Abstract
Background
The biocompatibility of two forms of calcined mesoporous silica particles, labeled as MCM41-cal and SBA15-cal, with fetal blood mononuclear cells was assessed in vitro.
Methods and results
Fetal mononuclear cells were isolated from umbilical cord blood and exposed to 0.5 mg/mL of MCM41-cal or SBA15-cal for several hours. Transmission electron micrographs confirmed the presence of particles in the cytosol of macrophages, neutrophils, and lymphocytes without noticeable damage to the cellular organelles. The particles (especially MCM41-cal) were in close proximity to plasma, and nuclear and mitochondrial membranes. Biocompatibility was assessed by a functional assay that measured cellular respiration, ie, mitochondrial O2 consumption. The rate of respiration (kc, in μM O2 per minute per 107 cells) for untreated cells was 0.42 ± 0.16 (n = 10), for cells treated with MCM41-cal was 0.39 ± 0.22 (n = 5, P > 0.966) and for cells treated with SBA15-cal was 0.44 ± 0.13 (n = 5, P > 0.981).
Conclusion
The results show reasonable biocompatibility of MCM41-cal and SBA15-cal in fetal blood mononuclear cells. Future studies are needed to determine the potential of collecting fetal cells from a fetus or neonate, loading the cells in vitro with therapeutic MCM41-cal or SBA15-cal, and reinfusing them into the fetus or neonate.
Introduction
The term “nanotoxicology” refers to the discipline that studies structural and functional cellular derangements produced by particles 1–100 nm in size.Citation1,Citation2 For example, treatment of lymphocytes with multiwalled carbon nanotubes (20–40 nm) induces apoptosis.Citation3 Further, some silica and water-soluble fullerene nanoparticles are reported to extract electrons from the mitochondrial respiratory chain, producing deleterious oxidative damage.Citation4–Citation7 Many of the toxic properties exhibited by nanomaterials are associated with ultrastructural alterations within the cell.Citation8 Not surprisingly, these changes are dependent on the dose, size, shape, surface area, aggregation, and surface functional conjugation of the materials.
Interestingly, a wide variety of other nanostructured and microstructured materials have been shown to be biocompatible with various cells and tissues.Citation9–Citation11 Many of them have also been investigated for biological and medical applications, such as drug deliveryCitation9,Citation10,Citation12–Citation17 and bioimaging.Citation8 A few types of nanoparticles have shown good promise and have been commercialized as smart delivery vehicles or diagnostic agents for various diseases. However, comprehensive in vitro studies of the effects of nanomaterials and micromaterials on fetal cells are still necessary before scientists and biomedical engineers can extend their use to the fetus and the neonate.
MCM-41 and SBA-15 mesoporous silica particles have a high surface area and monodispersed nanometer pores, typically about 2 nm and 8 nm in diameter, respectively.Citation18,Citation19 They are synthesized by supramolecular self-assembly of alkoxysilanes with cetyltrimethylammonium bromide (CTAB) surfactant or Pluronic 123 triblock copolymer templates. Removal of these organic templates is responsible for the formation of mesopores in the materials. Because of their attractive structures, with high surface area and pore volume, both MCM-41 and SBA-15 have been widely studied for delivery of small molecules, small interfering RNAs, and genes.Citation9,Citation10,Citation12–Citation17 Their biological properties (toxicity or biocompatibility) have also been widely investigated. Interestingly, contradictory results have been documented in a few studies. For instance, a recent in vivo study suggested efficient uptake and biocompatibility of mesoporous silica nanospheres in murine pneumatocytes and mesothelioma cells.Citation20 In contrast, toxicity of MCM-41 and SBA-15 in mesothelial cells has been reported, eg, intraperitoneal and intravenous injections of either particle produced pulmonary thromboembolic events.Citation21
Differences in dose, surface area, surface charge, pore diameter, residual reagents, and templates on the materials, as well as route of administration, have all been implicated as potential causes of conflicting results. For instance, differences in cellular bioenergetics, cellular uptake, distribution, and other adverse effects have been observed with a variety of mesoporous silica particles prepared by different synthetic methods.Citation4,Citation15,Citation17,Citation20,Citation21
In addition, the methods used for removing organic templates from mesoporous materials may produce mesoporous materials with different properties, as was discovered recently.Citation22 Generally, two methods are used to remove the organic templates from these mesoporous materials, ie, high-temperature calcination in air or simple solvent-washing (extraction). In our recent studies, we showed that MCM41-cal or SBA15-cal prepared by calcination did not inhibit cellular bioenergetics and were biocompatible in murine liver, lung, pancreas, kidney, and spleen tissue.Citation23 The particles also did not alter cellular configurations or organelles. However, their solvent-extracted counterparts inhibited pneumatocyte respiration. The differences between calcined and solvent-extracted mesoporous silicas were attributed to the lack of residual organic materials in the calcined particles, as well as to the less surface density of silanol (Si-OH) groups, which are often implicated in the toxicity of silicas.Citation24,Citation25
The present study investigated MCM41-cal and SBA15-cal particles in fetal mononuclear cells collected from umbilical cord blood immediately after delivery. The data overall show reasonable biocompatibility of calcined mesoporous silica particles in fetal mononuclear blood cells.
Materials and methods
Reagents
A Pd(II) complex of meso-tetra-(4-sulfonatophenyl)-tetrabenzoporphyrin was obtained from Porphyrin Products (Logan, UT). RPMI-1640 cell culture medium was sourced from Mediatech (Herndon, VA). Glucose oxidase (powder from Aspergillus niger), D (+) glucose anhydrous, Histopaque-1077, CTAB, tetraethyl orthosilicate, fluorescein 5(6)-isothiocyanate, and the remaining reagents were purchased from Sigma-Aldrich (St Louis, MO). Poly(ethylene oxide)-block-poly(butylene oxide)-block-poly(ethylene oxide) (Pluronic 123) [(PEO) 20(PPO) 70(PEO) 20] was purchased from BASF (Florham Park, NJ).
Solutions
Pd phosphor solution (2.5 mg/mL = 2 mM) was prepared in dH2O and stored at −20°C. Glucose oxidase solution was prepared in dH2O (10 mg/mL) and stored at −20°C. NaCN solution (1.0 M) was prepared in dH2O; the pH was adjusted to about 7.0 with 12 N HCl and the solution was stored at −20°C. Phosphate-buffered saline with glucose (137 mM NaCl, 2.7 mM KCl, 4.3 mM Na2HPO4, 1.4 mM KH2PO4, and 5 mM glucose; pH 7.4) was stored in the refrigerator for one week.
Isolation of peripheral blood mononuclear cells
Blood (5–10 mL) was collected from the umbilical cord immediately following delivery in heparinized syringes and processed within one hour of collection. Samples were diluted with an equal volume of phosphate-buffered saline plus 10 mM glucose and gently layered on top of 10 mL of Histopaque-1077. The mixtures were centrifuged at 15°C and 400 g for 30 minutes. The peripheral blood mononuclear cells collected were diluted with phosphate-buffered saline plus 10 mM glucose and centrifuged at 15°C and 400 g for 15 minutes. The pellets were suspended in phosphate-buffered saline plus 10 mM glucose, 3 μM Pd phosphor, and 0.5% fat-free bovine serum albumin. Cell count and viability were determined by light microscopy, using a hemocytometer under standard trypan blue staining conditions. Collection of blood from the umbilical cords was approved by the Institutional Review Board for Protection of Human Subjects. Informed consent was obtained for each participating subject.
Synthesis of MCM41-cal
MCM41 was synthesized as described previously.Citation18 Typically, CTAB (4.0 g, 1.1 mmol) was dissolved in Millipore water (960 mL) and 2.0 m NaOH solution (14 mL). After moderate stirring of the solution at 80°C for 30 minutes, tetraethyl orthosilicate (101.2 mmol) was added. The resulting solution was stirred for another 2 hours at 80°C. The hot solution was then filtered and the solid product was washed with Millipore water (4 × 80 mL), followed by ethanol (4 × 80 mL), and then dried in an oven at 80°C. This resulted in as-synthesized mesostructured MCM41. The CTAB surfactant template from the as-synthesized MCM41 was removed by calcination. Two grams of the as-synthesized MCM41 sample was calcined in a tube furnace at 550°C for 6 hours under airflow (the temperature was raised from room temperature to 550°C at a rate of 10°C per minute). This produced calcined MCM41 (labeled here as MCM41-cal). The properties of the particles are shown in and .
Table 1 Physical characteristics of MCM41-cal and SBA15-cal
Synthesis of SBA15-cal
SBA15 was prepared as reported previouslyCitation19 with slight modification. A solution containing 12 g of Pluronic 123, 313 g Millipore water, and 72 g HCl (36 wt%) was prepared and stirred vigorously at 40°C until the Pluronic template had dissolved. Tetraethyl orthosilicate 25.6 g was then added, and the solution was stirred at 45°C for 24 hours. After keeping it under static condition in the oven at 80°C for 24 hours, the solution was filtered. The solid material was washed with Millipore water and ethanol (3 × 80 mL) and dried under ambient conditions to produce as-synthesized SBA15 particles. The Pluronic 123 template from the as-synthesized SBA15 sample was removed by calcination as described above, and the resulting mesoporous sample was labeled as SBA15-cal. The particles were stored at 25°C in a vacuum desiccator until further use. The properties of the particles are shown in and .
Oxygen measurement
A phosphorescence analyzer that measures dissolved O2 in solutions as a function of time was used to determine the rate of cellular respiration. This method is based on the principle of O2 quenching the phosphorescence of palladium phosphor. The Pd (II) derivative of meso-tetra-(4-sulfonatophenyl)-tetrabenzoporphyrin has an absorption maximum at 625 nm and a phosphorescence emission maximum at 800 nm. Samples were exposed to light flashes (10 per second) from a pulsed light-emitting diode array with a peak output at 625 nm (OTL630A-5-10-66-E, Opto Technology Inc, Wheeling, IL). Emitted phosphorescent light was detected by a Hamamatsu photomultiplier tube after first passing the samples through a wide band interference filter centered at 800 nm. Amplified phosphorescence was digitized at 1–2 MHz using an analog/digital converter (PCI-DAS 4020/12 I/O board) with 1–20 MHz outputs (Computer Boards Inc, Mansfield, MA). Pulses were captured at 0.1–4.0 MHz, using a software program developed for this use.
The program was developed using Microsoft Visual Basic 6, Microsoft Access Database 2007, and Universal Library components. This allowed direct reading from the PCI-DAS 4020/12 I/O Board. The software included a relational database that stores experiments, pulses, and pulse metadata, including slopes. Pulse detection was accomplished by finding 10 phosphorescence intensities above 1.0 volt. Peak detection was accomplished by finding the highest 10% data points of a pulse, and then choosing the one in the group that was closest to the pulse decay curve. Depending on the sample rate, a minimum number of data points per pulse was set and used as a cutoff to remove invalid pulses with too few data points.
The phosphorescence decay rate (1/τ) was characterized by a single exponential, I = Ae−t/τ, where I = Pd phosphor phosphorescence intensity. The values of 1/τ were linear with dissolved O2: 1/τ = 1/τo + kq[O2], where 1/τ = the phosphorescence decay rate in the presence of O2, 1/τ° = the phosphorescence decay rate in the absence of O2, and kq = the second-order O2 quenching rate constant in sec−1 μM−1.
Cellular respiration was measured at 37°C in 1 mL sealed vials. Mixing was carried out with the aid of parylene-coated stirring bars. The respiratory substrates were endogenous metabolic fuels supplemented with glucose. In cell suspensions sealed from air, [O2] decreased linearly with time, indicating that the kinetics of mitochondrial O2 consumption were zero order. The rate of respiration (k, in μM O2 per minute) was thus the negative of the slope d[O2]/dt. Cyanide markedly inhibited respiration, confirming that O2 was consumed mainly by the mitochondrial respiratory chain. For samples that were each run three times, the coefficient of variation of rates of respiration was <12%. NaCN inhibited respiration ≥96%.
Transmission electron microscopy
Samples were immersed in McDowell and Trump fixative for 3 hours at 25°C. Tissues were rinsed with phosphate-buffered saline and fixed with 1% osmium tetroxide for one hour. Samples were then washed with dH2O, dehydrated in graded ethanol and propylene oxide, infiltrated, embedded in Agar 100 epoxy resin, and polymerized at 65°C for 24 hours. Blocks were trimmed, and semithin and ultrathin sections were cut using a Reichert Ultracuts ultramicrotome (Leica Microsystems GmbH, Wetzlar, Germany). The semithin (1 μm) sections were stained with 1% aqueous toluidine blue on glass slides. The ultrathin sections (95 nm) on 200 mesh copper grids were contrasted with uranyl acetate followed by a lead citrate double stain. The grids were examined and photographed under a Philips CM10 transmission electron microscope (TEM, Philips, Eindhoven, The Netherlands).
Results
MCM41-cal and SBA15-cal were synthesized using a surfactant supramolecular self-assembly method as described elsewhere.Citation18,Citation19 The surfactant templates from the synthesized mesoporous materials were removed by heating the samples at 550°C for 6 hours under a flow of air in a temperature-programmable tube furnace. The resulting calcined materials were labeled as MCM41-cal and SBA15-cal. The synthesized MCM41-cal and SBA15-cal were then characterized by various methods, including nitrogen gas adsorption, elemental analysis, TEM, and solid-state nuclear magnetic resonance spectroscopy. The characterization results for MCM41-cal and SBA15-cal are summarized in . The surface area and pore diameters of MCM41-cal and SBA15-cal were determined by N2 adsorption measurements (). N2 adsorption showed a type IV isotherm, indicating the presence of mesoporous silica. The surface area and average Barrett-Joyner-Halenda (BJH) pore diameter of MCM41-cal were found to be 1290 m2/g and 28 Å, respectively, whereas the surface area and average BJH pore diameter of SBA15-cal was 955 m2/g and 59 Å, respectively. TEM images of SBA15-cal and MCM41-cal are shown in . The TEM images show that the MCM41-cal material consisted of spherical or oval-shaped microparticles with sizes in the range of 300–1000 nm, whereas the SBA15-cal material consisted of irregularly shaped and micron-sized particles about 1000 nm long and having typical diameters of approximately 500 nm. The degree of condensation within the silica framework for MCM41-cal and SBA15-cal samples was determined using solid-stateCitation28 Si{1H} cross-polarization magic angle spinning. The spectrum for SBA15-cal is depicted in , which shows well defined (SiO)2Si(OH)2 (Q2), (SiO)3-SiOH (Q3), and (SiO)4-Si (Q4) silicate species, appearing at −94, −103, and −112 ppm, respectively.
shows the TEM micrographs of fetal blood mononuclear cells incubated with 500 μg/mL MCM41-cal particles for 2 hours. The number of MCM41-cal particles inside the cells was variable. Particles were seen inside the cytosol and engulfed by extended pseudopods. MCM41-cal was also seen in the cytosol of basophils without noticeable damage to the cellular organelles. MCM41-cal was seen in macrophages (Supplementary Figure 1A and B), neutrophil polymorphs (Supplementary Figure 1C–E), and lymphocytes (Supplementary Figure 1F). Particles were in the cytosol, either free (eg, Supplementary Figure 1E and F) or within vacuoles (eg, Supplementary Figure 1A). Some particles were attached to the plasma membrane (Supplementary Figure 1D–F), the nuclear membrane (with invaginations, Supplementary Figure 1B, C, and E), or the mitochondrial membrane (Supplementary Figure 1B–D).
Figure 2 Transmission electron microscopic images of fetal white blood cells incubated with 500 μg/mL MCM41-cal for 2 hours. The number of MCM41-cal (big arrows) inside the cells varied (Panels A–D). Panel A shows MCM41-cal inside the cytosol of a macrophage (large arrow) and another particle being engulfed by extended pseudopods (thin arrows). Panel D shows two MCM41-cal particles (big arrows) in the cytosol of a basophil; note the three lobes of the nucleus with a connecting strand (thin arrow) and large granules.
Note: Scale bar = 0.5 μm.

TEM micrographs of fetal blood mononuclear cells incubated with 500 μg/mL SBA15-cal for 2 hours are shown in and Supplementary Figure 2. Many particles were seen within the cytosol of macrophages (big arrows in ) or a neutrophil polymorphs (big arrows in ) without obvious damage to any organelle (big arrows in ). Many particles were seen in macrophages ( and Supplementary Figure 2A–C) and neutrophil polymorphs (Supplementary Figure 2D). Particles were within vacuoles (Supplementary Figure 2A and D), attached to lysosomes (Supplementary Figure 2C), or near mitochondrial membranes (Supplementary Figure 2B).
Figure 3 Transmission electron microscopic images of fetal blood mononuclear cells incubated with 500 μg/mL SBA15-cal for 2 hours. (A) Many SBA15-cal were seen within a vacuole in the cytosol of a macrophage without any obvious damage to any organelle (thick arrows). (B) Many SBA15-cal particles were seen within the cytosol of a macrophage and some of them were seen adherent to mitochondria without any obvious damage (thick arrow). (C) Many SBA15-cal were in the cytosol of a macrophage (thick arrow), in contact with a lysosome (thin arrow) without any evidence of phagosome-lysosome fusion. (D) Many SBA15-cal particles seen within a vacuole in the cytosol of a neutrophil polymorph, without any obvious damage to the organelles (thick arrows). Small arrows in (C) and (D) indicate artifacts.
Note: Scale bar = 0.5 μm.
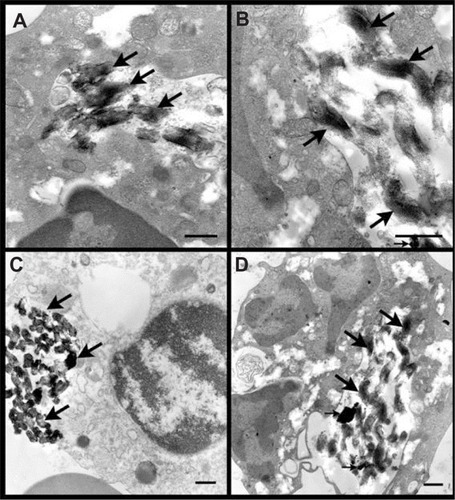
Representative experiments for fetal mononuclear cell respiration in the presence and absence of MCM41-cal are shown in and of SBA15-cal in . In , fetal cells (9.6 × 107 cells/mL) were suspended in approximately 3 mL of phosphate-buffered saline, 10 mM glucose, 3 μM Pd phosphor, and 0.5% fat-free bovine serum albumin. The mixture was divided into two equal aliquots. MCM41-cal (0.5 mg/mL) was added to one aliquot with gentle stirring. The contents of each reaction were then transferred to a 1 mL size O2 vial, which was sealed and placed in the instrument for O2 measurement at 37°C. The O2 concentration was determined every 0.6 seconds. The two O2 vials were alternated in the instrument every 5 minutes for a total of about 95 minutes. Thus, each [O2] data point represented the mean ± standard deviation of approximately 3000 determinations. The rate of respiration (k, in μM O2 per minute) was set as the negative of the slope of [O2] versus t. The value of k (in μM O2 per minute) for the untreated sample was 2.7 (R2 = 992) and for the treated sample was 1.9 (R2 = 975). In , fetal mononuclear cell (2.8 × 107 cells/mL) respiration was determined exactly as above. The value of k for the untreated sample was 1.8 (R2 = 996) and for the treated sample was 1.7 (R2 = 996). The results of a total of five independent experiments (expressed in μM O2 per minute per 107 cells) are shown in . As shown, fetal cell respiration was unaffected by MCM41-cal (P > 0.966).
Table 2 Respiration of fetal blood mononuclear cells with and without MCM41-cal or SBA15-cal
Figure 4 Representative experiments of fetal blood mononuclear cell respiration in the presence and absence of MCM41-cal.
Notes: All procedures were performed immediately following collection of venous blood from the umbilical cord. Respiration was measured in cells isolated from the whole blood with and without the addition of 0.5 mg/mL MCM41-cal. O2 measurements were performed at 37°C in 1 mL sealed vials, alternating every 5 minutes between untreated (closed circles) and MCM41-cal (open circles) samples; each time point represents mean ± standard deviation (n = 1800) of [O2] over 3 minutes. The rate of respiration (k) was the negative of the slope of [O2] versus t; the values of k (in μM O2 per minute) are shown. The lines are linear fits. Zero minutes corresponds to addition of particles.
![Figure 4 Representative experiments of fetal blood mononuclear cell respiration in the presence and absence of MCM41-cal.Notes: All procedures were performed immediately following collection of venous blood from the umbilical cord. Respiration was measured in cells isolated from the whole blood with and without the addition of 0.5 mg/mL MCM41-cal. O2 measurements were performed at 37°C in 1 mL sealed vials, alternating every 5 minutes between untreated (closed circles) and MCM41-cal (open circles) samples; each time point represents mean ± standard deviation (n = 1800) of [O2] over 3 minutes. The rate of respiration (k) was the negative of the slope of [O2] versus t; the values of k (in μM O2 per minute) are shown. The lines are linear fits. Zero minutes corresponds to addition of particles.](/cms/asset/e1b8b515-a59b-476d-bbb6-506509221ddf/dijn_a_32711_f0004_b.jpg)
Figure 5 Representative experiments of fetal blood mononuclear cell respiration in the presence and absence of SBA15-cal.
Notes: All procedures were performed immediately following collection of venous blood from the umbilical cord. Respiration was measured in cells isolated from whole blood with and without the addition of 0.5 mg/mL SBA15-cal. O2 measurements were performed at 37°C in 1 mL sealed vials, alternating every 5 minutes between untreated (closed circles) and SBA15-cal (open circles) samples; each time point represents the mean ± standard deviation (n = 1800) of [O2] over 3 minutes. The rate of respiration (k) was the negative of the slope of [O2] versus t; the values of k (in μM O2 per minute) are shown. The lines are linear fits. Zero minutes corresponds to addition of particles.
![Figure 5 Representative experiments of fetal blood mononuclear cell respiration in the presence and absence of SBA15-cal.Notes: All procedures were performed immediately following collection of venous blood from the umbilical cord. Respiration was measured in cells isolated from whole blood with and without the addition of 0.5 mg/mL SBA15-cal. O2 measurements were performed at 37°C in 1 mL sealed vials, alternating every 5 minutes between untreated (closed circles) and SBA15-cal (open circles) samples; each time point represents the mean ± standard deviation (n = 1800) of [O2] over 3 minutes. The rate of respiration (k) was the negative of the slope of [O2] versus t; the values of k (in μM O2 per minute) are shown. The lines are linear fits. Zero minutes corresponds to addition of particles.](/cms/asset/7a4437ea-a0d1-4395-b80b-039827873d8b/dijn_a_32711_f0005_b.jpg)
The same experiments were repeated with 0.5 mg/mL SBA15-cal. In , fetal mononuclear cell (7.2 × 107 cells/mL) respiration, determined exactly as above, was 3.8 μM O2 per minute (R2 = 997) for the untreated sample, and 3.7 μM O2 per minute (R2 = 995) for the treated sample. In , fetal mononuclear cell (18.3 × 107 cells/mL) respiration for the untreated sample was 5.4 μM O2 per minute (R2 = 974) and for the treated sample was 4.8 μM O2 per minute (R2 = 988). The results of a total of five independent experiments (expressed in μM O2 per minute per 107 cells) are shown in . As shown, fetal cell respiration was unaffected by SBA15-cal (P > 0.981).
Additional results
TEM micrographs of fetal blood mononuclear cells incubated with 500 μg/mL MCM41-cal for 2 hours are shown in Supplementary Figure 1. No obvious damage to organelles was noted in any of the micrographs studied. MCM41-cal was seen in macrophages (Panels A and B), neutrophil polymorphs (Panels C–E), and lymphocytes (Panel F). Particles were seen in the cytosol, either free (eg, Panels E and F) or within vacuoles (eg, Panel A). Some particles were attached to the plasma membrane (Panels D–F), nuclear membrane (with clear invaginations, panels B, C, and E) and mitochon-drial membrane (Panels B–D). There was an apparent attraction of MCM41-cal (eg, Supplementary Figure 1B) to the nuclear and mitochondrial membranes, a feature that could facilitate transferring particle contents to these organelles.
TEM micrographs of fetal blood mononuclear cells incubated with 500 μg/mL SBA15-cal for 2 hours are shown in Supplementary Figure 2. Similarly, no noticeable organelle damage was seen in any of the micrographs studied. Many SBA15-cal particles were seen in macrophages (Panels A–C) and neutrophil polymorphs (Panel D). Many particles were within vacuoles (Panels A and D) or attached to a lysosome (Panel C) or the mitochondrial membrane (Panel B). SBA15-cal was in contact with a lysosome without any evidence of phagosome-lysosome fusion (Supplementary Figure 2, Panel C). This phenomenon suggests a possible role for this type of particle in preventing this fusion, and could be beneficial from two points of view. In one view, it could inhibit lysosomal enzyme activation and consequent cell damage. In another view, inhibiting lysosomal enzymes could reduce damage to the particle contents.
Discussion
Mesoporous silica nanomaterials and micromaterials have prospective clinical use for delivering bioactive compounds.Citation27 In this study, the biocompatibility of two forms of calcined mesoporous silica particles, ie, MCM41-cal and SBA15-cal, was investigated in fetal cells collected from umbilical cord blood. These micromaterials were prepared using different amphiphilic templates, ie, CTAB and Pluronic 123, respectively. MCM41-cal has a spherical or oval shape, whereas SBA15-cal has an irregular rod shape. In addition, MCM41 shows smaller lattice spacing and smaller BJH pore diameters ( and ). Despite these differences, both micromaterials have similar potential biological applications, as a result of their large surface areas, large pore volumes, microscale sizes, nanometer pores, and suitable properties for loading and delivering drugs, genes, and other bioactive molecules. Furthermore, calcined mesoporous silica particles are shown to be bioavailable and biocompatible.Citation22,Citation23,Citation28
MCM41-cal and SBA15-cal were present inside fetal macrophages, neutrophils, and lymphocytes ( and , and Figures S1 and S2). Their engulfment occurred without a need for surface activation by organic or polymeric groups, as previously reported for similar materials in other tissues.Citation9,Citation10,Citation20,Citation29–Citation31 Uptake was likely mediated by the high affinity of phospholipids in the plasma membrane for particle surface groups, such as silanols and silanolate.Citation25,Citation32–Citation34
The TEM images ( and , and Figures S1 and S2) show that MCM41-cal and SBA15-cal are distributed in different cellular compartments and are abundant in macrophages. They are also seen in different areas of the cytoplasm, suggesting intracellular movement. The particles do not appear to disturb cellular configuration or organelles. Some firmly attach to the nuclear membrane, seen as indentations. A similar observation of particles within the cytoplasm but not getting inside the nucleus has been reported in murine tissuesCitation28 and in 3T3-L1 fibroblasts for chromophore-functionalized mesoporous materials.Citation27
Particle aggregates are noted inside some fetal cells, especially for rod-shaped SBA15-cal. Particle aggregation in cellular cytosol for rod-shaped mesoporous silica (CTAB template of about 450 nm size and aspect ratio of approximately 107 nm) has been previously reported.Citation35 Nevertheless, despite differences in shape, the results here show similar engulfment of MCM41-cal and SBA15-cal into fetal cells.
Compared with solvent-extracted particles, the calcined MCM41 and SBA15 used here have low-density surface silanols (as evident by 28Si solid-state nuclear magnetic resonance spectroscopy). Thus, they are expected to generate fewer silanol-induced free radicals.Citation24–Citation26 Moreover, absence of residual organics in the calcined materials (), due to the calcination procedure, might have contributed to their biocompatibility in fetal cells.
Another aim of this study was to investigate the biocom-patibility of MCM41 and SBA15 with fetal blood cell bioenergetics, ie, cellular mitochondrial O2 consumption. Recent studies have reported their safety in vitro,Citation23 but Hudson et al have described in vitro toxicity when using relatively high doses of MCM41 and SBA15 in mesothelial cells.Citation21
Cellular mitochondrial oxygen consumption (respiration) implies delivery of O2 and metabolic fuels to the mitochondria, oxidation of reduced metabolic fuels with release of electrons to O2, and synthesis of adenosine triphosphate. Impaired respiration thus entails an interference with any of these processes.Citation22 Normal cellular respiration, on the other hand, implies well preserved glucose uptake and glycolysis, steady delivery of O2 and metabolic fuels to the mitochondria, an intact mechanism and rate of oxidation of reduced metabolic fuels in the mitochondrial respiratory chain, normal passage of electrons to O2, and coupled synthesis of adenosine triphosphate. Thus, the biochemical method and data are appropriate for assessing biocompatibility. The use of such cellular bioenergetics to assess nanoparticle and microparticle biocompatibility is discussed in more detail in our previous publications.Citation22,Citation23,Citation28 The results of our present work show that respiration in fetal mononuclear blood cells in the presence of MCM41-cal and SBA15-cal is similar to that of untreated cells, indicating reasonable biocompatibility between calcined mesoporous silica particles and fetal cellular bioenergetics ( and and ).
The results show intact respiration (bioenergetics) of fetal blood cells treated with MCM41-cal or SBA15-cal for several hours. These findings are consistent with prior in vitro studies of biocompatibility of these two mesoporous silica particles in murine tissues.Citation23,Citation28 This compatibility could be attributable to the refined method of particle preparation, including the lack of residual surfactant molecules as well as less density of surface silanols (). MCM41 and SBA15, with their amphiphilic templates extracted by solvent washing, were not found to be biocompatible with lymphoid and myeloid hematopoietic cells.Citation22 These findings emphasize the impact of synthetic procedures on nanoparticle cytotoxicity and the need for careful assessment of the preparation protocols.
The biocompatibility of MCM41-cal and SBA15-cal in fetal blood cells is demonstrated here, primarily based on ultrastructure features and measurements of cellular bioenergetics,Citation23,Citation36 but further studies using different fetal tissues and functional and structural methodologies are needed to confirm these preliminary results.
TEM studies are the gold standard for assessing ultra-structural changes, and the results of these analyses are consistent with the biochemical (functional) data described earlier. The TEM images also clearly show abundant internalization of the particles inside cells, along with well preserved organelles.
It is worth noting that, despite their different sizes (), the two silica particles used here showed the same degree of biocompatibility. Unlike with traditional materials, findings with regard to biocompatibility of nanomaterials and micro-materials are difficult to generalize and require particle-specific investigation. This stems from the fact that composition, size, shape, surface charge, surface properties (hydrophilicity versus hydrophobicity), residual materials, method of preparation, and other structural features all impact biological properties and thus biocompatibility and cytotoxicity.
Among various structural and compositional factors, the size of nanomaterials and micromaterials plays a critical role. The impact of two different sizes of silica nanotubes on normal and cancer cells has been investigated. Particle size was found to influence both uptake and cytotoxicity. At the same concentration, 200 nm nanotubes were more toxic than 500 nm nanotubes.Citation37 Size-dependent neurotoxicity of copper nanomaterials (40–80 nm) was demonstrated in murine somatosensory neurons, with smaller particles being more toxic than larger ones.Citation38
The cytotoxicity of nanosized and microsized CuO materials was studied in a human alveolar cell line.Citation39 The 42 nm particles were significantly more toxic to the mito-chondria and DNA than were the 3 μm particles. However, the same study also showed that Fe2O3 (<1 μm) and TiO2 (1 μm) particles caused more DNA damage than their corresponding nanoparticles (29 nm and 63 nm, respectively). Thus, it is reasonable to conclude that the biological effects of particles are influenced by numerous variables including size, composition, and host cells.
In summary, this study suggests that MCM41-cal and SBA15-cal are reasonably biocompatible with fetal mononuclear blood cells. The results are consistent with other studies using cell culture or in vivo systems with longer exposure times.Citation2 The results also show well preserved mononuclear blood cell structure (by electron microscopy) and function (by cellular respiration) after treatment with either type of material, confirming biocompatibility. The benign properties of MCM41-cal and SBA15-cal are likely due to the refined methods of synthesis and calcination, resulting in fewer surface hydroxyl groups and free residual surfactants (as evident by the solid-state nuclear magnetic resonance data).
Acknowledgements
We thank the labor and delivery staff at Tawam Hospital for supporting this project. This research is supported by a grant from the United Arab Emirates University.
Disclosure
The authors report no conflicts of interest in this work.
Supplementary figures
Figure S1 Transmission electron microscopic images of fetal blood mononuclear cells incubated with 500 μg/mL MCM41-cal for 2 hours. (A) MCM41-cal particles were seen within vacuoles (thick arrows), in the cytosol of a macrophage, not obviously attached to any organelle. (B) MCM41-cal was seen within a vacuole (thin arrow) and strictly attached to the nuclear membrane of a macrophage with clear invagination of the nuclear membrane in the area of adherence without obvious damage (thick arrow), another MCM41-cal was seen adherent to a mitochondrion without any obvious damage (thin arrow). (C) MCM41-cal was in a neutrophil polymorph, within a vacuole (thick arrow), adhering to the nucleus and mitochondria (thin arrow) without causing any obvious damage. (D) MCM41-cal particles were in the cytosol of a neutrophil polymorph, within vacuoles and close to mitochondria (thick arrow). (E) MCM41-cal was seen in the cytosol of a neutrophil polymorph closely adhering to the nucleus (thick arrow) and another particle was seen passing the cell membrane without any obvious damage (thick arrow). (F) Two MCM41-cal particles were seen in the cytosol of a lymphocyte after crossing the cell membrane without any obvious damage (thick arrow).
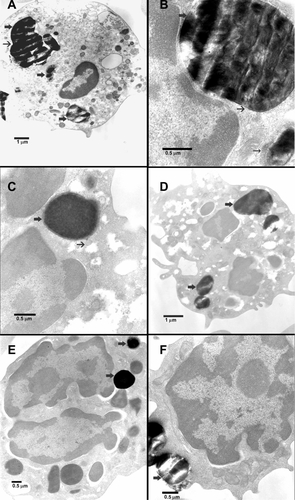
Figure S2 Transmission electron microscopic images of fetal blood mononuclear cells incubated with 500 μg/mL SBA15-cal for 2 hours. (A) Many SBA15-cal were seen within a vacuole in the cytosol of a macrophage without any obvious damage to any organelle (thick arrows). (B) Many SBA15-cal particles were seen within the cytosol of a macrophage and some of them were seen adherent to mitochondria without any obvious damage (thick arrow). (C) Many SBA15-cal were in the cytosol of a macrophage (thick arrow), in contact with a lysosome (thin arrow) without any evidence of phagosome-lysosome fusion. (D) Many SBA15-cal particles were within a vacuole in the cytosol of a neutrophil polymorph without any obvious damage to the organelles (thick arrows).
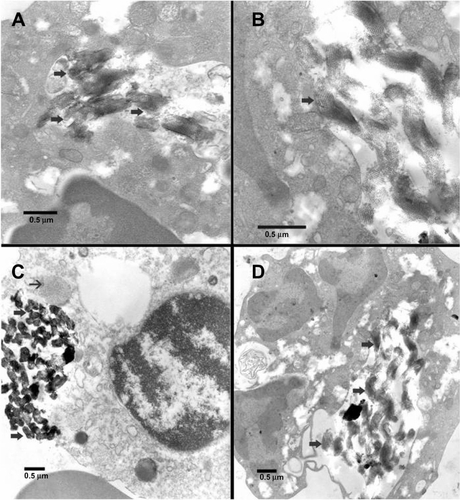
References
- KipenHMLaskinDLSmaller is not always better: nanotechnology yields nanotoxicologyAm J Physiol Lung Cell Mol Physiol20052895L696L70816214820
- NelAXiaTMadlerLLiNToxic potential of materials at the nanolevelScience2006311576162262716456071
- BottiniMBrucknerSNikaKMulti-walled carbon nanotubes induce T lymphocyte apoptosisToxicol Lett2006160212112616125885
- LinWHuangYWZhouXDMaYIn vitro toxicity of silica nanoparticles in human lung cancer cellsToxicol Appl Pharmacol2006217325225917112558
- SayesCMGobinAMAusmanKDMendezJWestJLColvinVLNano-C60 cytotoxicity is due to lipid peroxidationBiomaterials200526367587759516005959
- SlowingITrewynBGLinVSEffect of surface functionalization of MCM-41-type mesoporous silica nanoparticles on the endocytosis by human cancer cellsJ Am Chem Soc200612846147921479317105274
- Di PasquaAJSharmaKKShiYLCytotoxicity of mesoporous silica nanomaterialsJ Inorg Biochem200810271416142318279965
- HesslerJABudorAPutchakayalaKAtomic force microscopy study of early morphological changes during apoptosisLangmuir200521209280928616171363
- RosenholmJMPeuhuEErikssonJESahlgrenCLindenMTargeted intracellular delivery of hydrophobic agents using mesoporous hybrid silica nanoparticles as carrier systemsNano Lett2009993308331119736973
- XiaTKovochichMLiongMPolyethyleneimine coating enhances the cellular uptake of mesoporous silica nanoparticles and allows safe delivery of siRNA and DNA constructsACS Nano20093103273378619739605
- RosenholmJMPeuhuEBate-EyaLTErikssonJESahlgrenCLindenMCancer-cell-specific induction of apoptosis using mesoporous silica nanoparticles as drug-delivery vectorsSmall20106111234124120486218
- Vallet-RegiMBalasFArcosDMesoporous materials for drug deliveryAngew Chem Int Ed Engl200746407548755817854012
- LuJLiongMShermanSMesoporous silica nanoparticles for cancer therapy: energy-dependent cellular uptake and delivery of Paclitaxel to cancer cellsNanobiotechnology200732899519936038
- SlowingIITrewynBGLinVSYMesoporous silica nanoparticles for intracellular delivery of membrane-impermeable proteinsJ Am Chem Soc2007129288845884917589996
- LiongMLuJKovochichMMultifunctional inorganic nanoparticles for imaging, targeting, and drug deliveryACS Nano200825889889619206485
- RosenholmJMMeinanderAPeuhuETargeting of porous hybrid silica nanoparticles to cancer cellsACS Nano20093119720619206267
- HomCLuJLiongMMesoporous silica nanoparticles facilitate delivery of siRNA to shutdown signaling pathways in mammalian cellsSmall20106111185119020461725
- KresgeCTLeonowiczMERothWJVartuliJCBeckJSOrdered mesoporous molecular sieves synthesized by a liquid-crystal template mechanismNature19923596397710712
- ZhaoDFengJHuoQTriblock copolymer syntheses of mesoporous silica with periodic 50–300 angstrom poresScience199827953505485529438845
- BlumenSRChengKRamos-NinoMEUnique uptake of acid-prepared mesoporous spheres by lung epithelial and mesothelioma cellsAm J Respir Cell Mol Biol200736333334217038662
- HudsonSPPaderaRFLangerRKohaneDSThe biocompatibility of mesoporous silicatesBiomaterials200829304045405518675454
- TaoZMorrowMPAsefaTMesoporous silica nanoparticles inhibit cellular respirationNano Lett2008851517152618376867
- Al ShamsiMAl SamriMTAl-SalamSBiocompatibility of calcined mesoporous silica particles with cellular bioenergetics in murine tissuesChem Res Toxicol201123111796180520961102
- KonecnyRLeonardSShiXRobinsonVCastranovaVReactivity of free radicals on hydroxylated quartz surface and its implications for pathogenicity experimental and quantum mechanical studyJ Environ Pathol Toxicol Oncol200120Suppl 111913211570669
- MurashovVHarperMDemchukEImpact of silanol surface density on the toxicity of silica aerosols measured by erythrocyte haemolysisJ Occup Environ Hyg200631271872317133693
- JohnstonAPBattersbyBJLawrieGATrauMPorous functionalised silica particles: a potential platform for biomolecular screeningChem Commun (Camb)200521784885015700057
- LinYSTsaiCPHuangHYWell-ordered mesoporous silica nanoparticles as cell markersChem Mater2005171845704573
- Al-SalamSBalhajGAl-HammadiSIn vitro study and biocom-patibility of calcined mesoporous silica microparticles in mouse lungToxicol Sci20111221869921470958
- BeyerleAIrmlerMBeckersJKisselTStoegerTToxicity pathway focused gene expression profiling of PEI-based polymers for pulmonary applicationsMol Pharm20127372773720429563
- GalagudzaMMKorolevDVSoninDLTargeted drug delivery into reversibly injured myocardium with silica nanoparticles: surface functionalization, natural biodistribution, and acute toxicityInt J Nanomedicine2010523123720463939
- RaduDRLaiCYJeftinijaKRoweEWJeftinijaSLinVSA polyami-doamine dendrimer-capped mesoporous silica nanosphere-based gene transfection reagentJ Am Chem Soc200412641132161321715479063
- AllisonACHaringtonJSBirbeckMAn examination of the cytotoxic effects of silica on macrophagesJ Exp Med196612421411544288309
- ChunboYDaqingZAizhuoLJiazuanNA NMR study of the interaction of silica with dipalmitoylphosphatidylcholine liposomesJ Colloid Interface Sci19951722536538
- MurashovVVLeszczynskiJAdsorption of the phosphate groups on silica hydroxyls: an initio studyJ Phys Chem A1999103912281238
- TsaiCPHungYChouYHHigh-contrast paramagnetic fluorescent mesoporous silica nanorods as a multifunctional cell-imaging probeSmall20084218619118205156
- Al SamriMTAl ShamsiMAl SalamSMeasurement of oxygen consumption by murine tissues in vitroJ Pharmacol Toxicol Methods201163219620421034836
- NanABaiXSonSJLeeSBGhandehariHCellular uptake and cytotoxicity of silica nanotubesNano Lett2008882150215418624386
- PrabhuBMAliSFMurdockRCHussainSMSrivatsanMCopper nanoparticles exert size and concentration dependent toxicity on somatosensory neurons of ratNanotoxicology20104215016020543894
- KarlssonHLGustafssonJCronholmPMöllerLSize-dependent toxicity of metal oxide particles – a comparison between nano- and micrometer sizeToxicol Lett2009188211211819446243