Abstract
Nanosized maghemite particles were synthesized, precoated (with dimercaptosuccinic acid) and surface-functionalized with anticarcinoembryonic antigen (anti-CEA) and successfully used to target cell lines expressing the CEA, characteristic of colorectal cancer (CRC) cells. The as-developed nanosized material device, consisting of surface decorated maghemite nanoparticles suspended as a biocompatible magnetic fluid (MF) sample, labeled MF-anti-CEA, was characterized and tested against two cell lines: a high-CEA expressing cell line (LS174T) and a low-CEA expressing cell line (HCT116). Whereas X-ray diffraction was used to assess the average core size of the as-synthesized maghemite particles (average 8.3 nm in diameter), dynamic light scattering and electrophoretic mobility measurements were used to obtain the average hydrodynamic diameter (550 nm) and the zeta-potential (−38 mV) of the as-prepared and maghemite-based nanosized device, respectively. Additionally, surface-enhanced Raman spectroscopy (SERS) was used to track the surface decoration of the nanosized maghemite particles from the very first precoating up to the attachment of the anti-CEA moiety. The Raman peak at 1655 cm−1, absent in the free anti-CEA spectrum, is the signature of the anti-CEA binding onto the precoated magnetic nanoparticles. Whereas MTT assay was used to confirm the low cell toxicity of the MF-anti-CEA device, ELISA and Prussian blue iron staining tests performed with both cell lines (LS174T and HCT116) confirm that the as-prepared MF-anti- CEA is highly specific for CEA-expressing cells. Finally, transmission electron microscopy analyses show that the association with anti-CEA seems to increase the number of LS174T cells with internalized maghemite nanoparticles, whereas no such increase seems to occur in the HCT116 cell line. In conclusion, the MF-anti-CEA sample is a biocompatible device that can specifically target CEA, suggesting its potential use as a theragnostic tool for CEA-expressing tumors, micrometastasis, and cancer-circulating cells.
Introduction
Colorectal cancer (CRC) is one of the most frequently occurring cancers affecting patients worldwide. In the United States alone in 2011, there was an estimated 147,000 new cases and 50,000 deaths related to cancer of the large intestine (colon, rectum, and anus).Citation1 Furthermore, it has been reported that 5-year disease-free survival decreases with more advanced stages of CRC. This means that tools and methodologies that allow early cancer detection directly affect survival times and significantly reduce CRC mortality.Citation2
Recently, nanostructure-based materials, such as surface-functionalized magnetic nanoparticles, have been tested as promising devices for early cancer detection. Additionally, magnetic nanoparticles have been used as enhanced contrast agents in magnetic resonance imaging (MRI) for noninvasive detection of cancer and lymph node metastasisCitation3,Citation4 with remarkable action in imaging-guided therapy.Citation5 Maximum advantage of iron-based nanoparticles for imaging has been taken in special methods for MRI such as ultrashort echo time imaging, which improves nanoparticle contrast and enables iron quantification in tissues.Citation6
Magnetic nanoparticles have also been investigated for cancer therapy application, as they are suitable for magnetic hyperthermia.Citation7,Citation8 Magnetic nanoparticles may also be designed for multiple potential therapeutic approaches as chemotherapeutics delivery carriers and photodynamic therapy.Citation9,Citation10
Both diagnostics and therapeutics can be simultaneously provided by magnetic nanoparticles, and they are therefore considered as a platform of promising theragnostic precursors, especially because magnetic targeting of the tumor may be achieved, leading to an accumulation up to eightfold higher.Citation5 Furthermore, iron-based nanoparticles can be chemically modified with a targeting particular moiety, such as antibodies, specifically to detect tumorsCitation11,Citation12 and circulating tumor cells,Citation13 improving micrometastasis diagnosis. Monitoring micrometastasis appearance by detection of biological markers may be helpful in the clinical management of patients and in a specific therapy design device. In CRC patients, detection of circulating tumor cells expressing carcinoembryonic antigen (CEA) has been related to stabilization of the disease and overall survival.Citation14
CEA is a 180–200 kDa glycoprotein member of the immunoglobulin supergene family, whose expression is limited in normal adult tissue whereas it is widely detected in many types of primary and metastatic tumors, especially CRC.Citation15,Citation16 Therefore, CEA has been chosen as the targeted antigen for detection of primary and metastatic CRCCitation17,Citation18 and also as a possible target for antibody-mediated therapy.Citation19,Citation20
The aim of the present study was to develop a biocompatible magnetic fluid containing stably suspended maghemite nanoparticles (MNPs) conjugated to anti-CEA as a device for potential theragnostic approaches to CRC. The surface-enhanced Raman spectroscopy (SERS) method was innovatively used to probe the nanosized maghemite/anti-CEA conjugation. Additionally, the right conjugation of anti-CEA to MNPs is critical for the end material device to function, and was indeed confirmed by biological tests.
Material and methods
Preparation and characterization of the magnetic fluid (MF) sample
Magnetite nanoparticles were synthesized by chemical coprecipitation of Fe(II) and Fe(III) ions in aqueous solution after controlled addition of the alkaline aqueous solution. Following the coprecipitation step, the as-produced nanosized magnetite sample was oxidized into MNPs using oxygen bubbling at 95°C for 5 hours. The MNPs were then washed with 1.5 mol/L HCl aqueous solution and separated from the supernatant using a permanent magnet. The sediment, containing the MNPs, was dispersed in NaCl solution and then coated with meso-2,3-dimercaptosuccinic acid (DMSA), according to the procedure previously reported for the production of stable magnetic fluid samples.Citation21 The total iron concentration in the MF sample as determined by atomic absorption was 17 mg/mL and the crystalline mean diameter estimated from the X-ray diffractogram (X-ray diffractometer, Shimadzu XRD 6000; Shimadzu Corporation, Kyoto, Japan) using Scherrer’s equation was 8.3 nm. From these data, the concentration of nanoparticles was estimated within the as-prepared MF sample (1.5 × 1019 nanoparticles/mL). The average values of the hydrodynamic diameter and zeta potential of the DMSA-coated MNPs dispersed within the MF sample were 190 nm and −47.5 mV, respectively (ZetaSizer Nano S; Malvern Instruments Ltd, Malvern, UK).
Cell culture
Two human CRC cell lines were utilized in this study. The LS174T cell line was provided by Dr Fernando Kreutz (FKBiotec, Rio Grande do Sul, Brazil) and the HCT116 cell line was provided by Dr Jose Morgado Díaz (INCA, Rio de Janeiro, Brazil). Both LS174T and HCT116 cells were cultured in flasks (TPP, Trasadingen, Switzerland) with RPMI-1640 medium (Sigma-Aldrich, St Louis, MO) containing 1% (v/v) penicillin–streptomycin (Sigma-Aldrich) and 10% (v/v) heat-inactivated fetal bovine serum (Gibco, Carlsbad, CA). Cells were maintained at 37°C in humidified atmosphere with 5% CO2.
Cell viability
In order to evaluate the MF toxicity, the MTT assay (Invitrogen, Carlsbad, CA) was performed according to Carneiro et al.Citation9 LS174T and HCT116 cells were seeded into 96-well culture microplates at a density of 2 × 104 cells/well for viability tests after 5 and 12 hours or 104 cells/well for viability tests after 24 and 48 hours. The day after, cells were incubated with 150 μL of culture medium and 50 μL of phosphate-buffered saline (PBS) containing MF at eight different iron concentrations of 0.0 (negative control), 0.1, 1, 5, 10, 30, 60, 80, and 100 ng/μL culture media for 5, 12, 24, or 48 hours. For positive control, cells were incubated with hydrogen peroxide at 0.1 and 0.5 mM for the same period. After treatment, cells were incubated with MTT (5 mg/mL). Cell viability was assessed by measuring the absorbance at 595 nm, using a microplate reader (SpectraMax M2; Molecular Devices, Sunnyvale, CA).
Anti-CEA conjugation to MNPs
For conjugation of anti-CEA antibody onto MNPs EDC (1-ethyl-3-[3-dimethylaminopropyl] carbodiimide hydrochloride; Sigma-Aldrich) was used to activate terminal carboxyl groups in the DMSA-functionalized MNPs for the conjugation with primary amines of anti-human CEA antibodies. Addition of NHS (N-hydroxysuccinimide; Sigma- Aldrich) was used as a strategy to increase the efficiency of EDC-mediated coupling reactions by stabilization of the amine-reactive intermediate. The EDC/NHS protocol was employed as described by Acharya et al,Citation22 with slight modifications. Briefly, 1.5 mL of EDC (1 mg/mL in PBS) and 1.5 mL of NHS (1 mg/mL in PBS) were added to 30 mL PBS containing 1.5 mL MF sample (approximately 60 mg MNPs) and left at room temperature under shaking for 4 hours. Unreacted EDC and NHS were removed by three rounds of centrifugation at 20,000 rpm for 40 minutes, at room temperature (model CR22G/CR21G; Hitachi Ltd, Tokyo, Japan). The resultant pellet was dispersed in 2 mL PBS and a 500-μL aliquot was saved for tests and labeled MF-NHS. To the remaining MF sample (MF-NHS), anti-CEA antibody (rabbit polyclonal – DBS) was added at 200 μg/mL final concentration. The suspension was again left under shaking for 2 hours at room temperature and then incubated overnight at 4°C. The unbound antibody was removed by three rounds of centrifugation at 20,000 rpm for 40 minutes, at 4°C, while the supernatants were saved for tests. The MNP conjugated to anti-CEA was dispersed in 1 mL PBS and the resulting MF sample was labeled MF-anti-CEA.
Iron concentration of MF-anti-CEA
The MF-anti-CEA iron concentration was determined by the colorimetric ferrozine method. Briefly, 100 μL HCl 1.2 N was added to 100 μL diluted MF-anti-CEA (1:1000) and the suspension was incubated at 80°C for 1 hour. After cooling the suspension down to room temperature, 500 μL of 15% ammonium acetate was added, followed by the addition of 100 μL 4% ascorbic acid, 2.5% sodium dodecyl sulfate, and 1:10 diluted ferrozine (Labtest Diagnóstica SA, Lagoa Santa, Brazil). The sample was incubated at 37°C for 10 minutes and the absorbance was read at 560 nm. The MF-anti-CEA iron concentration was calculated by comparing this absorbance to the MF sample calibration curve, in which the iron concentration ran from 0.038 to 39.2 μg/mL. The calibration curve was equally assessed by the ferrozine method.
Characterization of MF-anti-CEA
Diluted MF-anti-CEA (1:800) was dropped onto a formvar-coated grid and examined using a JEOL 1011 transmission electron microscope (JEOL, Tokyo, Japan). The hydrodynamic diameter of anti-CEA-MNPs and the zeta potential of the MF samples were determined by laser light scattering using a particle size analyzer (ZetaSizer; Malvern Instruments). The MF samples containing MNPs or anti-CEA-MNPs were analyzed in a 1 cm quartz cell. Measurement was performed in triplicate. All experiments were carried out at 25°C in the range of 100–2000 Hz.
SERS
Anti-CEA conjugation onto the surface of the MNPs was assessed by SERS. Silver films used as substrate for SERS measurements were prepared by the electrolytic method, using a solution of AgNO3 (0.1 mg/mL) as electrolyte. For SERS studies a solution of 10 μL MF, MF-NHS, and MF-anti-CEA samples were diluted in 10 μL distilled water and dripped onto the silver film, then dried with dry nitrogen. SERS spectra were immediately recorded.
The samples were excited by the 514.5 nm line of an Argon ion laser. A cylindrical lens was used to focus the laser beam onto the probed surface containing the samples, at a power of 0.1 W/cm2. The scattered light was collected in almost backscattering configuration and analyzed using a triple spectrometer (Jobin Yvon Model T64000; Horiba, Tokyo, Japan) equipped with a charge-coupled device detector.
In vitro cell binding and specificity
In order to evaluate if the suspended nanoparticles within the MF-anti-CEA sample were capable of specifically binding to their target (CEA), LS174T (high level of CEA expression)Citation23 and HCT116 (low level of CEA expression)Citation24 cells were assessed by enzyme-linked immunosorbent assay (ELISA). Cells were seeded into 96-well culture microplates (2 × 104 cells/well) and incubated for 24 hours to allow cell adhesion. Then cells were fixed for 10 minutes with 4% paraformaldehyde in PBS, washed three times with PBS, and then blocked overnight with 5% dried milk powder in PBS. The day after, MF-anti-CEA was used as the primary antibody for 2 hours. Unbounded anti-CEA-MNPs were washed away with PBST (PBS containing 0.05% Tween-20) and cells were incubated with alkaline phosphatase-conjugated mouse-anti-rabbit IgG secondary antibody (Sigma-Aldrich) for 1 hour. After two washes with PBST and one wash with APB (100 mM tris HCl pH 9.5, 100 mM NaCl, 50 m M MgCl2), 200 μL of pNPP (para-nitro-phenyl-phosphate) – 1 mg/mL in APB – were added. After 30 minutes, absorbance was measured at a 405 nm wavelength. As experimental control, the MF sample and supernatants saved from antibody conjugation were also analyzed.
Perls Prussian blue iron staining
In order to confirm anti-CEA-MNP specificity, 105 cells were seeded on glass coverslips placed in a 24-well culture microplate and incubated for 24 hours. Either MF or MF-anti- CEA samples were added to cell culture at 30 ng/μL final iron concentration in culture medium. After 5 hours, the cells were visualized by Prussian blue staining for iron detection. Cells were fixed for 5 minutes in ice-cold methanol, stained for 15 minutes with an equal volume of 2% hydrochloric acid and 2% potassium ferrocyanide trihydrate, and counterstained for 3 minutes with 0.5% nuclear fast red. Cells were washed with distilled water and air-dried, and coverslips were mounted in Kisser’s glycerol jelly (equal volume of glycerol and 2.5% gelatin in distilled water).
Transmission electron microscopy (TEM)
Routine TEM analyses were carried out to evaluate MNP uptake by LS174T and HCT116 cells incubated for 5 hours with both MF and MF-anti-CEA samples at a final iron concentration of 60 ng/μL. Briefly, cultured cells were centrifuged (1000 g, 2 minutes) after trypsin digestion in culture plates. The cell masses were fixed in modified Karnovsky’s fixative (2% paraformaldehyde, 2.5% glutaraldehyde in 0.1 M sodium cacodylate buffer, pH 7.2) for 2 hours at room temperature. Samples were post-fixed in a solution containing 1% osmium tetroxide, 0.8% potassium ferricyanide, and 5 mM calcium chloride and contrasted in bloc with uranyl acetate. Samples were then dehydrated in acetone and embedded in Spurr. Semi-thin sections (3 μm) were stained with toluidine blue and examined under a light microscope to localize cells with visible nucleus. Ultra-thin sections (70 nm) were examined using a JEOL 1011 transmission electron microscope (JEOL). For all treatments, at least 50 cells in the same grid area were counted and quantified for the presence of nanoparticles inside or outside cells.
Statistical analysis
GraphPad Prism 5.0 (Sigma Stat; Prism 5.0; GraphPad Software Inc, San Diego, CA) was utilized to perform the two-way analysis of variance (ANOVA) test. When statistically significant differences were found (significance level adopted was 5%), analysis was complemented by the Bonferroni method. Data were presented as means ± standard error.
Results
MF toxicity
As a mandatory step in the development of the potential cancer theragnostics MF-anti-CEA device, its precursor, the MF sample (DMSA-coated MNPs) was investigated in order to assess its biocompatibility. The MF sample’s cytotoxicity was assessed by the MTT assay (), using the two colon cancer cell lines (HCT116 and LS174T cells).
Figure 1 Evaluation of MF biocompatibility in human colorectal cancer cells. MTT assay in HCT116 (A) and LS174T (B) cells cultivated with MF at different iron concentrations.
Notes: Data were normalized with the negative control (cells without treatment) and expressed as mean ± standard error of experiments performed in triplicate. Different symbols indicate statistical difference (P < 0.05) compared to the negative control in time 5 hours (*), 12 hours (#), 24 hours (⊗), and 48 hours (●). Cells cultivated with H2O2 (hydrogen peroxide at 0.1 and 0.5 mM) are positive control groups.
Abbreviation: MF, magnetic fluid.
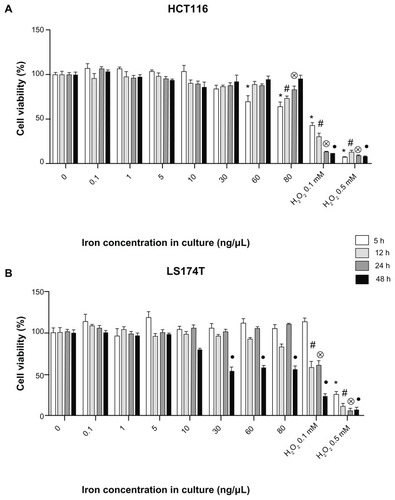
The results show that the MF sample induced a decrease in HCT116 cell viability () when cultivated for 5 hours at iron concentrations of 60 and 80 ng/μL. A decrease in cellular viability was also observed after 12 and 24 hours, but only at the highest iron concentration (80 ng/μL). However, after 48 hours, cell viability in all groups was statistically equal to the negative control ().
An MTT assay was also performed for LS174T cells (). No decrease in cell viability was observed in LS174T cells cultivated with the MF sample until 24 hours. Nevertheless, a decrease in LS174T cell viability was observed after 48 hours culture with the MF sample at iron concentration ranging from 30 to 80 ng/μL.
Synthesis and characterization of MF anti-CEA
In order to develop a magnetic material device for therapeutic and diagnostic applications in CRC, anti-CEA antibody was bound to surface-functionalized MNPs dispersed in the MF sample using the EDC/NHS method. The resulting MF sample containing anti-CEA-MNPs was labeled MF-anti-CEA. The iron concentration of the as-prepared MF-anti-CEA was determined as 9.2 mg/mL using the ferrozine colorimetric method, while comparing its absorbance against a standard curve obtained for the MF sample (R2 = 0.9975).
TEM micrographs showed that MNPs in the MF sample () as well as the MNPs in the MF-anti-CEA sample () present a spherical shape, evidencing that the antibody conjugation did not alter the MNPs’ morphology. Different sized nanoparticles were also observed in the samples analyzed by TEM. Nevertheless, the polydispersity index of MNPs in the MF samples, as determined by dynamic light scattering, was 0.15. The average hydrodynamic diameter of the MNPs suspended within the MF sample was 190 nm (). However, after antibody conjugation, the average hydrodynamic diameter of the suspended MNPs increased to approximately 550 nm (). Small peaks around 100 nm and 5 μm are also showed in .
Figure 2 Characterization of nanoparticles by transmission electron microscopy and dynamic light scattering. Electron micrograph of maghemite nanoparticles in MF sample (A) and anti-CEA conjugated nanoparticles in MF-anti-CEA sample (B). Hydrodynamic size of nanoparticles in MF sample (C) and MF-anti-CEA sample (D).
Note: Red, green, and blue lines represent the first, the second, and the third measurement of the triplicate, respectively.
Abbreviations: MF, magnetic fluid; CEA, carcinoembryonic antigen; (d · nm), diameter in nanometers.
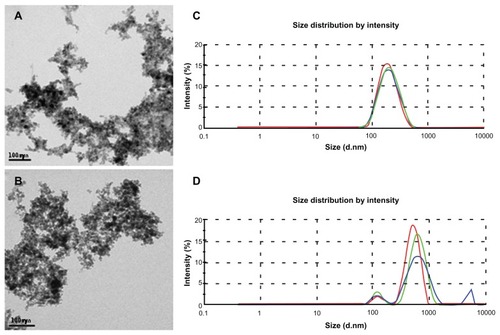
Zeta potential shifts from −47.5 mV (MF sample) to −38 mV (MF-anti-CEA sample), revealing that MNPs in both MF samples are negatively charged.
SERS
In this study SERS was used as the spectroscopy tool to evaluate the efficacy of anti-CEA antibody binding onto MNPs. show the recorded SERS spectra of the as-prepared MF, the intermediate MF-NHS, and the MF-anti-CEA samples, respectively. Notice that in the SERS spectrum of the anti-CEA shell is also included for comparison, revealing characteristic Raman bands while not binding onto the MNPs.
Figure 3 SERS. Raman spectra recorded for (A) MF, (B) MF-NHS, and (C) MF-anti- CEA, and (D) free anti-CEA samples.
Abbreviations: SERS, surface enhanced Raman spectroscopy; MF, magnetic fluid; CEA, carcinoembryonic antigen.
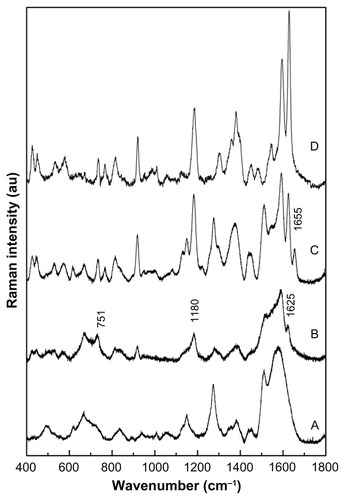
In , in the 1300–1650 cm−1 region there are bands related to the carboxylate, whereas in the 800–1300 cm−1 region Raman modes of (C–S) and (C–C) bonds of the skeletal chain of DMSA are observed. Additionally, the SERS spectrum of the MF sample reveals a new band around 500 cm−1 (S–S).
The MF-NHS SERS spectrum included in presents the same features as the MF sample SERS spectrum included in . However, a more detailed analysis of the SERS spectrum shown in reveals that the shoulder at 1625 cm−1 and weak features peaking at 1180cm−1 and 751 cm−1 are respectively associated with the vibrations of C=O, S=O, and N–O bonds of NHS. Raman shifts observed for vibrational modes of the MF-NHS carboxylate groups compared to the MF sample were slight.
In the MF-anti-CEA SERS spectrum a Raman peak at 1655 cm−1, related to the C=O stretching of a secondary amide, is observed, as shown in . Note that the 1655 cm−1 feature peak is absent in the free anti-CEA spectrum ().
It is important to note that all peaks observed in the free anti-CEA SERS spectrum were also observed in the MF-anti-CEA SERS spectrum (modes assigned with the amide groups of the protein structure, amino acid and terminal amines and carboxylic). Bands associated with the DMSA molecule are also identified in the SERS spectrum of the MF-anti-CEA sample included in . In addition, the vibrational Raman energies associated with the anti-CEA conjugated onto MNPs show only slight changes when compared to the vibrational modes of free anti-CEA.
In vitro cell binding and specificity
shows CEA expression levels of the two human colorectal carcinoma cell lines in this study. LS174T cells have a high level of CEA expression, whereas no CEA was detected by ELISA in HCT116 cells. In order to evaluate cell-binding efficacy of the suspended nanoparticles within the MF-anti-CEA sample, ELISA was performed with LS174T cells and MF-anti-CEA as the primary antibody (). Data show cell binding by MF-anti-CEA and also by supernatants 1 and 2, but not supernatant 3.
Figure 4 In vitro cell binding and specificity of MF-anti-CEA by ELISA. (A) Detection of CEA expressed in human colorectal cancer cells. LS174T and HCT116 cells were assessed by ELISA with anti-CEA at two different concentrations as primary antibody; (B) LS174T cells’ binding efficacy of MF-anti-CEA. ELISA was performed with either MF-anti-CEA or supernatants from antibody conjugation process as primary antibody; (C) MF-anti-CEA specificity for CEA was assessed in LS174T and HCT116 cells by ELISA with MF-anti-CEA as primary antibody. In figures (B and C) PBS and MF were utilized as negative controls and free anti-CEA antibody as positive control.
Note: Different letters mean statistical difference (P < 0.05).
Abbreviations: MF, magnetic fluid; CEA, carcinoembryonic antigen; ELISA, enzyme-linked immunosorbent assay; PBS, phosphate buffered saline.
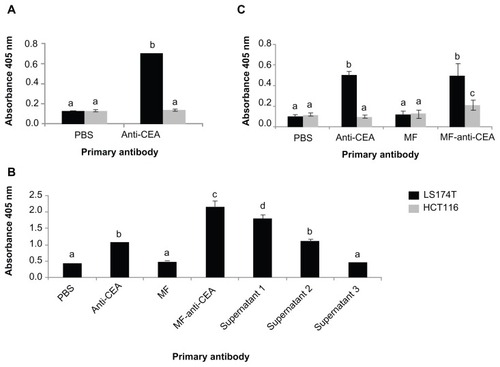
ELISA was also performed with both LS174T and HCT116 cells () to verify MF-anti-CEA specificity for CEA. The signal of LS174T cells assessed by MF-anti- CEA was statistically equal (P = 0.63) to the signal of anti- CEA free antibody and statistically higher (P = 0.00) than the signal observed for HCT116 cells.
Perls Prussian blue iron staining
Perls Prussian blue staining was another test performed to confirm MF-anti-CEA specificity (). Although the same iron concentration was employed in all cell cultures, there was an increase in the amount of blue-stained iron in cells cultivated with the MF-anti-CEA sample when compared to those cultivated with the MF sample (). We also observed that the amount of blue-stained iron was higher for LS174T than for HCT116 cells ().
Figure 5 In vitro specificity of MF-anti-CEA by Prussian blue iron staining. LS174T (A–C) and HCT116 (D–F) cells were cultivated for 5 hours with MF (B and E), and MF-anti-CEA (C and F) at same iron concentration (60 ng/μL). Untreated cells (A and D) were negative control.
Abbreviations: MF, magnetic fluid; CEA, carcinoembryonic antigen.
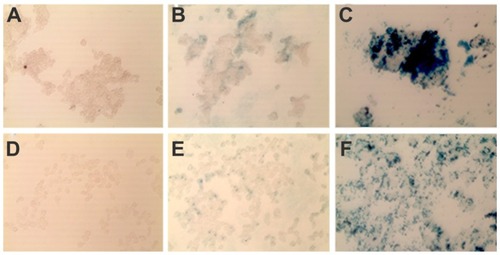
Transmission electron microscopy
TEM was performed to evaluate if the anti-CEA bonded in MNPs would improve MNP uptake by CEA-expressing cells. Both LS174T and HCT116 cells were cultured with either MF or MF-anti-CEA and analyzed about MNPs’ localization inside or outside the cells.
MNPs of both MF and MF-anti-CEA samples were observed in the cytoplasm of both HCT116 and LS174T cells (). However, an apparently increased number of LS174T cells with internalized MF-anti-CEA MNPs were observed while in comparison to the number of LS174T cells which internalized MF MNPs. Additionally, the presence of anti-CEA seems not to cause this increase in HCT116 cells.
Figure 6 MNP uptake into human colorectal cancer cells. Electron micrographs of LS174T cells incubated with MF MNP (A) or MF-anti-CEA MNP (B) and HCT116 cell incubated with MF-anti-CEA MNP (C).
Notes: Arrows point to MNP aggregates. Bars = 2 um (A) 1 μm (B) 0.5 μm (C).
Abbreviations: MNP, maghemite nanoparticle; MF, magnetic fluid; CEA, carcinoembryonic antigen.

Discussion
It has been reported that the early detection of cancer improves disease prognosis and cure and therefore sensitive methodologies with minimal invasion that are able to detect cancer cells are being developed.Citation25–Citation27 In this context, MNPs are very promising. Due to their superparamagnetic core, MNPs can be efficiently detected by MRI with high sensitivity and tissue resolution.Citation28 Furthermore, MNPs are more biocompatible and present longer circulation time than the toxic gadolinium complex-based contrast agent used clinically.Citation29 Besides these interesting characteristics, MNPs have been also studied for cancer therapy application. Due to their iron core, they are suitable for magnetic hyperthermia. When submitted to an A/C magnetic field, MNPs release thermal energy, providing heating and leading to cell death and subsequently to a decrease in tumor size.Citation7,Citation8 Besides application in the magnetohyperthermia process, MNPs may also be designed for multiple potential therapeutic approaches such as chemotherapeutic delivery carriers, and photodynamic therapy.Citation9,Citation10,Citation30
In the present study we developed a biocompatible nanosized material, labeled MF-anti-CEA, with potential for both diagnosis and treatment of CEA-expressing cancer cells, such as CRC cells. MF-anti-CEA biocompatibility was confirmed by MTT assay using HCT116 and LS174T cells cultivated with MF, the MF-anti-CEA precursor. Both cell lines were chosen to evaluate the specificity of the as-prepared MF-anti-CEA device.
MTT data show that, in spite of an initial decrease in HCT116 cell viability, cells were able to recover to normal levels whereas the time for cell retrieval was found to be dependent upon iron concentration. For cultures with 60 ng/μL MF sample, the cell viability was already comparable to the negative control in the time window of 12 hours, whereas at 80 ng/μL 48 hours were needed to achieve the same result. It is reasonable to assume that cells were able to recover because they were not irreversibly damaged. Indeed, for human lung epithelial cells cultivated for 25 hours with maghemite and carbon black nanoparticles, Berg et alCitation31 observed a decrease in cell viability assessed by MTT while no cell death was detected by other tests.
In LS174T cells, no toxicity was observed for 24 hours. Similar results were obtained by Chen et alCitation32 for HeLa cells cultivated for 24 hours with graphene oxide-loaded magnetite nanoparticles, which were considered as a biocompatible sample because they caused no decrease in HeLa cells’ viability, even at iron concentrations up to 80 ng/μL, the same maximum concentration we used in our study.
Ankamwar et alCitation33 suggested that magnetite nanoparticles presenting no reduction in cell viability (for different cell lines) at a concentration of 10 ng/μL may be considered biocompatible, even though at 100 ng/μL they may induce a decrease in cell viability of some cell lines. In this way, despite the decrease in LS174T cell viability after 48 hours culture with the MF sample at iron concentrations ranging from 30 to 80 ng/μL, the sample may still be considered biocompatible.
Furthermore, comparing HCT116 and LS174T cells’ viability after culture with H2O2 (positive control), we observed that the former is more susceptible to oxygen peroxide effects. After being cultivated for 5 hours with 0.1 mM H2O2, HCT116 cells presented a significant decrease in cell viability, whereas for LS174T cells this decrease was observed only after 12 hours. As H2O2 iron-based nanoparticles can generate reactive oxygen species (ROS),Citation34 it is reasonable to assume that the transient decrease in HCT116 cell viability induced by the MF sample was more likely caused by ROS. If the effects of H2O2 on the LS174T cell line are delayed when compared to the HCT116 cell line, we can hypothesize that LS174T cells would be able to recover to normal cell viability levels if MTT assays are performed after longer periods of time.
Viability results obtained by in vitro MTT tests show that the MF sample containing DMSA-coated MNPs used in this study is biocompatible. Likewise, data in the literature show that magnetic fluid samples based on DMSA-coated magnetite nanoparticles investigated in animals are also biocompatible.Citation35 Therefore, we considered that the employed MF sample could be used as an adequate MF-anti-CEA precursor.
After anti-CEA conjugation onto MNPs, MF-anti-CEA was characterized by TEM and dynamic light scattering. The conjugation process did not alter MNPs’ morphology but did increase the hydrodynamic size. The increase in hydrodynamic size is frequently observed after antibody conjugation onto nanosized particles.Citation36,Citation37 However, the hydrodynamic diameter increase is usually smaller (ranging from 15 to 60 nm) than that observed in this study. This finding is probably related not only to anti-CEA conjugation but also to MNP aggregation. Actually, the literature reports that both large particles and particle aggregates increase light scattering.Citation38 The small peak around 100 nm observed in MF-anti-CEA suggests that some MNPs were not bound to anti-CEA or did not aggregate after conjugation, whereas the small feature peaking at about 5 μm indicates MNPs sedimentation in the MF-anti-CEA sample, especially because it was apparent only in the third measurement. Dynamic light scattering also provided the zeta potential of MF-anti-CEA and its precursor MF. The negative values obtained for MF and MF-anti-CEA samples, ranging from −47.5 mV to −38 mV, are in accordance with those reported in the literature for antibody-conjugated nanoparticles and suggest stability.Citation39
In order to assess the efficiency of anti-CEA binding onto nanoparticles, SERS was successfully used. This tool allowed tracking the surface decoration of the nanosized maghemite particles from the very first precoating up to the attachment of the anti-CEA moiety. In the MF sample spectrum, the bands related to carboxylate and the Raman modes of (C–S) and (C–C) bonds of the skeletal chain of DMSA indicate that DMSA is covalently bonded through the carboxylate terminal onto the MNPs surface. Furthermore, in the MF sample spectrum the Raman mode of v(S–S) was also observed, which is characteristic of disulfide cross-linkages (S–S) among molecular units of neighboring DMSA attached onto the MNPs surface. Actually, the S–S cross-linkages are alleged to play a key role in the stabilization of the built in surface molecular layer.Citation40 Data obtained by Raman also allow the observation of a NHS-linker effectively attached at the surface of the MNPs suspended within the MF sample, because the shoulder at 1625 cm−1 and weak features peaking at 1180 and 751 cm−1 are respectively associated with the vibrations of C=O, S=O, and N–O bonds of NHS. Small Raman shifts observed for vibrational modes of the MF-NHS carboxylate groups compared to the MF sample suggest that NHS is not strongly bonded to the DMSA shell, as expected. In fact, NHS is an intermediate linker for a subsequent step, namely the reaction between DMSA carboxyl and anti-CEA amine groups. This reaction is evidenced by the onset of a Raman peak at 1655 cm−1 present in the MF-anti-CEA SERS spectrum, but absent in the free anti-CEA spectrum. According to ParkerCitation41 the Raman mode peaking at ~1655 cm−1 is assigned to the C=O stretching of a secondary amide. Thus, the presence of this peak is claimed to be due to the vibration of a carbonyl group formed after conjugation of the anti- CEA to the DMSA linked onto the MNPs. Interestingly, modes assigned to the amide groups of the protein structure, amino acid and terminal amines and carboxylic observed in all peaks of free anti-CEA spectrum, are also present in the MF-anti-CEA spectrum, confirming anti-CEA conjugation. Furthermore, the slight changes in the vibrational Raman energies associated with the anti-CEA conjugated onto MNPs when compared to the vibrational modes of free anti-CEA demonstrate that the process of conjugation does not lead to a relevant denaturation, consequently suggesting that its biological functions are fully preserved. Indeed, as revealed in the discussion presented above, SERS was used as a key experimental tool to investigate the binding process of molecules onto MNPs as well as the signatures of the molecular surface decoration in the final product, in our case the MF-anti-CEA sample. According to Occhipinti et al,Citation42 conservation of the antibody biological functionality after conjugation is poorly evidenced due to the lack of reliable methods for assessing information in regard to the structural/conformational features that indicate antibody functionality. In the present study, however, we found SERS was successfully employed to evaluate the anti-CEA binding onto MNPs. Besides being suitable for demonstrating the antibody conjugation and how the antibody is chemically bonded, SERS also provided information that suggests anti- CEA functionality was preserved.
Anti-CEA functionality after the conjugation process was confirmed by ELISA and Prussian blue iron staining performed with both LS174T and HCT116 cells. ELISA was performed to compare CEA expression between cell lines, and the results are in accordance with the literature. LS174T are high-level CEA-expressing cells,Citation23 producing around 2 μg/106 cells/10 days,Citation43 whereas HCT116 cells express low levels of CEA estimated to be about 1–2 ng/106 cells/3 days,Citation24 which was not detected by ELISA. Due to their different CEA expression levels these two cell lines were chosen to assess cell binding and specificity of the as-prepared MF-anti- CEA sample.
MF-anti-CEA was able to target LS174T cells, confirming Raman evidence of anti-CEA functionality. Since MF-anti-CEA targeting of LS174T cells was statistically higher than of HCT116 cells, as observed by ELISA and confirmed by Perls Prussian iron staining, we can state that the as-developed MF-anti-CEA is highly specific for CEA-expressing cells. It is also important to emphasize that there was no binding when supernatant 3 was used as the primary antibody. Since supernatant 3 represents the last washing of MNPs in the anti-CEA conjugation process, this important result shows that unconjugated antibodies were successfully removed from MF-anti-CEA.
After verifying that the suspended nanoparticles within the MF-anti-CEA sample are able to specifically bind to their target, TEM was performed to evaluate the cellular uptake of MNPs. Indeed the binding of MF-anti-CEA to CEA expressed in cell membranes improved MNP uptake by target cells. Apart from providing specific binding of MNPs to target cells, which is very suitable for specific diagnosis and magnetohyperthermia applications, the anti-CEA conjugated to MNPs facilitated MNP uptake by target cells. These data strongly suggest that MF-anti-CEA is a very promising material device for carrying drugs, since the efficiency of a drug-delivery system based on MNPs may depend on the specific accumulation of MNPs inside cancer cells.Citation22
Taken together, the results obtained in this study indicate that the as-developed MF-anti-CEA device has enormous therapeutic potential as MF-anti-CEA and can be further engineered to act as a drug-delivery system or alternatively to be used in magnetohyperthermia, while responding to an A/C magnetic field. Moreover, MF-anti-CEA can also be tailored as a contrast agent in magnetic resonance for both diagnosis and imaging guided therapy.
Conclusion
In conclusion, this study reports on the preparation, characterization, and biological test of a potential theragnostics material device (MF-anti-CEA) engineered to specifically target CEA-expressing cancer cells. Anti-CEA antibody was successfully conjugated to DMSA-coated nanosized maghemite particles. The as-prepared MF-anti-CEA device was analyzed by TEM, dynamic light scattering, and electrophoretic mobility measurements, revealing desirable characteristics. SERS indicated that anti-CEA is successfully bonded onto DMSA-coated nanosized maghemite particles, leading to a specific targeting capability to CEA expressing CRC cells, as showed by ELISA and Prussian blue iron staining. Furthermore, the presence of the antibody anti-CEA seems to facilitate the preferential uptake of the MF-anti-CEA device by CEA expressing LS174T cells. Data suggests MF-anti-CEA is a potential theragnostics tool for CEA-expressing tumors, micrometastasis, and cancer circulating cells.
Acknowledgments
/disclosures
The authors report no conflicts of interest in this work. We thank the Brazilian agencies CNPq, REDE CON-NANO/CAPES-, INCT-Nanobiotecnologia (MCT/CNPq), CNANO/IB/UnB, DPP/UnB, and FAP-DF for financial support. We are grateful to Dr Izabel Cristina da Silva for assistance with statistical analysis. We thank Dr Alexsandro Galdino and Msc José Luiz Jivago de Paula for assistance with artwork.
References
- SiegelRWardEBrawleyOJemalACancer statistics, 2011: the impact of eliminating socioeconomic and racial disparities on premature cancer deathsCA Cancer J Clin201161421223621685461
- BüngerSHaugUKellyFMToward standardized high-throughput serum diagnostics: multiplex-protein array identifies IL-8 and VEGF as serum markers for colon cancerJ Biomol Screen20111691018102621807963
- LiMKimHSTianLYuMKJonSMoonWKComparison of two ultrasmall superparamagnetic iron oxides on cytotoxicity and MR imaging of tumorsTheranostics201221768522272221
- LimSWKimHWJunHYTCL-SPION-enhanced MRI for the detection of lymph node metastasis in murine experimental modelAcad Radiol201118450451121216633
- ChengLYangKLiYMultifunctional nanoparticles for upconversion luminescence/MR multimodal imaging and magnetically targeted photothermal therapyBiomaterials20123372215222222169825
- ZhangLZhongXWangLT1-weighted ultrashort echo time method for positive contrast imaging of magnetic nanoparticles and cancer cells bound with the targeted nanoparticlesJ Magn Reson Imaging201133119420221182139
- PortilhoFEstevanatoLMiranda-VilelaAInvestigation of a magnetohyperthermia system efficacyJ Appl Phys2011109707B307
- BalivadaSRachakatlaRWangHA/C magnetic hyperthermia of melanoma mediated by iron (0)/iron oxide core/shell magnetic nanoparticles: a mouse studyBMC Cancer20101011920350328
- CarneiroMLNunesESPeixotoRCFree Rhodium (II) citrate and rhodium (II) citrate magnetic carriers as potential strategies for breast cancer therapyJ Nanobiotechnology201191121443799
- PrimoFLRodriguesMMSimioniARLacavaZGMoraisPCTedescoACPhotosensitizer-loaded magnetic nanoemulsion for use in synergic photodynamic and magnetohyperthermia therapies of neoplastic cellsJ Nanosci Nanotechnol20088115873587719198320
- TaylorRMHuberDLMonsonTCAliAMBisoffiMSillerudLOMultifunctional iron platinum stealth immunomicelles: targeted detection of human prostate cancer cells using both fluorescence and magnetic resonance imagingJ Nanopart Res201113104717472922121333
- QuanGDuXHuoTTargeted molecular imaging of antigen OC183B2 in ovarian cancers using MR molecular probesAcad Radiol201017121468147620869887
- GalanzhaEIShashkovEVKellyTKimJWYangLZharovVPIn vivo magnetic enrichment and multiplex photoacoustic detection of circulating tumour cellsNat Nanotechnol200941285586019915570
- ShimadaRIinumaHAkahaneTHoriuchiAWatanabeTPrognostic significance of CTCs and CSCs of tumor drainage vein blood in Dukes’ stage B and C colorectal cancer patientsOncol Rep201227494795322267181
- ZhaoYPRuanCPWangHIdentification and assessment of new biomarkers for colorectal cancer with serum N-glycan profilingCancer2012118363965021853445
- LiMLiJYZhaoALSurvival stratification panel of colorectal carcinoma with combined expression of carcinoembryonic antigen, matrix metalloproteinases-2, and p27 kip1Dis Colon Rectum200750111887189817882488
- ArtikoVPetrovićMSobić-SaranovićDRadioimmunoscin-tigraphy of colorectal carcinomas with 99 mTc-labelled antibodiesHepatogastroenterology20115810634735121661394
- AartsFBoermanOCSharkeyRMPretargeted radioimmunoscin-tigraphy in patients with primary colorectal cancer using a bispecific anticarcinoembryonic antigen CEA X anti-di-diethylenetriaminepentaacetic acid F (ab′) 2 antibodyCancer2010116Suppl 41111111720127959
- FrampasEMaurelCRemaud-Le SaëcPPretargeted radioimmunotherapy of colorectal cancer metastases: models and pharmacokinetics predict influence of the physical and radiochemical properties of the radionuclideEur J Nucl Med Mol Imaging201138122153216421858527
- HomayounfarKLierschTNiessnerMMultimodal treatment options for bilobar colorectal liver metastasesLangenbecks Arch Surg2010395663364120213463
- ValoisCRBrazJMNunesESThe effect of DMSA-functionalized magnetic nanoparticles on transendothelial migration of monocytes in the murine lung via a beta2 integrin-dependent pathwayBiomaterials201031236637419822361
- AcharyaSDilnawazFSahooSKTargeted epidermal growth factor receptor nanoparticle bioconjugates for breast cancer therapyBiomaterials200930295737575019631377
- FahlgrenABaranovVFrängsmyrLZoubirFHammarströmMLHammarströmSInterferon-gamma tempers the expression of carcinoembryonic antigen family molecules in human colon cells: a possible role in innate mucosal defenceScand J Immunol200358662864114636419
- WangHRajagopalSReynoldsSCederbergHChakrabartySDifferentiation-promoting effect of 1-O (2 methoxy) hexadecyl glycerol in human colon cancer cellsJ Cell Physiol1999178217317810048581
- KendallCDayJHutchingsJEvaluation of Raman probe for oesophageal cancer diagnosticsAnalyst2010135123038304120949209
- WajedSAPersonalized treatment-the promise of molecular genetics diagnosticsJ Gastrointest Surg201014Suppl 1S2S519768512
- BharaliDJMousaSAEmerging nanomedicines for early cancer detection and improved treatment: current perspective and future promisePharmacol Ther2010128232433520705093
- YigitMVMooreAMedarovaZMagnetic nanoparticles for cancer diagnosis and therapyPharm Res20122951180118822274558
- KimBHLeeNKimHLarge-scale synthesis of uniform and extremely small-sized iron oxide nanoparticles for high-resolution T1 magnetic resonance imaging contrast agentsJ Am Chem Soc201113332126241263121744804
- EstevanatoLCintraDBaldiniNPreliminary biocompatibility investigation of magnetic albumin nanosphere designed as a potential versatile drug delivery systemInt J Nanomedicine201161709171721980234
- BergJMHoSHwangWInternalization of carbon black and maghemite iron oxide nanoparticle mixtures leads to oxidant productionChem Res Toxicol201023121874188221067130
- ChenWYiPZhangYZhangLDengZZhangZComposites of aminodextran-coated Fe3O4 nanoparticles and graphene oxide for cellular magnetic resonance imagingACS Appl Mater Interfaces20113104085409121882840
- AnkamwarBLaiTCHuangJHBiocompatibility of Fe(3)O(4) nanoparticles evaluated by in vitro cytotoxicity assays using normal, glia and breast cancer cellsNanotechnology20102177510220090199
- FoySPLabhasetwarVOh the irony: Iron as a cancer cause or cure?Biomaterials201132359155915821963282
- Monge-FuentesVGarciaMPTavaresMCBiodistribution and biocompatibility of DMSA-stabilized maghemite magnetic nanoparticles in nonhuman primates (Cebus spp.)Nanomedicine (Lond)2011691529154421675858
- DriskellJDJonesCATompkinsSMTrippRAOne-step assay for detecting influenza virus using dynamic light scattering and gold nanoparticlesAnalyst2011136153083309021666913
- LingYWeiKLuoYGaoXZhongSDual docetaxel/superparamagnetic iron oxide loaded nanoparticles for both targeting magnetic resonance imaging and cancer therapyBiomaterials201132297139715021726899
- JansHLiuXAustinLMaesGHuoQDynamic light scattering as a powerful tool for gold nanoparticle bioconjugation and biomolecular binding studiesAnal Chem200981229425943219803497
- SatishkumarRVertegelAAAntibody-directed targeting of lysostaphin adsorbed onto polylactide nanoparticles increases its antimicrobial activity against S. aureus in vitroNanotechnology2011225050510322107797
- SolerMALimaECNunesESSpectroscopic study of maghemite nanoparticles surface-grafted with DMSAJ Phys Chem A201111561003100821261312
- ParkerFSApplications of Infrared, Raman, and Resonance Raman Spectroscopy in Biochemistry1st edNew YorkSpringer1983
- OcchipintiEVerderioPNatalelloAInvestigating the structural biofunctionality of antibodies conjugated to magnetic nanoparticlesNanoscale20113238739020877896
- ATCCCell lines and hybridomas [homepage on the Internet] Available from: http://www.atcc.org/ATCCAdvancedCatalogSearch/ProductDetails/tabid/452/Default.aspx?ATCCNum=CL-188&Template=cellBiologyAccessed March 5, 2012