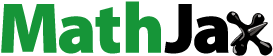
Abstract
Background
Chelation therapy involving organic chelators for treatment of heavy metal intoxication can cause cardiac arrest, kidney overload, mineral deficiency, and anemia.
Methods
In this study, superparamagnetic iron oxide nanoparticles (SPIONs) modified with an edible biopolymer poly(γ-glutamic acid) (PGA) were synthesized by coprecipitation method, characterized and evaluated for their removal efficiency of heavy metals from a metal solution, and simulated gastrointestinal fluid (SGIF).
Results
Instrumental characterization of bare- and PGA-SPIONs revealed 7% coating of PGA on SPIONs with a spherical shape and an iron oxide spinel structure belonging to magnetite. The particle sizes as determined from transmission electron microscopy images were 8.5 and 11.7 nm for bare- and PGA-SPIONs, respectively, while the magnetization values were 70.3 and 61.5 emu/g. Upon coating with PGA, the zeta potentials were shifted from positive to negative at most of the environmental pH (3–8) and biological pH (1–8), implying good dispersion in aqueous suspension and favorable conditions for heavy metal removal. Batch studies showed rapid removal of lead and cadmium with the kinetic rates estimated by pseudo-second-order model being 0.212 and 0.424 g/mg·min, respectively. A maximum removal occurred in the pH range 4–8 in deionized water and 5–8 in SGIF corresponding to most gastrointestinal pH except for the stomach. Addition of different ionic strengths (0.001–1 M sodium acetate) and essential metals (Cu, Fe, Zn, Mg, Ca, and K) did not show any marked influence on lead removal by PGA-SPIONs, but significantly reduced the binding of cadmium. Compared to deionized water, the lead removal from SGIF was high at all pH with the Langmuir monolayer removal capacity being 98.70 mg/g for the former and 147.71 mg/g for the latter. However, a lower cadmium removal capacity was shown for SGIF (23.15 mg/g) than for deionized water (31.13 mg/g).
Conclusion
These results suggest that PGA-SPIONs could be used as a metal chelator for clinical treatment of metal poisoning.
Introduction
Numerous industrial activities and modern agricultural practices have led to the accumulation of toxic heavy metals in the human body via the food chain. Nonessential heavy metals such as lead, cadmium, mercury, arsenic, and aluminum are poisonous to cardiovascular, nervous, hematopoietic, immunological, and gastrointestinal systems.Citation1–Citation3 Additionally, they can cause kidney dysfunction, anemia, liver toxicity, cancer, and Alzheimer’s disease.Citation2–Citation5 Because of chemical similarities, these heavy metals also act as effective competitors for essential metals and interact with several divalent metal transporters causing disruption of various physiological functions.Citation2,Citation3 Therefore, it is imperative to develop a potential and safe detoxifying agent for the treatment of metal intoxication.
Currently, chelation therapy involving organic chelators such as ethylenediaminetetraacetic acid (EDTA), meso-2, 3-dimercaptosuccinic acid, and British anti-lewisite is the preferred method for treatment of patients with heavy metal poisoning.Citation6–Citation8 Upon administration into the body, the organic chelators bind with heavy metals for eventual removal through the kidneys; however, these methods require multiple administrations, which may burden and damage the renal system during clearance of metal chelates from the body.Citation9 In addition, these chelating agents are capable of binding to essential metals, resulting in severe mineral deficiency and anemia.Citation10 For example, the occurrence of cardiac arrest due to hypocalcemia was reported in patients undergoing EDTA chelation therapy.Citation11 Thus, developing an alternative solid-phase adsorbent capable of selectively binding toxic heavy metals without affecting essential metals absorption would be therapeutically beneficial. Moreover, such solid-phase adsorbents administered orally would facilitate the removal of toxic metals from the gastrointestinal tract, resulting in their subsequent elimination through feces.Citation9
Consequently, over a decade, dietary fibers from various food products such as rice bran, wheat bran, oat bran, and fruit fibers have been evaluated as alternatives to conventional organic chelators.Citation12,Citation13 Nevertheless, recent advancements in the field of nanoscience and nanotechnology have paved the way for the tailored synthesis of nanoparticles by coating or encapsulating them with functional materials. Iron oxide nanoparticles are particularly attractive as nanosorbents for toxin removal owing to their superparamagnetic behavior and large surface area.Citation14–Citation16 The advantages of superparamagnetic iron oxide nanoparticles (SPIONs) are low diffusion resistance, fast and efficient separation within a short time by employing a simple magnet, and they can be magnetically driven to the specific organs of application;Citation14–Citation16 but, they tend to aggregate in solution due to dipole–dipole, hydrophobic, and van der Waals interactions, limiting their application in various fields.Citation16 To overcome this problem, iron oxide nanoparticles are often coated with a variety of functional materials such as surfactants or polymers, which has been well reviewed in the literature.Citation16 More recently, several functionalized SPIONs were evaluated for removal of heavy metals, including chitosan-, humic acid-, dimercaptosuccinic acid-, oleic acid-, and polyrhodanine-functionalized SPIONs for lead, cadmium, mercury, copper, arsenic, chromium, and manganese.Citation14,Citation15,Citation17–Citation19 However, their application as possible metal chelators for clinical treatment of metal intoxication still remains to be explored.
In this study, we intended to synthesize poly(γ-glutamic acid) (PGA) functionalized SPIONs and evaluate their ability to remove heavy metals in vitro. The PGA is an edible and biodegradable natural biopolymer composed of numerous repetitive glutamic acid units connected by γ-peptide linkage, leaving the α-carboxyl groups free for conjugation to a variety of compounds (−[−NH−CH(COOH)−(CH2)2−CO−]n−).Citation20,Citation21 It is extracellularly synthesized by Bacillus species, by either the de nova method through solid state fermentation, or by the salvage bioconversion method in submerged fermentation.Citation20,Citation21 Because of its unique γ-amide linkage, multifunctionality, and flexibility to be synthesized in different ionic forms (H, Ca, Na, Mg, etc) with varying molecular weights (10,000 to 2 million Daltons), PGA finds wide application in many different fields such as food, medicine, agriculture, hygiene, cosmetics, and the environment.Citation20,Citation21 Our previous studies have demonstrated the efficiency of PGA as an adsorbent for the removal of heavy metals, organic dyes, and heterocyclic amines;Citation22–Citation25 however, the application of PGA-functionalized SPIONs for removal of heavy metals remains unknown. The objectives of this study were to synthesize, characterize, and evaluate the efficiency of PGA-SPIONs in removing heavy metals like lead and cadmium from both a metal solution and simulated gastrointestinal fluid.
Materials and methods
Materials
Iron salts ferric chloride (FeCl3 · 6H2O) and ferrous sulfate (FeSO4 · 7H2O) for the synthesis of SPIONs were purchased from Nacalai Tesque (Kyoto, Japan); ammonium hydroxide (28%, w/w) was procured from JT Baker (Phillipsburg, NJ); and hydrochloric acid was bought from Riedel de Haën (Seelze, Germany). The sodium form of PGA with low MW (∼250 kDa) synthesized from Bacillus subtilis var Natto by a salvage bioconversion pathway in a submerged fermentation process was supplied by Vedan Enterprise Corporation (Taichung, Taiwan). An overhead mechanical stirrer (IKA® RW 20; IKA Works, Inc, Staufen, Germany) was used for vigorous stirring during the synthesis of SPIONs.
To prevent oxidation of iron salts during SPIONs synthesis, the milli-Q deionized water from EMD Millipore (Bedford, MA) was deoxygenated by bubbling N2 gas prior to use. The synthesized SPIONs were dried by using a vacuum incubator from Hong Sheng Instruments (Taipei, Taiwan). The nitrate salt of lead (Pb(NO3)2) and sulfate salt of cadmium (3CdSO4 · 8H2O) used for metal removal experiments were from Sigma-Aldrich (St Louis, MO). Several other metal salts, namely, potassium chloride and magnesium(II) sulfate heptahydrate were purchased from Nacalai Tesque, Inc (Kyoto, Japan); copper(II) sulfate and zinc(II) sulfate heptahydrate were obtained from Sigma-Aldrich; calcium(II) chloride dihydrate was procured from JT Baker; and iron(II) sulfate heptahydrate was purchased from Ajax Finechem Pty Ltd (Sydney, Australia). Chemicals such as nitric acid, sodium hydroxide and, sodium bicarbonate used for adjusting the pH of both the metal solution and the simulated gastrointestinal fluid were obtained from Lab-Scan Analytical Sciences (Bangkok, Thailand), Nacalai Tesque, and Sigma-Aldrich, respectively. A neodymium-iron-boron (Nd-Fe-B) magnet from New Favor Industry Co, Ltd (Taipei, Taiwan) was used for decanting the SPIONs after both the synthesis and metal removal experiments. The chemicals used for preparation of the synthetic gastrointestinal fluid such as sodium chloride and 700 FIP U/g pepsin (a digestive enzyme) were bought from JT Baker and Merck and Co, Inc (Taipei, Taiwan), respectively.
Syntheses of bare-SPIONs and PGA-SPIONs
Syntheses of both bare- and PGA-SPIONs was based on a conventional co-precipitation method as described by Inbaraj et al.Citation26 An aqueous mixture of 6.1 g ferric chloride and 4.2 g ferrous sulfate was prepared in deoxygenated deionized water, and a few drops of concentrated HCl were added for complete dissolution of iron salts. The mixture was then vigorously stirred at 2000 rpm with simultaneous heating to 85°C and bubbling of nitrogen gas to prevent oxidation of ferrous ions. At 85°C, 14 mL of ammonium hydroxide were rapidly added to coprecipitate ferrous and ferric ions, and the color of solution eventually changed from light orange into black to produce SPIONs. For coating with PGA, 50 mL of 0.5 g NaPGA dissolved in deoxygenated deionized water was subsequently added, with stirring and nitrogen gas bubbling being continued for 1 hour at 85°C. Upon completion of the reaction, the precipitated PGA-SPIONs were cooled at room temperature, followed by magnetic decanting of the supernatant fluid using a Nd-Fe-B magnet, washing several times with deoxygenated deionized water until the supernatant became neutral, and vacuum-drying at 0 mm Hg and 40°C for 24 hours for further characterization. Bare-SPIONs were synthesized by adopting the same procedure as described above but without the addition of PGA, and the chemical reaction involved can be represented as:
Characterization of bare-SPIONs and PGA-SPIONs
Both bare- and PGA-SPIONs were characterized for the elucidation of PGA functionalization and percentage coating on SPIONs, as well as for structure, particle size, surface morphology, magnetic property, and surface charge at different solution pH levels. Fourier transform infrared spectroscopy (FTIR) of bare- and PGA-SPIONs were recorded on a Horiba FTIR spectrophotometer (FT 730; Horiba, Ltd, Kyoto, Japan) in the frequency range from 4000–400 cm−1 by mixing the sample with KBr crystals and pelletizing at a pressure of 150 kg/cm2. An average of 32 interferograms at a resolution of 2 cm−1 was measured for each sample. For determination of structure, the X-ray diffraction (XRD) pattern was recorded on a Multiflex model Rigaku diffractometer (Rigaku; Multiflex, Tokyo, Japan) in the 2θ range of 20°–70° using Cu–Kα radiation (λ = 1.540556 Å) at 40 kV and 40 mA. The mean particle size and surface morphology were determined by imaging bare- and PGA-SPIONs in a JEOL transmission field emission electron microscope (TEM, JEM 2100F; JEOL, Ltd, Tokyo, Japan) at 80 kV. Prior to imaging, a drop of diluted aqueous solution of each sample was spread on a 150 mesh copper TEM grid (Ted Pella Inc, Redding, CA) and vacuum-dried for 1 hour.
To determine the weight percentage of PGA coating on SPIONs, each sample weighing 5–15 mg was heated from 25°C–700°C in a versa Therm HS model Cahn thermogravimetric analyzer (Thermo Fisher Scientific Inc, Waltham, MA) at a heating rate of 10°C/min. Magnetization measurements of SPIONs were carried out at room temperature (301 K) in the applied magnetic field range from +13,500 to −13,500 Oe by employing a DMS 1660 model vibrating sample magnetometer (MicroSense, LLC, Lowell, MA). A Malvern Zetasizer Nano ZS (Malvern Instruments Ltd, Worcestershire, UK) was used for measuring the zeta potential of bare- and PGA-SPIONs by dispersing 0.1 g/L of each sample in 0.001 M NaCl solutions adjusted to different pH values (2, 3, 4, 5, 6, 7, and 8). The concentration of synthesized SPIONs was determined by digesting samples at room temperature with sequential addition of concentrated HCl and concentrated HNO3 at a ratio of 2:1 (v/v) and aspirating into a GBC 932 model flame atomic absorption spectrophotometer (FAAS; GBC Scientific Equipment Manufacturer Pty, Ltd, Melbourne, Australia) for iron analysis.
Metal removal experiments and analysis
Initially, 1000 mg/L stock solutions of lead and cadmium were prepared separately and diluted to desired working solutions of different concentrations by using deionized water. The metal removal experiments were carried out by taking 10 mL of each metal solution in a 20 mL glass vial and adjusting the solutions to a desired pH using a Suntex 701 pH meter (Suntex Instruments Co, Ltd, Taipei, Taiwan) coupled with a Horiba 9611-10D glass electrode (Horiba Instruments Inc, CA). Then, 0.5 mL of 0.2 g/L PGA-SPIONs were added and shaken in a Firstek B601D reciprocating water bath shaker (Firstek Scientific Co, Tau-Yen, Taiwan) at 150 rpm at 37°C. After predetermined time intervals, the metal-loaded PGA-SPIONs were magnetically decanted using a Nd-Fe-B magnet, and the residual unbound metal in the solution was analyzed by FAAS. Control experiments were also performed to estimate the possible metal binding on the glass vials.
Initially, a hollow cathode lamp was inserted into a lamp socket in FAAS with wavelength, slit width, and lamp current set at 283.3 nm, 0.2 nm and 5 mA for lead and 228.8 nm, 0.2 nm, and 3 mA for cadmium, respectively. Air-acetylene gas mixture with an oxidizing flame at a ratio of 5:1 was employed. From respective stock solutions, four concentrations each of lead (5, 20, 35, and 50 mg/L) and cadmium (0.5, 1, 2, and 3 mg/L) were prepared for plotting calibration curves, and were then aspirated into FAAS after initial optimization of instrumental parameters. The levels of lead and cadmium in the supernatants obtained after magnetically decanting PGA-SPIONs were automatically determined based on the calibration curves.
Metal removal at different pH levels
To study the influence of solution pH on metal removal, 10 mL each of lead and cadmium solutions at 50 mg/L were adjusted to different pH values at 1, 1.5, 2, 2.5, 3, 3.5, 4, 5, 6, 7, and 8 separately and shaken with 0.5 mL of 0.2 g/L PGA-SPIONs for 2 hours at 37°C. After 2 hours, the metal-loaded PGA-SPIONs were magnetically decanted for analyzing free lead and cadmium in the solution.
Metal removal kinetics
The kinetics of metal removal was studied by taking 10 mL each of the lead and cadmium solutions at 50 mg/L, followed by adjusting pH to 5.5, adding 0.5 mL of 0.2 g/L PGA-SPIONs, and shaking the samples at 37°C for different time intervals of 1, 2, 3, 5, 10, 30, 60, and 120 minutes. After each time interval, a portion of the sample was removed for magnetic decantation of metal-loaded PGA-SPIONs and the supernatant was eventually analyzed for lead or cadmium.
Metal removal isotherms
For developing binding isotherms, 10 mL each of lead and cadmium concentrations at 10, 20, 30, 40, 50, 60, 80, and 100 mg/L were adjusted to a pH level of 5.5. Next, 0.5 mL of 0.2 g/L PGA-SPIONs was added and shaken for 2 hours at 37°C. At equilibrium, the unbound metal in the solution was analyzed by FAAS after magnetically decanting the PGA-SPIONs.
Metal removal at different ionic strengths
Based on a method used by Yantasee et al,Citation9 the effect of ionic strength in the biological matrix of metal removal by PGA-SPIONs was studied by spiking 50 mg/L of lead or cadmium solution in four different concentrations of sodium acetate (0.001, 0.01, 0.1, and 1 M) and mixing each with 0.5 mL of 0.2 g/L PGA-SPIONs. The mixtures were then shaken for 2 hours at 37°C, followed by magnetically decanting the metal-bound PGA-SPIONs for the analysis of free lead and cadmium in the solution.
Lead or cadmium removal as affected by essential metals
Selective removal of lead or cadmium by PGA-SPIONs in the presence of several biologically important essential metals was studied in a bisolute system by incorporating potassium, calcium, magnesium, zinc, iron or copper into the metal solution at a ratio of 1:1 (lead/cadmium:essential metal). The binary mixture of four different concentrations of lead or cadmium and each of the essential metals at 5, 25, 50, and 100 mg/L was adjusted to pH 5.5 and shaken with 0.5 mL of 0.2 g/L PGA-SPIONs at 37°C for 2 hours.
Metal removal from simulated gastrointestinal fluid
Simulated gastrointestinal fluid (SGIF) was prepared daily by adopting the procedure outlined by US Pharmacopeia for drug dissolution studies,Citation27 and modifying the procedure based on a method described by Yantasee et al.Citation9 The SGIF solution consisted of 0.03 M NaCl, 0.085 M HCl, and 0.32% (w/v) pepsin. For evaluating the effectiveness of PGA-SPIONs towards the removal of lead or cadmium at different gastrointestinal pH, 50 mg/L lead or cadmium solution was spiked into SGIF solutions and adjusted to different pH values (1, 2, 2.5, 3, 3.5, 4, 5, 6, 7, and 8) using 0.2 M sodium bicarbonate. To each, 0.5 mL of 0.2 g/L PGA-SPIONs was added, followed by shaking at 37°C for 2 hours, magnetically decanting the metal-bound PGA-SPIONs, and analyzing the supernatant for free lead or cadmium. In comparison with the isotherms obtained in deionized water, metal removal isotherms were also developed by preparing eight different concentrations (10, 20, 30, 40, 50, 60, 80, and 100 mg/L) each of lead and cadmium in SGIF, adjusting to pH 5.5, and adding 0.5 mL of 0.2 g/L PGA-SPIONs. The mixtures were then shaken at 37°C for 2 hours, and the metal-laden PGA-SPIONs were magnetically decanted to analyze residual lead and cadmium in the solution.
Data analyses
Metal removal experiments were done in duplicate and the average values, which showed a maximum deviation of only ±5%, were used for calculation and modeling. Metal removal capacity (q, mg/g) at different shaking times was determined based on the mass balance equation q = (Ci − C)/(V/W), where Ci (mg/L) is the initial metal concentration, C (mg/L) is the metal concentration in solution after time t (min), V (L) is the volume of metal solution, and W (g) is the weight of PGA-SPIONs.Citation22,Citation24 Likewise, metal binding at equilibrium can be calculated using the same equation described above by replacing C with Ce and q with qe, where Ce and qe are the metal concentration (mg/L) in the solution and removal capacity (mg/g) at equilibrium, respectively. Modeling of experimental data on metal removal kinetics and isotherms were performed by a nonlinear regression method using a GNUPLOT software program (© Thomas Williams and Colin Kelley, version 4.0 for Windows) through the Levenberg–Marquardt algorithm.Citation22,Citation24
Results and discussion
PGA functionalization and percentage coating
The FTIR spectrum of bare-SPIONs revealed a sharp peak at 578.8 cm−1, which is typical of the Fe-O bond in iron oxide nanoparticles (). However, three distinctive peaks were shown for pure PGA corresponding to C=O stretch of free carboxylic acids at 1633.4 cm−1, asymmetric COO− stretch and peak overlap of N-H/C-N deformation at 1578.2 cm−1, and symmetric COO− at 1400.9 cm−1.Citation26 After coating of PGA on SPIONs, peaks of both bare-SPIONs and pure PGA appeared in the spectrum of PGA-SPIONs, affirming the coating of PGA did occur on the surface of the SPIONs ().
Figure 1 Comparative Fourier transform infrared spectra of bare-SPIONs. (A–a) Pure PGA (A–a′) and PGA-SPIONs (A–b), as well as thermogravimetric curves of bare-SPIONs (B–a) and PGA-SPIONs (B–b).
Abbreviations: SPIONs, superparamagnetic iron oxide nanoparticles; PGA, poly(γ-glutamic acid); PGA-SPIONs, poly(γ-glutamic acid)-superparamagnetic iron oxide nanoparticles.
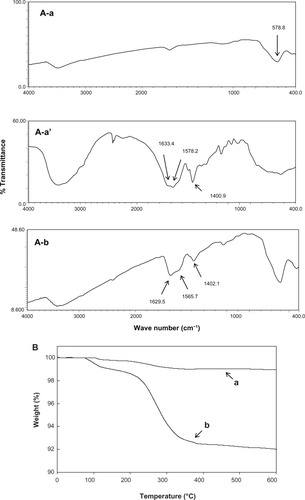
The thermogravimetric analyzer curves depicted an initial small weight loss of ∼0.5%–1% caused by the removal of adsorbed water or surface hydroxyl groups (). However, at temperatures > 200°C, the weight loss rose to 1% for bare-SPIONs and 8% for PGA-SPIONs. It is possible that the decomposition of amorphous iron hydroxides and the formation of iron oxide for bare-SPIONs and the evaporation followed by the decomposition of PGA coating for the PGA-SPIONs led to these results. Based on the relative comparison of weight loss curves of bare- and PGA-SPIONs, the amount of PGA coated on SPIONs was estimated to be 7% ().
Structure and surface morphology
The XRD pattern showed six characteristic diffraction peaks corresponding to the Bragg reflections (2 2 0) at 30°, (3 1 1) at 35°, (4 0 0) at 43°, (4 2 2) at 53°, (5 1 1) at 57°, and (4 4 0) at 62°, implying that the iron oxide spinel structure is typical of either magnetite (Fe3O4) or maghemite (γ-Fe2O3)Citation28 (). Nonetheless, the estimated lattice constant for bare-SPIONs (8.3945 Å) and PGA-SPIONs (8.3925 Å) was close to the standard value for magnetite (8.3960 Å) and not maghemite (8.3515 Å), demonstrating that the synthesized SPIONs are mainly magnetite. A 1 × 105 magnified TEM image showed the morphology of both bare-SPIONs and PGA-SPIONs to be roughly spherical in shape, with the mean particle size determined by the particle size distribution histograms, being 8.5 and 11.7 nm, respectively ().
Magnetic property
The magnetization curves obtained from vibrating sample magnetometer data at varying applied magnetic fields (H, Oe) illustrated a superparamagnetic behavior with zero coercivity or remanence, indicating that a high dispersibility at H=0 and a high attractive force at H≠0 may occur (). This phenomenon is particularly important for fast separation of metal-loaded PGA-SPIONs within a short time, and the driving of metals to specific organs or tissues by using a magnet. Based on the linear plot of magnetization moment versus 1/H, the saturation magnetization (Ms) values were obtained by extrapolating the straight-line to zero magnetic field, and were 70.3 emu/g for bare-SPIONs and 61.5 emu/g for PGA-SPIONs (). However, the Ms value reported for bulk magnetite is relatively higher (89–92 emu/g),Citation28 which may be attributed to the small-particle surface effect resulting from the large surface area to volume ratio of synthesized SPIONs.Citation28,Citation29 A drop in Ms value by 12.5% upon the coating of SPIONs with PGA confirmed the PGA coating on SPIONs. Nevertheless, an abundant magnetic property was still retained for PGA-SPIONs to provide fast separation using a magnet.
Figure 3 Magnetization curves showing magnetic moments as a function of applied magnetic field (A), and linear plots depicting the variation in magnetic moments as affected by reciprocal of applied magnetic field (B). (a) Bare-SPIONs; (b) PGA-SPIONs.
Abbreviations: SPIONs, superparamagnetic iron oxide nanoparticles; PGA-SPIONs, poly(γ-glutamic acid)-superparamagnetic iron oxide nanoparticles.

Zeta potential, surface charge, and point of zero charge
The zeta potentials measured in millivolts (mV) as a function of different pH (2–8) revealed positive surface charge for bare-SPIONs at most pH values (2–6), except for pH 7 and 8 (). Conversely, upon coating with PGA, an opposite trend was observed with the surface charge being negative at pH 3–8 and positive at pH 2. The cross-over point from positive to negative zeta potential denoting the pH of point of zero charge (pHPZC) was 6.8 for bare-SPIONs, which was similar to the reported value of 6.5.Citation17 However, after the coating of PGA, the pH value declined to 2.4 amid the presence of abundant carboxylic acid groups. The low pHPZC of PGA-SPIONs indicated that their negative surface charges at most of the environmental pH (3–9) and biological pH (1–8) should prevent aggregation and facilitate the removal of positively-charged metal ions. A similar pHPZC value of ∼2.3 was reported for carboxylic acid functionalized humic acid-SPIONs.Citation14
Metal removal at different pH levels
A potential oral therapeutic drug is subjected to a range of pH conditions when administered into the human body, as gastrointestinal pH varies widely from highly acidic to slightly basic (pH 1–8) due to the presence of complex mixtures of different minerals and secretions.Citation23,Citation24 Additionally, the varying pH levels can affect the dissociation ability and structural conformation of polymer-coating material as well as metal speciation and stability of metal complex in a solution.Citation30 Removal of lead or cadmium by PGA-SPIONs was poor at pH 1–2.5 for the former and at pH 1–3 for the latter. However, significant metal removal occurred afterwards reaching a plateau in the pH range from 4–8 ().
Figure 5 Effect of solution pH on lead and cadmium removal by PGA-SPIONs.
Abbreviation: PGA-SPIONs, poly(γ-glutamic acid)-superparamagnetic iron oxide nanoparticles.
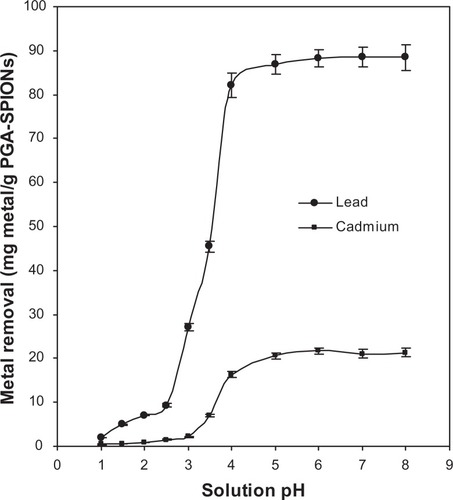
From a physiological point of view, an efficient removal of metals could be attained under the gastrointestinal pH 5.5–7 in the large intestine, 5.5 in the cecum/ascending colon, 6–6.5 in the duodenum, 6.8 in the distal colon, 7 in the small intestine, and 7–8 in the jejunum and ileum, but the metal removal efficiency remained inadequate at a stomach pH of 1–2.5. Nevertheless, the small intestine is the main organ from where minerals and toxic compounds are absorbed. More importantly, the removal capacity of PGA-SPIONs towards lead or cadmium increased over a pH range of 1–4 or remained stable at 4–8, revealing that the bound metals were unleached under the gastrointestinal condition.
Following a rise in pH from 2.5 to 5, both the lead and cadmium removal by PGA-SPIONs reached 86.91 and 20.54 mg/g, respectively (). This variation in removal capacity may be due to differences in electronegativity between lead (2.33) and cadmium (1.69). It is also possible that the high affinity of several labile hydrolysis and polymeric species formed by lead at high concentrations (such as [Pb4(OH)4]4+, [PB3(OH)5]+, Pb(OH)+, and Pb(OH)2) may have resulted in a higher removal capacity than by simple ion exchange rules.Citation24 As the coating material PGA contains numerous carboxyl groups, the solution pH and pHZPC of PGA-SPIONs should strongly influence the metal removal capacity. Consequently, the carboxyl groups on PGA- SPIONs remained unionized at pH < pHZPC of PGA-SPIONs (pH 2.4, ). However, a pronounced metal removal observed at pH > 2.5 for lead and pH > 3 for cadmium was probably caused by ionization of the carboxyl groups. In addition, the amide groups on PGA-SPIONs may also be responsible for metal removal and could be explained based on the Pearson’s hard soft acid base (HSAB) principle.Citation31 The HSAB principle depicts that a hard acid prefers to bind with a hard base through ionic interactions, while a soft acid binds with a soft base through covalent interactions. Given the classification of lead as a borderline acid and cadmium as a soft acid, their interaction with a hard base like carboxylate anions on PGA may not be possible unless through contribution from some other functional groups like amides, as pointed out by Siao et al.Citation24
Metal removal kinetics
Rapid removal kinetics is therapeutically and diagnostically advantageous given that fast uptake could reduce both the residence time of metal and the amount absorbed in human body.Citation9,Citation24 Binding kinetics of lead or cadmium by PGA-SPIONs as shown in revealed a 92%–95% metal uptake within 1 minute and reached a peak within 5 minutes. Such rapid kinetic behavior of PGA-SPIONs suggests the absence of internal diffusion resistance during mass transfer, resulting in faster surface attachment, which is a critical factor for the treatment of acute metal intoxication. Similar rapid kinetics of 99% lead removal was shown within 1 minute by dimercaptosuccinic acid-SPIONs,Citation15 and 99% cadmium removal within 3 minutes by thiol-self-assembled monolayers on mesoporous silica.Citation9 However, a relatively slower rate of 100% removal within ∼15 minutes was reported for lead, cadmium, mercury, and copper by humic acid-SPIONs,Citation14 which was attributed to the slow site–site exchange of heavy metals in a disordered structure of the humic acid layer. To derive the removal rate and capacity, the kinetic data were fitted with two commonly used pseudo-first-order and pseudo-second-order models, whose nonlinear forms are represented as given below:Citation22,Citation24
Metal removal isotherms
The development of removal isotherms is pivotal for elucidating the interactions between heavy metals and PGA-SPIONs, as are the removal mechanism and removal affinity.Citation22,Citation24 More importantly, the modeling of equilibrium data provides the maximum removal capacity value for comparison with a wide variety of reported adsorbents. As the optimum pH for maximum lead or cadmium removal ranged from 4–8, the removal isotherms were developed at pH 5.5 in order to be representative for most regions of the gastrointestinal environment (5.5–8), with the exception of the stomach (1–2.5). depicts the isotherm data obtained for the removal of lead or cadmium by PGA-SPIONs over a range of metal concentration varying from 10–100 mg/L. Following an increase in metal concentration from 10–100 mg/L, the amount of metal removed by PGA-SPIONs rose from 37.33–93.12 mg/g for lead and 7.14–24.35 mg/g for cadmium. By comparing these data with the classification of several isotherm shapes discussed by Giles et al,Citation32 the shape of the removal isotherm for lead or cadmium by PGA-SPIONs was designated as L-type, implying that the metal removal mechanism mainly involved chemical forces instead of physical interactions. Such L-type isotherms are actually Langmuir-type isotherms, signifying a high degree of removal at a low metal concentration.Citation22
Two popular isotherms, namely, the FreundlichCitation33 and the Langmuir,Citation34 were used for modeling the isotherm data and establishing an appropriate correlation with minimum deviation. The non-linear representation of the isotherm models is shown below:Citation22,Citation24
Metal removal at different ionic strengths
It has been reported that some of the ion-exchange resins currently used as oral drugs for the removal of toxins suffer from swelling and shrinking due to variation in ionic strength, thereby reducing the therapeutic potential of such resin-based drugs.Citation9 For instance, a cation-exchange resin sodium poly(styrenesulfonate) and cross-linked allylamine hydrochloride used for binding excess potassium and phosphate in the gastrointestinal tract were shown to impede their detoxification efficiency.Citation9 Moreover, the ionic strengths in biological matrices (blood and urine) are likely to be greater than in most natural waters (river and ground water). Consequently, the ability of PGA-SPIONs to remove lead or cadmium was evaluated in the presence of varying ionic strengths (0.001–1 M) prepared by different concentrations of sodium acetate (). Compared to control without sodium sulfate (87.7 mg/g), the removal capacity of lead by PGA-SPIONs remained unaltered in the 0.001 M sodium acetate solution (88.1 mg/g), but soared to 136.3, 132.9, and 110.1 mg/g in the 0.01, 0.1, and 1 M solution, respectively, probably due to extra mobility gained by lead through competition with sodium ions. In contrast, for cadmium removal, the ionic strength varied from 0–0.01 M sodium acetate, and did not show any impact on the removal capacity (20.3–21.5 mg/g). However, a drastic decline occurred afterwards, as evident by a drop in removal capacity to 2.3 and 0 mg/g in 0.1 and 1 M sodium acetate solution, respectively (). Thus, the varying ionic strengths encountered in biological matrices may be unable to prevent the lead removal by PGA-SPIONs. Instead, the removal capacity was greatly enhanced.
Lead or cadmium removal as affected by essential metals
One of the major drawbacks of detoxification therapy is the ability of chelators to bind with some other essential metals like calcium and zinc, facilitating urinary excretion and ultimately causing deficiency in essential minerals. Take EDTA as an example; it can be used in chelation therapy, but it was found that calcium levels can exhibit a 2-fold reduction, while zinc levels can show an 18-fold reduction.Citation10 Though this effect could be partly remedied by appropriate supplementation of essential minerals during detoxification therapy, the detoxification efficiency of chelating agents on target toxic metals may be reduced.Citation24 Therefore, the effect of six essential metals including copper, zinc, iron, magnesium, calcium, and potassium on lead or cadmium removal by PGA-SPIONs was examined in a bisolute 1:1 mixture of lead/cadmium:essential metal ().
Figure 8 Effect of different essential metals on lead removal (A) and cadmium removal (B) by PGA-SPIONs.
Abbreviation: PGA-SPIONs, poly(γ-glutamic acid)-superparamagnetic iron oxide nanoparticles.
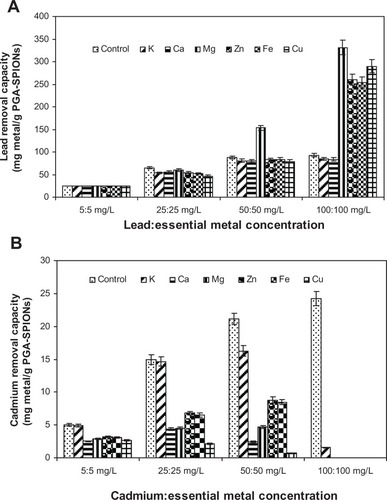
Lead removal by PGA-SPIONs in the presence of four different levels (5, 25, 50, and 100 mg/L) in a 1:1 mixture of lead/cadmium:essential metals showed no interference at 5 mg/L (). However, upon raising the concentration to 25 mg/L, the lead removal dropped by 28.4% for copper, 20.2% for iron, 17.4% for potassium, 16.6% for zinc, 14.3% for calcium, and 8.2% for magnesium. Interestingly, at 50 mg/L, the decrease in lead removal was only 9.8% for copper, 8.9% for calcium, 8.5% for potassium, 5.8% for zinc, and 5.3% for iron, but with a sharp rise (∼2-fold) for magnesium when compared to the control without essential metals (). Likewise, the lead removal capacity climbed to a peak (∼3-fold) in the presence of magnesium, zinc, iron, and copper at 100 mg/L, but no significant difference was noted for calcium and potassium between 50 and 100 mg/L. It may be postulated that the competition between essential metals and lead ions could enhance the mobility of lead ions for additional lead binding onto unoccupied sites of PGA-SPIONs. Unlike lead removal, the cadmium removal by PGA-SPIONs dropped sharply in the presence of essential metal ions, with the degree of interference following the following order: copper > calcium > magnesium > iron > zinc > potassium (). Being a monovalent ion, potassium showed a slight interference (2.0%–2.6%) in cadmium binding at 5 and 25 mg/L; however, this effect became more pronounced at 50 mg/L (23.1%) and 100 mg/L (93.6%). On the contrary, at 5, 25, and 50 mg/L, the divalent ions reduced the cadmium binding to a larger extent, which amounted to 47.7, 85.7, and 96.6% for copper; 50.3, 70.6, and 88.4% for calcium; 42.0, 69.7, and 77.8% for magnesium; 38.4, 56.5, and 60.0% for iron; and 36.6, 54.6, and 58.4% for zinc, respectively ().
Comparatively, PGA-SPIONs were more selective towards lead removal than cadmium, which may be explained based on the HSAB principleCitation31 as described in the preceding section. Since cadmium is a soft acid, its interaction with a hard base like the carboxyl groups in PGA-SPIONs is unfavorable in the presence of hard acids like calcium, magnesium, and potassium as well as borderline acids like iron and zinc. This should also explain why iron and zinc were the least interfering essential metals noted in this study. Furthermore, for lead removal, an interference of only ≤20% was shown by all the essential metals except for copper (28.4%). As pointed out previously, the high selectivity of PGA-SPIONs towards lead can be attributed to the fact that lead is a borderline acid and a highly electronegative ion (2.33). Also, lead tends to form several hydrolyzed and polymeric species, all of which are more prone to binding with PGA-SPIONs.
Metal removal from SGIF
To learn about the impact of the gastrointestinal environment on metal removal by PGA-SPIONs, the effect of pH level and isotherm studies were carried out with SGIF prepared following the procedure described previously. depicts the influence of pH on the removal of lead or cadmium by PGA-SPIONs from both SGIF and deionized water for comparison. Obviously, the pH profile for SGIF followed a similar trend as the deionized water, with the lead removal capacity being higher at all pH levels for the former, which should be beneficial for in vivo application. Nevertheless, the lead removal capacity attained a plateau between pH 5–8 for SGIF and pH 4–8 for deionized water. Conversely, the cadmium removal capacity for SGIF showed a slightly lower value than that of the deionized water at all pH levels, which should be caused by sodium ions competing more effectively with cadmium for the active sites on the PGA-SPIONs (). Upon raising the pH level from 1 to 8, the removal capacity of lead and cadmium from the SGIF climbed to 138.59 and 16.02 mg/g, respectively, which was 56.4% higher and 25.0% lower than that for deionized water.
Figure 9 Solution pH variation (A) and isotherm (B) studies for removal of lead and cadmium by PGA-SPIONs from simulated gastrointestinal fluid.
Abbreviation: PGA-SPIONs, poly(γ-glutamic acid)-superparamagnetic iron oxide nanoparticles.

The high efficiency in lead removal by PGA-SPIONs across all pH levels for SGIF when compared to deionized water may be accounted for by the additional mobility gained by lead ions through the competition of sodium ions in SGIF. It is worth pointing out that sodium chloride (0.03 M) was used as one of the ingredients in the preparation of SGIF. This tendency is further substantiated by a 55% enhancement in lead removal capacity in the presence 0.01 M sodium acetate when compared to the control (without sodium acetate), as observed in the preceding study on effect of ionic strength. However, this effect was not observed for cadmium removal by PGA-SPIONs, which may be explained based on the HSAB theory.Citation31 Accordingly, the sodium ions (a hard acid) should more readily bind with a hard base like the carboxyl groups on PGA-SPIONs when compared to cadmium (a soft acid), thereby preventing binding of cadmium on PGA-SPIONs. Based on this phenomenon, one would expect a similar trend to occur for lead removal as well, as lead is only a borderline acid. However, the high lead removal capacity by PGA-SPIONs in the presence of sodium ions may be attributed to its high electronegativity (2.33) as compared to sodium ions (0.93). Though cadmium possesses a higher electronegativity (1.69) than sodium, its lower binding on PGA-SPIONs should be due to strong acid-base interaction between sodium and carboxyl groups on PGA-SPIONs.
As the pH study showed different removal capacities for SGIF when compared to deionized water, the removal isotherms were developed for a range of metal concentrations (10–100 mg/L) in SGIF at pH 5.5 and modeled with the Langmuir model (Eq 4) (). Metal removal isotherms obtained in SGIF are shown in along with those obtained in deionized water for comparison. Modeling of isotherm data with the Langmuir model provided a more precise fit than the Freundlich model, with the r2 for the former being 0.9993 and 0.9967 for lead and cadmium, respectively (Freundlich model not shown) (). Based on the fitted Langmuir parameters, the maximum removal capacity (qm) and equilibrium constant (Ke) obtained were 147.71 mg/g and 0.483 L/mg for lead and 23.15 mg/g and 0.041 L/mg for cadmium, respectively. Apparently, the isotherms for lead removal by PGA-SPIONs that developed for SGIF displayed a 1.5-fold increment in the qm value compared to that for deionized water, while a 0.3-fold drop in qm value was observed for cadmium.
Conclusion
The present study demonstrated the potential of poly(γ-glutamic acid)-coated superparamagnetic nanoparticles in the removal of heavy metals from a metal solution and simulated gastrointestinal fluid. Positively-charged PGA-SPIONs belonging to a magnetite structure were synthesized with a particle size of 11.7 nm and a magnetization value of 61.5 emu/g. Efficient removal of heavy metals was shown at most of the gastrointestinal pH conditions, as illustrated with rapid removal kinetics (1–5 min) and good removal capacity (147.71 mg/g for lead and 23.15 mg/g for cadmium). Unlike cadmium, different ionic strengths and essential metals showed no impact on lead binding by PGA-SPIONs, which is considered to be therapeutically beneficial for the selective removal of lead from the biological matrix, and thus can be applied for clinical treatment of metal intoxication.
Acknowledgements
The authors wish to thank the Electron Microscope Laboratory of Prof Tzong Jwo Jang at the School of Medicine, Fu Jen Catholic University, Taipei, Taiwan for technical assistance.
Disclosure
The authors report no conflicts of interest in this work.
References
- TandonSKSinghSRole of vitamins in treatment of lead intoxicationThe Journal of Trace Elements in Experimental Medicine2000133305315
- BarbierOJacquilletGTaucMCougnonMPoujeolPEffect of heavy metals on, and handling by, the kidneyNephron Physiol2005994105110
- SabolićICommon mechanisms in nephropathy induced by toxic metalsNephron Physiol20061043107114
- Damek-PoprawaMSawicka-KapustaKHistopathological changes in the liver, kidneys, and testes of bank voles environmentally exposed to heavy metal emissions from the steelworks and zinc smelter in PolandEnviron Res2004961727815261786
- NeriLCHewittDAluminum, Alzheimer’s disease, and drinking waterLancet199133887633901677733
- MikirovaNCasciariJHunninghakeRRiordanNEDTA chelation therapy in the treatment of toxic metals exposureSpatula DD2011128189
- WangCFangYPengSMaDZhaoJSynthesis of novel chelating agents and their effect on cadmium decorporationChem Res Toxicol199912433133410207121
- FloraSJMittalMMehtaAHeavy metal induced oxidative stress and its possible reversal by chelation therapyIndian J Med Res2008128450152319106443
- YantaseeWRutledgeRDChouyyokWFunctionalized nanoporous silica for the removal of heavy metals from biological systems: adsorption and applicationASC Appl Mater Interfaces201021027492758
- WatersRSBrydenNAPattersonKYVeillonCCAndersonRAEDTA chelation effects on urinary losses of cadmium, calcium, chromium, cobalt, lead, magnesium, and zincBiol Trace Elem Res200183220722111794513
- BrownMJWillsTOmaluBLeikerRDeaths resulting from hypocalcemia after administration of edetate disodium: 2003–2005Pediatrics20061182e534e53616882789
- NawirskaABinding of heavy metals to pomace fibersFood Chem2005903395400
- ZhangNHuangCOuSIn vitro binding capacities of three dietary fibers and their mixture for four toxic elements, cholesterol, and bile acidJ Hazard Mater2011186123623921095057
- LiuJFZhaoZSJiangGBCoating Fe3O4 magnetic nanoparticles with humic acid for high efficient removal of heavy metals in waterEnviron Sci Technol200842186949695418853814
- YantaseeWWarnerCLSangvanichTRemoval of heavy metals from aqueous systems with thiol functionalized superparamagnetic nanoparticlesEnviron Sci Technol200741145114511917711232
- GuptaAKGuptaMSynthesis and surface engineering of iron oxide nanoparticles for biomedical applicationsBiomaterials200526183995402115626447
- ChangYCChenDHPreparation and adsorption properties of monodisperse chitosan-bound Fe3O4 magnetic nanoparticles for removal of Cu(II) ionsJ Colloid Interface Sci2005283244645115721917
- YavuzCTMayoJTYuWWLow-field magnetic separation of monodisperse Fe3O4 nanocrystalsScience2006314580196496717095696
- SongJKongHJangJAdsorption of heavy metal ions from aqueous solution by polyrhodanine-encapsulated magnetic nanoparticlesJ Colloid Interface Sci2011359550551121543080
- HoGHHoTIHsiehKHγ-polyglutamic acid produced by Bacillus subtilis (natto): structural characteristics, chemical properties, and biological functionalitiesJournal of the Chinese Chemical Society20065313631384
- ShihILVanYTThe production of poly-(γ-glutamic acid) from microorganisms and its various applicationBioresour Technol200179320722511499575
- InbarajBSChienJTHoGHYangJChenBHEquilibrium and kinetic studies on sorption of basic dyes by a natural biopolymer poly(γ-glutamic acid)Biochem Eng J2006313204215
- InbarajBSChiuCPChiuYTHoGHYangJChenBHEffect of pH on binding of mutagenic heterocyclic amines by the natural biopolymer poly(γ-glutamic acid)J Agric Food Chem200654176452645916910744
- SiaoFYLuJFWangJSInbarajBSChenBHIn vitro binding of heavy metals by an edible biopolymer poly(γ-glutamic acid)J Agric Food Chem200957277778419128012
- WangTLKaoTHInbarajBSSuYTChenBHInhibition effect of poly(γ-glutamic acid) on lead-induced toxicity in miceJ Agric Food Chem201058231256212567
- InbarajBSKaoTHTsaiTYChiuCPKumarRChenBHThe synthesis and characterization of poly(γ-glutamic acid)-coated magnetite nanoparticles and their effects on antibacterial activity and cytotoxicityNanotechnology201122707510121233545
- The United States Pharmacopeia and National Formulary26th edRockville, MDUnited States Pharmacopeial Convention Inc.2003
- InbarajBSTsaiTYChenBHSynthesis, characterization and antibacterial activity of superparamagnetic nanoparticles modified with glycol chitosanScience and Technology of Advanced Materials201213015002
- ShanZYangWSZhangXHuangQMYeHPreparation and characterization of carboxyl-group functionalized superparamagnetic nanoparticles and the potential for bio-applicationsJ Braz Chem Soc200718713291335
- HeLMNeuMPVanderbergLABacillus licheniformis γ-glutamyl exopolymer: physiochemical characterization and U(VI) interactionEnviron Sci Technol200034916941701
- PearsonRGHard and soft acids and basesJ Am Chem Soc1963852235333539
- GilesCHMacEwanTHNakhwaSNSmithDStudies in adsorption. Part XI. A system of classification of solution adsorption isotherms, and its use in diagnosis of adsorption mechanisms and in measurement of specific surface areas of solidsJ Chem Soc196011139733993
- FreundlichHMFÜber die adsorption in lösungen (Adsorption in solution)Zeitschrift fur Physikalische Chemi190657384470
- LangmuirIThe adsorption of gases on plane surfaces of glass, mica and platinumJ Am Chem Soc191840913611403
- OuSGaoKLiYAn in vitro study of wheat bran binding capacity for Hg, Cd, and PbJ Agric Food Chem199947114714471710552878
- SergushchenkoISKovalevVVBednyakVEKhotimchenkoYSA comparative evaluation of the metal-binding activity of low-esterified pectin from the seagrass Zostera marina and other sorbentsRussian Journal of Marine Biology20043017072