Abstract
Background
One of the critical problems in cancer management is local recurrence of disease. Between 20% and 30% of patients who undergo tumor resection surgery require reoperation due to incomplete excision. Currently, there are no validated methods for intraoperative tumor margin detection. In the present work, we demonstrate the potential use of gold nanoparticles (GNPs) as a novel contrast agent for photothermal molecular imaging of cancer.
Methods
Phantoms containing different concentrations of GNPs were irradiated with continuous-wave laser and measured with a thermal imaging camera which detected the temperature field of the irradiated phantoms.
Results
The results clearly demonstrate the ability to distinguish between cancerous cells specifically targeted with GNPs and normal cells. This technique, which allows highly sensitive discrimination between adjacent low GNP concentrations, will allow tumor margin detection while the temperature increases by only a few degrees Celsius (for GNPs in relevant biological concentrations).
Conclusion
We expect this real-time intraoperative imaging technique to assist surgeons in determining clear tumor margins and to maximize the extent of tumor resection while sparing normal background tissue.
Introduction
Cancer is a leading cause of death worldwide.Citation1 One of the critical problems in cancer management is local recurrence of disease. Between 20% and 30% of patients who undergo tumor resection surgery require reoperation because of incomplete excision.Citation2,Citation3 Currently, tumor margins are examined using conventional histological tests which are usually performed following the surgical procedure. A few methods have been developed in recent years for real-time intraoperative tumor margin detection with some success, including diffusive reflectance,Citation4,Citation5 radiofrequency-based detection,Citation6 and targeted fluorescence imaging.Citation7 However, there remains an urgent need to develop a highly specific and sensitive intraoperative real-time tumor margin detection method which will reduce the risk of cancer recurrence and the need for reoperation.
During the last decade, much research has focused on developing nanoparticles which enhance scattering or absorption in the near infrared region because of the relatively high transmittance of tissue in that region. Particles such as fluorescence dyes, gold nanoshells, and gold nanorods have been utilized either as diagnostic toolsCitation8–Citation10 or as photothermal therapy-mediated agents.Citation8,Citation9,Citation11–Citation14
Gold nanorods have gained much attention as a diagnostic tool due to their biocompatibility, their relatively easy fabrication and bioconjugation with biomolecules for targeting, their unique optical properties, and enhanced optical extinction coefficient as compared with conventional fluorescence dyes;Citation15,Citation16 by changing their size and aspect ratio, their surface plasmon resonance wavelength and absorption to scattering coefficient ratio can be tuned and controlled.Citation15 Therefore, they have been widely utilized as near infrared,Citation14 photoacoustic,Citation17 Raman scattering,Citation8 and diffusion reflectionCitation18,Citation19 imaging contrast agents.
In addition to research regarding early detection of cancer, gold nanorods have been utilized as photothermal therapy-mediated agents. In these studies, the absorption properties of gold nanorods in the surface plasmon resonance wavelength are used to elevate temperature to 50°C and above, usually using lasers with a high optical output (above 10 W/cm2 for about 20 minutes) in order to achieve effective denaturation and coagulation of cellular proteins, as well as cell death.Citation11,Citation12,Citation20,Citation21
An in vitro proof of concept for photothermal imaging using targeted gold nanoparticles (GNPs) is demonstrated in this paper. By selectively increasing the temperature of GNPs that specifically target and decorate the surface of cancer cells, we can distinguish between cancerous and noncancerous cells (ie, normal background tissue). We show that this technique has the ability to discriminate sensitively between adjacent concentrations of GNPs, while the temperature of the sample is elevated by only a few degrees Celsius. This is important because elevation of temperature to above 40°C may denature proteins and release heat shock proteins.Citation11
Using a thermal camera, the photothermal imaging technique overcomes the inevitable background signal caused by light scattering from tissue. Another important advantage of this imaging technique is the use of the absorption properties of GNPs rather than their scattering properties. Imaging techniques based on the scattering properties of GNPs suffer from relatively high background noise and low contrast due to the relatively high scattering of tissue. By utilizing only the absorption properties of GNPs, higher contrast between the targeted cancer cells and normal background tissue can be achieved.
Materials and methods
Nanoparticle synthesis and characterization
Gold nanospheres with a diameter of 30 nm were synthesized using sodium citrate according to the methodology described by Enüstün and Turkevich.Citation22 Gold nanorods (25 nm × 65 nm) were synthesized using the seed-mediated growth method.Citation23 Particle size, shape, and uniformity were measured using transmission electron microscopy. For the bioconjugation process, a protective layer of poly(ethylene glycol) (PEG) was adsorbed onto the surfaces of the gold nanospheres in order to prevent aggregation. The PEG layer consisted of a mixture of heterofunctional PEGs (SH-PEG-OCH3, about 85%, molecular weight approximately 5 kDa and an SH-PEG-COOH, about 15%, molecular weight approximately 3.4 kDa, Creative PEGWorks, Winston-Salem, NC). For cancer cell targeting, heterofunctional PEGs were covalently conjugated with an anti-epithelial growth factor receptor (EGFR) monoclonal antibody (Erbitux®, Merck KGaA, Darmstadt, Germany), using 1-ethyl-3-(3′-(dimethylamino) propyl)carbodiimide and sulfo-NHS (Thermo Fisher Scientific, Waltham, MA).Citation24,Citation25 Bioconjugation of the gold nanorods to the anti-EGFR antibody was achieved according to the method described by Lvov et al,Citation26 using polystyrene sulfonate.
Phantoms
Solid phantoms were prepared in order to simulate the optical properties of skin tissue.Citation27 Phantoms were prepared using 3 × 10−3 percent of India ink as an absorbing component and 2% of Intralipid (Lipofundin MCT/LCT 20%, B Braun Melsungen AG, Melsungen, Germany) as a scattering component.Citation28 Gold nanospheres (30 mg/mL) or gold nanorods (5 mg/mL) were added to the phantom solutions, achieving final gold concentrations of 0.002, 0.02, 0.022, 0.03, 0.04, and 0.1 mg/mL.
In order to prepare solid phantoms, the solutions were heated and mixed at a temperature of approximately 90°C while 1% agarose powder (SeaKem LE Agarose, Lonza, Allendale, NJ) was slowly added. The heated phantom solutions were cooled under vacuum conditions to avoid formation of bubbles. All phantoms were poured into a 24-well plate (16 mm diameter wells), each well containing different concentrations of gold.
Cell preparation and bioconjugation
A431 human head and neck cancer cells (2.5 × 106), which are known to express an extremely high level of EGFR,Citation29 were cultured in 5 mL of Dulbecco’s modified Eagle’s medium containing 5% fetal calf serum, 0.5% penicillin, and 0.5% glutamine at 37°C under 5% CO2. For cell-GNP conjugation, 1 mL of cell suspension (2.5 × 106/mL) was mixed with 1 mL of antibody-coated gold nanorod solution (5 mg/mL), and allowed to interact for 30 minutes at room temperature. Then, the solution was centrifuged three times at 1000 rpm for 5 minutes to wash out unbound gold nanorods, and after each centrifugation, the mixture was redispersed in phosphate-buffered solution (1 mL total volume).
Experimental setup
This experiment was designed to image the distribution of temperature over the sample area under laser illumination. The laser beam was directed at the sample from above, as shown in . Two different lasers were used: one, a red diode laser at wavelength of 663 nm (custom built), and the other, a green Nd:YAG diode pumped solid state laser at 532 nm (Suwtech Laser, DPGL2200 Photop, Fuzhou, China). In accordance with the peak absorption spectrum of GNPs, the red laser was used for samples with gold nanorods and the green one for samples with gold nanospheres.
Temperature elevation over the sample was imaged using a radiometric thermal imaging camera (FLIR Systems Inc, Boston, MA, model A325). The camera has 320 × 240 pixels and a temperature sensitivity of 0.07°C. The spatial resolution of the camera is 0.5 mm. By adding an extra lens, it can achieve a spatial resolution of 0.1 mm at a working distance of 80 mm. This kind of camera is sensitive to thermal radiation at a wavelength range of 8–14 μm, and is completely blind to lasers and other light sources at the visible or near infrared spectral range. For each experiment, a few seconds of ambient temperature were recorded (at the center of the laser beam). Next, the samples were irradiated until reaching a saturation temperature. The irradiance was measured to be 10 W/cm2 at the center of the beam.
Results and discussion
GNP synthesis and characterization
shows the absorbance spectra (ultraviolet-visible spectrometer, Shimadzu, UV1650 PC, Tokyo, Japan) of the gold nanorods and gold nanospheres, and the wavelength of the lasers used (532 nm and 663 nm spectrophotometer, USB2000, Ocean Optics Inc, Dunedin FL). The absorption peaks of gold nanospheres and gold nanorods are around 515 nm and 690 nm, respectively. Particle size, shape, and uniformity were measured using transmission electron microscopy, showing 30 nm gold nanospheres and 25 nm × 65 nm gold nanorods, with a narrow size distribution (10%, , right). In order to evaluate the potential of tumor margin delineation using targeted GNP with photothermal imaging techniques, phantoms containing different concentrations of gold nanospheres and gold nanorods, and cancer cells (A431) that were specifically targeted with gold nanorods, were irradiated, and their heating profiles were measured.
Figure 2 Left: Absorbance spectra of gold nanospheres (green) and gold nanorods (dashed red).
Notes: The wavelengths of the lasers are shown by the arrows. Right: transmission electron microscopy image of 30 nm gold nanospheres (upper figure, scale bar 100 nm) and 25 nm × 65 nm gold nanorods (lower figure, scale bar 100 nm).
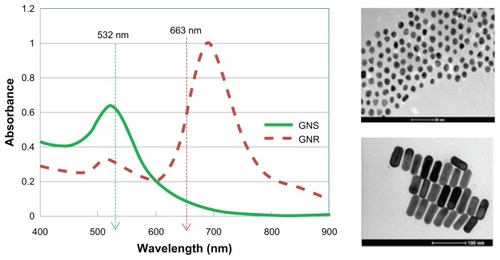
Photothermal imaging of phantoms with GNPs
shows the temperature difference profiles for irradiated gold nanorod solutions as a function of time for different concentrations of gold nanorods. As demonstrated, after less than 5 seconds of laser irradiation, the temperature profiles of the irradiated gold nanorod solutions were significantly different than those of the control solution without gold nanorods. The temperature of the control sample remained unchanged during the experiment, while the temperature of the gold nanorod samples was elevated. In addition, it can be seen that there was a positive correlation between the gold nanorod concentrations and temperature elevation. For the highest gold nanorod concentration (0.1 mg/mL), a temperature difference of about 14°C was observed after 10 seconds of irradiation, while for the lowest detectable concentration (0.02 mg/mL), a concentration that is clinically relevant,Citation12,Citation18,Citation30 the temperature was elevated by about 1°C after the same period of time.
Figure 3 Temperature elevation as a function of irradiation time with the 663 nm laser for different concentrations of gold nanorods in aqueous solution.
Note: The graph reaches a plateau after approximately 5 seconds.

The effect of laser wavelength on the heating properties of the gold nanospheres and gold nanorods was further investigated using green and red lasers. A red laser was used because the near infrared region of the spectrum provides maximal penetration of light due to relatively lower scattering and absorption from intrinsic tissue chromophores. In this region, the penetration depth of red light is up to 10 cm, depending on the type of tissue.Citation31 In comparison, the tissue penetration depth of green light (532 nm) is very low (less than 500 μm),Citation31 which could be useful for superficial lesions and margin detection during surgery.
Different concentrations of gold nanospheres (absorption peak at 515 nm) and gold nanorods (absorption peak at 690 nm) were irradiated with both 663 nm and 532 nm lasers for 30 seconds. As expected, the temperature profile was strongly affected by the optical properties of the nanoparticles. When gold nanospheres (0.1 mg/mL) were irradiated with 532 nm, the temperature increased by 5.5°C after 5 seconds, while following irradiation with the 663 nm laser for the same period of time, a temperature elevation of only 2.5°C was observed (). depicts similar results for gold nanorods; when gold nanorods (0.1 mg/mL) were irradiated with 663 nm, the temperature increased by 14°C after 5 seconds, while following irradiation with the 532 nm laser for the same period of time, a temperature elevation of only 4.5°C was observed. The temperature elevation of the samples when irradiated with lasers not at the GNP resonance peak can be explained by the tail of the resonance peak of the gold nanospheres at 690 nm and the “short axis” gold nanorod peak at 510 nm. In addition, because of the larger cross-section of the gold nanorods compared with that of the gold nanospheres,Citation15 the gold nanorods had a higher absorption efficiency, which was converted to thermal energy ().
Figure 4 Temperature elevation as a function of concentrations of gold nanospheres (A) and gold nanorods (B) for the two lasers.
Notes: The time point for comparison was after 5 seconds of laser irradiation, when the temperature curves had reached a plateau. The laser optical intensity on the sample was 10 W/cm2 for both lasers.

This study further investigated the potential of this imaging modality to delineate tumor margins during surgery. Two half-cylindrical solid phantoms, one with gold nanorods (0.04 mg/mL) and the other a phantom without gold nanorods, were bonded and irradiated with the 663 nm laser. As demonstrated in , after 10 seconds of irradiation, the gold nanorod phantom region and the homogenous phantom are easily distinguishable. The temperature in the gold nanorod region was elevated by 3.5°C after 10 seconds of irradiation (), and by 9°C after 5 minutes of irradiation (). This concentration of gold nanorods was found to be clinically relevant in several in vivo studiesCitation32,Citation33 and, therefore, demonstrates the ability to sensitively delineate tumor margins during surgery.
Figure 5 Thermal images of a sample made of two half-cylindrical solid phantoms joined together, one with gold nanorods and the other without. Image (A) was taken before laser irradiation. The border between the phantoms is shown by a dashed straight line. The elliptical contour shows the place where the laser beam hits the sample. Images (B) and (C) were taken after 10 seconds and 5 minutes of laser irradiation, respectively.
Note: It can be seen that the temperature in the part of the sample with the gold nanorods rises compared with that in the part without gold nanorods.
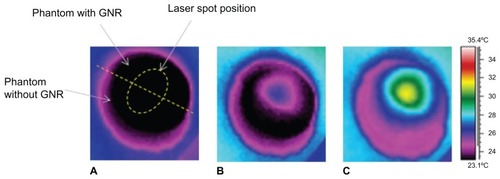
Photothermal imaging of gold nanorodtargeted cancer cells
In order to demonstrate the ability to perform photothermal imaging using targeted gold nanorods, the specificity of the interaction between the antibody-coated gold nanorods and the A431 SCC cancer cells (which highly express epithelial growth factor) was evaluated. Two types of gold nanorods were introduced to the cells; the first was specifically coated with anti-EGFR antibody, while the second, which was used as a negative control, was coated with a non-specific antibody (anti-rabbit IgG). Flame atomic absorption spectroscopy measurements (SpectrAA 140, Agilent Technologies, Santa Clara, CA) quantitatively demonstrated that active tumor targeting (anti-EGFR-coated gold nanorods) was significantly more specific than the control experiment (anti-rabbit IgG coated gold nanorods). The A431 cells took up 26.3 ± 2.3 μg of targeted gold nanorods (3.9 × 104 gold nanorods per A431 cell), while parallel cells in the negative control experiment took up only 0.2 ± 0.01 μg of gold nanorods (3.4 × 103 gold nanorods per cell). Our results correlate well with those from previously published studies which report that head and neck squamous cell carcinoma expresses from 2 × 104 to 2 × 106 EGFR per cell.Citation34,Citation35
For photothermal imaging studies, gold nanorod-A431 bioconjugated cancerous cells (2.5 × 106/mL) and control samples (A431 cells without gold nanorods) were irradiated for 6 minutes. As shown in , in about 5 seconds, there is a distinct difference of 1°C between the gold-targeted cells and the non-targeted cells. Following 30 seconds of laser irradiation, a temperature difference of 3°C has been observed, which is an appropriate temperature for imaging without affecting cell viability.Citation36
Conclusion
In summary, this in vitro study demonstrates the feasibility of photothermal imaging of tumor margins when targeted with GNPs. By reducing the laser irradiation, GNP-targeted cancer cells can be detected without affecting cell viability. It has been shown that a low concentration of GNPs (0.02 mg/mL), lower than that found in several in vivo studies,Citation18,Citation32,Citation33 can be easily detected. An important advantage of this imaging technique is the use of the absorption properties of GNPs rather than their scattering properties, leading to high contrast between targeted cancer cells and normal background tissue.
The possibility of combining photothermal imaging with photothermal therapy is an exciting approach toward theranostics. Additional laser irradiation following the detection of residual tumors adjacent to the primary tumor would lead to selective destruction of the cancer cells targeted with GNPs within the same clinical setting. We expect this in vitro proof of concept study will eventually lead to an intraoperative photothermal imaging technique which will assist surgeons in determining tumor margins accurately during surgery, leading to complete tumor resection and improved patient outcome.
Acknowledgments
This work was supported by the Elias, Genevieve, and Geogianna Atol Charitable Trust and the Katz Family Grant Incentive Program. The authors would like to thank Rinat Ankry and Vital Peretz for their help in the preparation of the GNPs and the phantoms.
Disclosure
The authors report no conflicts of interest in this work.
References
- World Health OrganizationCancer Fact Sheet2972009 Available from: http://www.who.int/mediacentre/factsheets/fs297/en/index.htmlAccessed July 23, 2012
- SmittMCNowelsKCarlsonRWJeffreySSPredictors of reexcision findings and recurrence after breast conservationInt J Radiat Oncol Biol Phys200357497998514575828
- MenesTTartterPBleiweissIGodboldJEstabrookASmithSThe consequence of multiple re-excisions to obtain clear lumpectomy margins in breast cancer patientsAnn Surg Oncol2005121188188516195834
- WilkeLGBrownJQBydlonTMRapid noninvasive optical imaging of tissue composition in breast tumor marginsAm J Surg2009198456657419800470
- BrownJQBydlonTMRichardsLMOptical assesssment of tumor resection margins in the breastIEEE J Sel Top Quantum Electron201016353054421544237
- AllweisTMKaufmanZLelcukSA prospective, randomized, controlled, multicenter study of a real-time, intraoperative probe for positive margin detection in breast-conserving surgeryAm J Surg2008196448348918809049
- van DamGMThemelisGCraneLMAIntraoperative tumor- specific fluorescence imaging in ovarian cancer by folate receptor-[alpha] targeting: first in-human resultsNat Med201117101315131921926976
- von MaltzahnGCentroneAParkJHSERS-coded gold nanorods as a multifunctional platform for densely multiplexed near-infrared imaging and photothermal heatingAdv Mater200921313175318020174478
- LooCLoweryAHalasNWestJDrezekRImmunotargeted nanoshells for integrated cancer imaging and therapyNano Lett20055470971115826113
- HuangXQianWEl-SayedIHEl-SayedMAThe potential use of the enhanced nonlinear properties of gold nanospheres in photothermal cancer therapyLasers Surg Med200739974775317960762
- TerentyukGSMaslyakovaGNSuleymanovaLVLaser-induced tissue hyperthermia mediated by gold nanoparticles: toward cancer phototherapyJ Biomed Opt200914202101619405729
- von MaltzahnGParkJHAgrawalAComputationally guided photothermal tumor therapy using long-circulating gold nanorod antennasCancer Res20096993892390019366797
- TongLWeiQWeiAChengJXGold nanorods as contrast agents for biological imaging: optical properties, surface conjugation and photothermal effectsPhotochem Photobiol2009851213219161395
- GobinAMLeeMHHalasNJJamesWDDrezekRAWestJLNear-infrared resonant nanoshells for combined optical imaging and photothermal cancer therapyNano Lett2007771929193417550297
- JainPKLeeKSEl-SayedIHEl-SayedMACalculated absorption and scattering properties of gold nanoparticles of different size, shape, and composition: applications in biological imaging and biomedicineJ Phys Chem B2006110147238724816599493
- AraiTHayashiTNakamuraNBiology Society1998Proceedings of the 20th Annual International Conference of the IEEEOctober 29 to November 1, 1998
- KimCChoECChenJIn vivo molecular photoacoustic tomography of melanomas targeted by bioconjugated gold nanocagesACS Nano2010484559456420731439
- AnkriRDuadiHMotieiMFixlerDIn-vivo tumor detection using diffusion reflection measurements of targeted gold nanorods – a quantitative studyJ Biophotonics20125326327322234916
- AnkriRPeretzVMotieiMPopovtzerRFixlerDA new method for cancer detection based on diffusion reflection measurements of targeted gold nanorodsInt J Nanomedicine2012744945522334777
- GlazerESCurleySARadiofrequency field-induced thermal cytotoxicity in cancer cells treated with fluorescent nanoparticlesCancer2010116133285329320564640
- BirisASBoldorDPalmerJNanophotothermolysis of multiple scattered cancer cells with carbon nanotubes guided by time-resolved infrared thermal imagingJ Biomed Opt200914202100719405720
- EnüstünBVTurkevichJCoagulation of colloidal goldJ Am Chem Soc1963852133173328
- NikoobakhtBEl-SayedMAPreparation and growth mechanism of gold nanorods (NRS) using seed-mediated growth methodChem Mater20031519571962
- QianXPengX-HAnsariDOIn vivo tumor targeting and spectroscopic detection with surface-enhanced Raman nanoparticle tagsNat Biotechnol2008261839018157119
- WangXQianXBeitlerJJDetection of circulating tumor cells in human peripheral blood using surface-enhanced Raman scattering nanoparticlesCancer Res20117151526153221212408
- AiHFangMJonesSALvovYMElectrostatic layer-by-layer nano-assembly on biological microtemplates: plateletsBiomacromolecules20023356056412005529
- DamJSPedersenCBDalgaardTFabriciusPEArunaPAndersson-EngelsSFiber-optic probe for noninvasive real-time determination of tissue optical properties at multiple wavelengthsAppl Opt20014071155116418357101
- CubedduRPifferiATaroniPTorricelliAValentiniGA solid tissue phantom for photon migration studiesPhys Med Biol19974210197119799364593
- HaiglerHAshJFSingerSJCohenSVisualization by fluorescence of binding and internalization of epidermal growth-factor in human carcinoma cells A-431Proc Natl Acad Sci U S A197875733173321356052
- LiZHuangPZhangXRGD-conjugated dendrimer-modified gold nanorods for in vivo tumor targeting and photothermal therapyMol Pharm2009719410419891496
- WeisslederRA clearer vision for in vivo imagingNat Biotechnol200119431631711283581
- HainfeldJFO’ConnorMJDilmanianFASlatkinDNAdamsDJSmilowitzHMMicro-CT enables microlocalisation and quantification of Her2-targeted gold nanoparticles within tumour regionsBr J Radiol201184100252653321081567
- HainfeldJFSlatkinDNFocellaTMSmilowitzHMGold nanoparticles: a new x-ray contrast agentBr J Radiol20067993924825316498039
- ToddRWongDTWEpidermal growth factor receptor (EGFR) biology and human oral cancerHistol Histopathol199914249150010212811
- StantonPRichardsSReevesJEpidermal growth-factor receptor expression by human squamous-cell carcinomas of the head and neck, cell-lines and xenograftsBr J Cancer19947034274338080726
- EvdoninAKinevATsupkinaNGuerrieroVRaynesDAMedvedevaNExtracellular HspBP1 and Hsp72 synergistically activate epidermal growth factor receptorBiol Cell2009101635136018986301