BSA was assessed by circular dichroism. BSA contains 67% of α-helix, 10% of turn, and no β-sheet.Citation31 The far ultraviolet circular dichroism spectrum (260–200 nm) has been used to characterize the structural stability of BSA.Citation47 The α-helix structure is indicated by two negative peaks at 208 nm and 222 nm with a minimum peak at 208 nm, which is attributed to n → π* and π → π* transition of the α-helix structure.Citation48,Citation49 It was observed that the secondary structure of BSA encapsulated in the microneedles was similar to a freshly prepared solution of BSA, as shown in . The degraded BSA used as a control showed significantly lower ellipticity values than the standard BSA and microneedle release samples. The percentage of α-helix from the microneedle release samples was consistent with the reported amount of helix in the native BSA structure (about 67%).Citation50 The percentage of α-helix in the BSA released from the microneedle samples was comparable with the standard (P > 0.05, ) and significantly different from heat-denatured and acid-denatured BSA samples (P < 0.05). Circular dichroism analysis has been similarly reported for lysozyme.Citation25 These results demonstrated that secondary structural integrity of BSA was maintained during ultraviolet-dependent photopolymerization.
Figure 3 Circular dichroism analysis to assess the stability of encapsulated bovine serum albumin (BSA). Stability of BSA released from microneedles after storage for 3 days at 37°C is compared with a freshly prepared BSA solution and BSA degraded by heating at 75°C and under acidic conditions, pH 2 (A) mean residue ellipticity and (B) percentage of alpha-helix. All results confirmed the alpha helix structure of BSA was preserved during encapsulation and release over a period of 3 days.
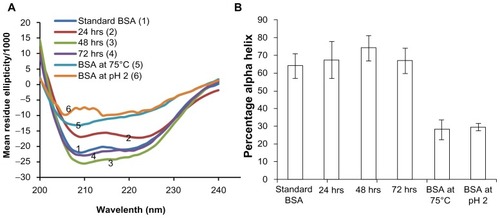
Because proteins contain aromatic amino acids like tyrosine, tryptophan, and phenylalanine, which are inherently fluorescent, fluorescence spectra and intensity can be used as a marker of protein structural stability. Tryptophan is the most dominant fluorophore and displays the largest extinction coefficient. Thus, the emission spectra of proteins can be measured at a fixed excitation wavelength of 280 nm.Citation51 The emission maximum of tryptophan in water is observed around 350 nm and is dependent on the polarity of the solvent. BSA emission spectra were scanned between 300 nm and 400 nm and an emission maximum was observed at 338 nm for all the release samples and a standard solution of BSA (). This is in accordance with previously reported resultsCitation52 and demonstrates the feasibility of our fabrication process for retaining protein stability in the microneedles. On the other hand, BSA samples denatured using heat or acid did not show any fluorescence (excitation wavelength 280 and emission wavelength 300–400 nm).
Figure 4 Fluorescence spectroscopic analysis to assess the tertiary structure of encapsulated bovine serum albumin (BSA). Stability of BSA released from microneedles after storage for 1–3 days at 37°C is compared with freshly prepared BSA solution and BSA degraded by heating at 75°C and under acidic conditions, (pH2) by analyzing the emission spectra of BSA. Peak BSA emission wavelength was found to be similar for all samples. No fluorescence was observed in degraded BSA samples.

The analysis of primary, secondary, and tertiary structural stability of BSA demonstrated the microneedles to be a potentially useful carrier for protein drugs. The ultraviolet photo cross-linking did not significantly alter the structural properties of BSA, possibly due to the short time of exposure.
In vitro release of BSA from microneedles
The release profile of BSA is depicted in (percentage release) and (cumulative amount). We used phosphate-buffered saline (pH 7.4) as a release medium because it closely resembles extracellular fluids and plasma. It was observed that 80%–100% of the protein encapsulated in the microneedle array was released within a period of 6 hours. Most of the protein was encapsulated in the microneedle backing layer (about 90%) and the needles owing to their micron-scale geometry containing less drug. Similar release kinetics were observed in previous reports.Citation27
Figure 5 Release profile of bovine serum albumin encapsulated in microneedles over a period of 6 hours. (A) Percentage amount and (B) cumulative amount released.

The transient pores created by microneedles and diffusion of the protein through the polymeric layer to the epidermal and dermal regions to create a depot of protein drugs have been implicated.Citation10,Citation53 The drugs can be subsequently absorbed into the blood stream or lymphatic circulation.Citation11,Citation54 It is expected that the protein released will accumulate in the subepidermal tissues and be rapidly absorbed from the highly vascularized regions lying underneath.
In vitro permeation through rat skin
Microneedles increased the amount of BSA permeated as compared with passive diffusion of BSA. Because BSA is a large molecule (66 kDa), it cannot diffuse passively through the skin. shows the increase in the cumulative amount permeated per unit area on microneedle application as compared with a propylene glycol solution of BSA, which showed practically no permeation at the end of 48 hours. The amount permeated in the case of microneedles increased with the increase in the encapsulated amount of BSA. The permeation curve resembles the in vitro release profile observed in phosphate-buffered saline, with an initial burst-release followed by a slow-release phase. A similar BSA permeation pattern has been reported previously.Citation37 Other protein molecules, like fluorescein isothiocyanate-labeled BSA and insulin, incorporated in polymeric microneedles have also shown such burst-release patterns.Citation40 Such permeation profiles are common for other highly water-soluble drugs, such as calcein (log P = −5.02). However, we observed in our previous studies that relatively lipophilic drugs (eg, rhodamine B, log P = 2.43) do not show a burst in permeation and their absorption is somewhat limited by interaction with stratum corneum lipids.Citation29
Figure 6 In vitro permeation through rat skin. (A) Percentage amount and (B) cumulative amount permeated through rat skin when applied with a microneedle patch (containing 0.71%–1.85% w/w bovine serum albumin) or a propylene glycol solution of bovine serum albumin over a period of 48 hours.
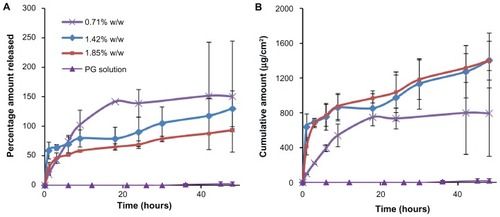
The A215–A225 method has been previously used in determining the BSA concentration in in vitro permeation studies.Citation37 The concentration of BSA is a linear function of the difference between extinction at 215 nm and 225 nm.Citation55 The method is sensitive for protein concentrations as low as 5 ng/mL, which are usually expected in in vitro permeation studies. The protein concentration was calculated using the following equation:
Microneedles containing no BSA were used as a control to minimize any absorption from the dissolved polymer. When lower amounts of BSA were encapsulated, the permeation curve demonstrated a plateau at nearly 18 hours, because most of the drug was released. When higher amounts were encapsulated, the protein continued to be released for 2 days, suggesting that the microneedle array developed in the study is amenable to dose adjustment as per the requirements of the therapeutic regimen. As compared with microneedles, passive diffusion of BSA using a propylene glycol solution did not result in any significant amount of drug permeation through the skin.
Conventional skin permeation where steady state can be established is based on the fact that the donor concentration is constant throughout the diffusion process. This can be proven by Fick’s first law for membrane diffusion.Citation5 However, in this study, the donor concentration decreased during the permeation process. Moreover, it is not a pure membrane diffusion process because micropassages were created by these microneedles, making the process a combination of diffusion through the skin membrane and mass transport through micron-sized channels across skin.
In vitro biocompatibility of polymeric microneedles
Human dermal fibroblasts and HaCaT keratinocytes are representative of skin cells and hence were used to assess the toxicity of the polymeric microdevice on the skin. These cells have been used previously to study the toxicity of transdermal polymeric dosage forms.Citation56,Citation57 HEK293 cells were used because they are representative of healthy human cells. HEK293 cells have been used in numerous in vitro toxicity studies as being representative of human cells since they offer a convenient model to evaluate toxicity at the cellular level.Citation58 They have also been previously reported with regard to transdermal toxicity from topical gels.Citation59 Using three different cells, we aimed to assess the local and systemic biocompatibility of our novel polymeric microneedles.
The MTT assay was used to assess the toxicity of microneedle extracts to these cells. Viable cells, possessing the active mitochondrial succinate-tetrazolium reductase system reduce MTT to formazan crystals which were quantified by colorimetric determination.Citation59 The cell viabilities were calculated as Apolymer/Acontrol × 100, where Apolymer and Acontrol were the absorbance measurements of the wells containing polymeric extracts and control (phosphate-buffered saline), respectively. Each value was an average of six replicates.
High cell viabilities with respect to the control were found for human dermal fibroblasts and HaCaT cells for exposure of cells to polymeric extracts up to 72 hours (), with cell survival numbers statistically insignificant between 24 and 72 hours (P > 0.05). This shows that the polymer used for fabricating the microneedles was safe to skin locally. HEK293 cell viability assays yielded similar viability for the first 24 hours, which subsequently decreased (P < 0.05, ). However, it has been reported that PEGDA with a molecular weight less than 20,000 Da can be cleared rapidly via the kidney by dissolution in body fluids.Citation60 Hence we expect minimal systemic toxicity due to PEGDA owing to its rapid clearance.
Figure 7 In vitro biocompatibility testing using MTT assay in (A) human dermal fibroblasts (HDF), (B) human adult low calcium high temperature (HaCaT) cells, and (C) human embryonic kidney 293 (HEK293) cells demonstrated high cell viability, indicating the biocompatibility of polymerized PEGDA microneedles.
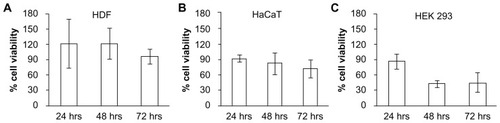
Fluorometric determination of lactate dehydrogenase leaked out from damaged cell membranes into the supernatant medium has been accepted as a method to determine the cytotoxicity of compounds and has been previously used for polymers as well.Citation61 In our study, low cell toxicities were observed in all the three cell lines for cells treated with polymer extracts. The percentage cytotoxicity for cells treated from 24–72 hours did not vary significantly (P > 0.05), supporting the results from the MTT assay and further proving the biocompatibility of polyethylene glycol diacrylate (). Overall, the in vitro toxicity results showed promising biocompatibility of the polymeric microneedles, both transdermally and systemically.
Figure 8 In vitro cytotoxicity testing using lactate dehydrogenase assay in (A) human dermal fibroblasts (HDF), (B) human adult low calcium high temperature (HaCaT) cells, and (C) human embryonic kidney 293 (HEK293) cells demonstrated low toxicity, indicating the biocompatibility of PEGDA microneedles.
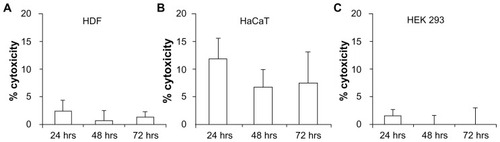
Conclusion
We have demonstrated the amenability of our photilithographic technique to encapsulate protein drugs. Drug distribution was found to be uniform across the microneedle array. Moreover, the process was proven to maintain protein stability and is hence expected to retain the biological activity of the encapsulated proteins. The encapsulated protein was released and permeated through the skin in much larger amounts as compared with passive diffusion. In vitro biocompatibility of the polymeric microneedles has been demonstrated by the low cytotoxicity of the polymeric extracts on different cell lines, indicating the safety of these microneedles. In conclusion, the prepared microneedles are expected to serve as a potentially useful drug delivery system to deliver biological drugs.
Acknowledgments
The authors would like to acknowledge Joleen Lim for her assistance with imaging the microneedle samples. We also thank Sathya Unudurthi, Sandeep Shelar, and Jun Yan for their help with the experiments in this study, and Brian W Dymock for his careful proofreading. We would also like to thank Joyce Lee and Aparna Saigal for their assisstance in preparation of the video abstract. Jaspreet Singh is the recipient of a National University of Singapore research scholarship. This study was supported by a startup grant from the National University of Singapore.
Disclosure
The authors report no conflicts of interest in this work.
References
- Food and Drug AdministrationApproved Drug Products with Therapeutic Equivalence Evaluations31st edRockville, MDUS Department of Health and Human Services2011
- DaughertyALMrsnyRJEmerging technologies that overcome biological barriers for therapeutic protein deliveryExp Opin Biol Ther20033710711081
- AhadAAqilMKohliKChemical penetration enhancers: a patent reviewExpert Opin Ther Pat200919796998819552513
- PrausnitzMRLangerRTransdermal drug deliveryNat Biotechnol200826111261126818997767
- KangLYapCWLimPFFormulation development of transdermal dosage forms: quantitative structure-activity relationship model for predicting activities of terpenes that enhance drug penetration through human skinJ Control Release2007120321121917582639
- GillHSPrausnitzMRCoated microneedles for transdermal deliveryJ Control Release2007117222723717169459
- ItoYMurakamiAMaedaTSugiokaNTakadaKEvaluation of self-dissolving needles containing low molecular weight heparin (LMWH) in ratsInt J Pharm20083491–212412917826015
- DonnellyRFMajithiyaRSinghTRDesign, optimization and characterisation of polymeric microneedle arrays prepared by a novel laser-based micromoulding techniquePharm Res2011281415720490627
- KaushikSHordAHDensonDDLack of pain associated with microfabricated microneedlesAnesth Analg200192250250411159258
- JiangJGillHSGhateDCoated microneedles for drug delivery to the eyeInvest Ophthalmol Vis Sci20074894038404317724185
- KoutsonanosDGdel Pilar MartinMZarnitsynVGTransdermal influenza immunization with vaccine-coated microneedle arraysPLoS One200943e477319274084
- KumarALiXSandovalMAPermeation of antigen protein-conjugated nanoparticles and live bacteria through microneedle-treated mouse skinInt J Nanomedicine201161253126421753877
- BaekCHanMMinJPrausnitzMRParkJHLocal transdermal delivery of phenylephrine to the anal sphincter muscle using microneedlesJ Control Release2011154213814721586307
- SullivanSPKoutsonanosDGDel Pilar MartinMDissolving polymer microneedle patches for influenza vaccinationNat Med201016891592020639891
- YouXQChangJHJuBKPakJJRapidly dissolving fibroin microneedles for transdermal drug deliveryMater Sci Eng C Mater Biol Appl201131816321636
- ItoYKashiwaraSFukushimaKTakadaKTwo-layered dissolving microneedles for percutaneous delivery of sumatriptan in ratsDrug Dev Ind Pharm201137121387139321545233
- MigalskaKMorrowDIGarlandMJLaser-engineered dissolving microneedle arrays for transdermal macromolecular drug deliveryPharm Res20112881919193021437789
- PanyamJDaliMMSahooSKPolymer degradation and in vitro release of a model protein from poly(D, L-lactide-co-glycolide) nano- and microparticlesJ Control Release2003921–217318714499195
- XieJWangCHEncapsulation of proteins in biodegradable polymeric microparticles using electrospray in the Taylor cone-jet modeBiotechnol Bioeng20079751278129017216662
- AngelovaAAngelovBMutafchievaRLesieurSCouvreurPSelf-assembled multicompartment liquid crystalline lipid carriers for protein, peptide, and nucleic acid drug deliveryAcc Chem Res201144214715621189042
- PanJChanSYLeeWGKangLMicrofabricated particulate drug-delivery systemsBiotechnol J20116121477148722076813
- AngelovaAOllivonMCampitelliABourgauxCLipid cubic phases as stable nanochannel network structures for protein biochip development: X-ray diffraction studyLangmuir2003191769286935
- SullivanSPMurthyNPrausnitzMRMinimally invasive protein delivery with rapidly dissolving polymer microneedlesAdv Mater2008205933938
- ItoYHagiwaraESaekiASugiokaNTakadaKFeasibility of microneedles for percutaneous absorption of insulinEur J Pharm Sci2006291828816828268
- LeeJWParkJHPrausnitzMRDissolving microneedles for transdermal drug deliveryBiomaterials200829132113212418261792
- DonnellyRFMorrowDIJSinghTRRProcessing difficulties and instability of carbohydrate microneedle arraysDrug Dev Ind Pharm200935101242125419555249
- ParkJHAllenMGPrausnitzMRPolymer microneedles for controlled- release drug deliveryPharm Res20062351008101916715391
- YangSFengYZhangLA scalable fabrication process of polymer microneedlesInt J Nanomedicine201271415142222457598
- KochharJSGohWJChanSYKangLA simple method of microneedle array fabrication for transdermal drug deliveryDrug Dev Ind Pharm4232012 [Epub ahead of print.]
- LaemmliUKCleavage of structural proteins during the assembly of the head of bacteriophage T4Nature197022752596806855432063
- MurayamaKTomidaMHeat-induced secondary structure and conformation change of bovine serum albumin investigated by Fourier transform infrared spectroscopyBiochemistry20044336115261153215350138
- EsteyTKangJSchwendemanSPCarpenterJFBSA degradation under acidic conditions: a model for protein instability during release from PLGA delivery systemsJ Pharm Sci20069571626163916729268
- WhitmoreLWallaceBAProtein secondary structure analyses from circular dichroism spectroscopy: methods and reference databasesBiopolymers200889539240017896349
- WhitmoreLWallaceBADICHROWEB, an online server for protein secondary structure analyses from circular dichroism spectroscopic dataNucleic Acids Res200432Web Server issueW668W67315215473
- AndradeMAChaconPMereloJJMoranFEvaluation of secondary structure of proteins from UV circular dichroism spectra using an unsupervised learning neural networkProtein Eng1993643833908332596
- ParkJHAllenMGPrausnitzMRBiodegradable polymer microneedles: fabrication, mechanics and transdermal drug deliveryJ Control Release20051041516615866334
- XieYXuBGaoYControlled transdermal delivery of model drug compounds by MEMS microneedle arrayNanomedicine20051218419017292077
- MosmannTRapid colorimetric assay for cellular growth and survival: application to proliferation and cytotoxicity assaysJ Immunol Methods1983651–255636606682
- BerckmansPLeppensHVangenechtenCWittersHScreening of endocrine disrupting chemicals with MELN cells, an ER-transactivation assay combined with cytotoxicity assessmentToxicol In Vitro20072171262126717572059
- GarlandMJCaffarel-SalvadorEMigalskaKWoolfsonADDonnellyRFDissolving polymeric microneedle arrays for electrically assisted transdermal drug deliveryJ Control Release20121591525922265694
- Gonzalez-GonzalezESpeakerTJHickersonRPSilencing of reporter gene expression in skin using siRNAs and expression of plasmid DNA delivered by a soluble protrusion array device (PAD)Mol Ther20101891667167420571543
- TitusJAHauglandRSharrowSOSegalDMTexas red, a hydrophilic, red-emitting fluorophore for use with fluorescein in dual parameter flow microfluorometric and fluorescence microscopic studiesJ Immunol Methods19825021932046806389
- KerwinBARemmeleRLJrProtect from light: photodegradation and protein biologicsJ Pharm Sci20079661468147917230445
- WeberKOsbornMThe reliability of molecular weight determinations by dodecyl sulfate-polyacrylamide gel electrophoresisJ Biol Chem196924416440644125806584
- HeYYeungESRapid determination of protein molecular weight by the Ferguson method and multiplexed capillary electrophoresisJ Proteome Res20021327327712645904
- ÇetinMVuralIÇapanYHincalAAPreparation and characterization of BSA-loaded alginate microspheresFabad J Pharm Sci2007323103107
- HusbandFAGarroodMJMackieARBurnettGRWildePJAdsorbed protein secondary and tertiary structures by circular dichroism and infrared spectroscopy with refractive index matched emulsionsJ Agric Food Chem200149285986611262041
- PriceNCConformational issues in the characterization of proteinsBiotechnol Appl Biochem200031Pt 1294010669400
- HuXCuiSLiuJFluorescence studies of interaction between flavonol p-coumaroylglucoside tiliroside and bovine serum albuminSpectrochim Acta A Mol Biomol Spectrosc201077254855320615751
- ZhuangWLiLLinGDengZPengMIlaprazole metabolites, ilaprazole sulfone and ilaprazole sulfide decreased the affinity of ilaprazole to bovine serum albuminJ Lumin20121322350356
- LakowiczJRProtein fluorescenceLakowiczJRPrinciples of Fluorescence SpectroscopyNew York, NYSpringer2006
- TanLLiuLXieQZhangYYaoSFluorescence quenching of bovine serum albumin in reversed micelles by CdS nanoparticlesAnal Sci200420344144415068284
- ParkJHAllenMGPrausnitzMRPolymer microneedles for controlled- release drug deliveryPharm Res20062351008101916715391
- HarveyAJKaestnerSASutterDEMicroneedle-based intradermal delivery enables rapid lymphatic uptake and distribution of protein drugsPharm Res201128110711620354765
- AitkenALearmonthMPProtein determination by UV absorptionWalkerJMThe Protein Protocols HandbookNew Jersey, NJHumana Press Inc2002
- MangalathillamSRejinoldNSNairACurcumin loaded chitin nanogels for skin cancer treatment via the transdermal routeNanoscale20114123925022080352
- MaupasCMoulariBBeduneauALamprechtAPellequerYSurfactant dependent toxicity of lipid nanocapsules in HaCaT cellsInt J Pharm20114111–213614121463666
- GoncharukVNezheradzeKDatskevichEThe use of HEK 293 human cells for assessing water toxicity before and after purification of ions of copper and zincJ Water Chem Tech20103215055
- RozmanBZvonarAFalsonFGasperlinMTemperature-sensitive microemulsion gel: an effective topical delivery system for simultaneous delivery of vitamins C and EAAPS Pharm Sci Tech20091015461
- VargheseSElisseeffJHHydrogels for musculoskeletal tissue engineeringAdv Polym Sci200620395144
- HiplerUCSchonfelderUHiplerCElsnerPInfluence of cyclodextrins on the proliferation of HaCaT keratinocytes in vitroJ Biomed Mater Res A2007831707917380497