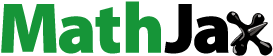
Abstract
CB13 (1-Naphthalenyl[4-(pentyloxy)-1-naphthalenyl]methanone)-loaded poly(lactic-co-glycolic acid) nanoparticles (NPs) were produced by nanoprecipitation and tested for their in vitro release behavior and in vitro cytotoxicity assays. The effects of several formulation parameters such as polymer type, surfactant concentration, and initial drug amount were studied. NPs had a particle size 90–300 nm in diameter. Results obtained show that the main influence on particle size was the type of polymer employed during the particle production: the greater the hydrophobicity, the smaller the particle size. In terms of encapsulation efficiency (%), high values were achieved (∼68%–90%) for all formulations prepared due to the poor solubility of CB13 in the external aqueous phase. Moreover, an inverse relationship between release rate and NP size was found. On the other hand, low molecular weight and low lactide content resulted in a less hydrophobic polymer with increased rates of water absorption, hydrolysis, and erosion. NPs showed no cytotoxicity and may be considered to be appropriate for drug-delivery purposes.
Keywords:
Introduction
Nanotechnology has become one of the most intensively studied research areas in recent times. Materials at the scale of a nanometer have unique physicochemical properties that are due to their small size, surface area, chemical composition, surface structure, solubility, and shape.
Over the last several decades, numerous nanocarrier platforms have been studied for their use as therapeutic agents, with great enthusiasm, in both academic and industrial applications. These nanocarrier platforms include liposomes, polymer therapeutic conjugates, polymeric micelles, dendrimers, nanoshells, and nucleic acid-based nanoparticles (NPs). These polymeric nanocarriers bear great potential for biomedical applications owing to their biomolecular design and small size, and are known to have shown exciting results in preclinical studies demonstrating their potential as therapeutic carriers.Citation1 Among the polymeric carriers, poly(lactic-co-glycolic acid) (PLGA) has a great potential in applications combining targeting, imaging, diagnostics, and therapy.Citation2 Furthermore, as is known, this polymer can easily be hydrolyzed into individual monomers (lactic acid or glycolic acid), which are then removed from the body via normal metabolic pathways.Citation3
However, low water solubility of these drugs limits their oral bioavailability and absorption. There is thus an urgent need for adequate options to deliver these drugs to the patient. Although there are diverse strategies (use of cosolvents, salt formation, complexes with cyclodextrins, etc) to solve this problem,Citation4 several nanotechnology-based drug delivery systems have emerged to increase the bioavailability of numerous drugs that are poorly soluble in water.Citation5,Citation6 Currently, there are a considerable number of nanobased drug-delivery systems being developed by various pharmaceutical companies. A comprehensive review of strategies to improve the oral bioavailability of this type of drugs can be found in Fasinu et al.Citation7
There have been controversial indications as to the extent and mechanism of transport of these nanocarriers, but there is now no dispute over the fact that particulate uptake does take place, especially via the Peyer’s patches M-cells and isolated follicles in gut-associated lymphoid tissue, and also via the normal enterocytes.Citation8
In this paper, the cannabinoid 1-Naphthalenyl [4-(pentyloxy)-1-naphthalenyl]methanone (CB13), which acts as a potent agonist at both the CB1 and CB2 receptors, is used as a model drug. This compound, in behavioral animal models (rat and guinea pig) of chronic pain (neuropathic and nociceptive), has been shown to reverse established mechanical hyperalgesia after both oral administration and local injection into a hind-paw. In behavioral tests for central nervous system (CNS) activity in rat, CB13 produced significant CNS effects only at doses that were 20-fold higher than the oral doses required to reverse hyperalgesia. Thus, these data indicate that CB13 produces antihyperalgesic activity predominantly via an action on peripheral sensory nerves.Citation9
However, CB13 is highly lipophilic and belongs to the class 2 compounds (low solubility and a high permeability) of the Biopharmaceutics Classification System, showing a low water solubility (∼0.001–0.002 mg/mL).Citation9 As a consequence of its poor solubility and dissolution in the gastrointestinal fluids, this compound is incompletely absorbed.Citation9 Hence, this drug was selected on the basis of its therapeutic interest and inadequate physicochemical properties. It is expected that, by including CB13 in a PLGA nanocarrier, problems associated with low water solubility will disappear. This way, CB13 oral viability would be improved.
Hydrophobic drugs are usually encapsulated in biodegradable polymers using one of three methods: homogenization, sonication, or nanoprecipitation. An ideal method would produce NPs with the following characteristics: narrow size distribution; size in the range 100–1000 nm (with unimodal population); capacity for high drug incorporation; controlled drug content over a wide range; nontoxic; and relatively easily produced.Citation10 The selected preparation method of NPs was the nanoprecipitation method, also known as solvent displacement method. It is based on interfacial deposition of a polymer after displacement of a semipolar solvent, miscible with water, from a lipophilic solution. Rapid diffusion of the solvent into the aqueous phase results in a decrease in the interfacial tension between the two phases, which increases the surface area and leads to the formation of small droplets of organic solvent, even without any mechanical stirring.Citation11 Moreover, this method provides high encapsulation efficiency (EE) for drugs presenting low water solubility.Citation3
So, the main objectives of the present study were: (1) to produce CB13-PLGA loaded NPs using nanoprecipitation as the most suitable technique of synthesis; (2) to realize the in vitro characterization of the NPs; and (3) to determine the in vitro cytotoxicity of NPs and evaluate their safety and potential use as nanocarriers for oral drug administration.
Methods
Materials
CB13 was obtained from Tocris Cookson Ltd (Bristol, UK). PLGA 50:50 was obtained in different formulations, Resomer® RG 502 (molecular weight [Mw]: 12,000; inherent viscosity: 0.24 dL/g), Resomer RG 502H (Mw:12,000; inherent viscosity: 0.19 dL/g), Resomer RG 504 (Mw:48,000; inherent viscosity: 0.5 dL/g), Resomer RG 504H (Mw: 48,000; inherent viscosity: 0.53 dL/g) and PLGA 75:25, and Resomer RG 752S (Mw: 15.000; inherent viscosity: 0.24 dL/g), from Boehringer Ingelheim Gmbh (Ingelheim, Germany).
The surfactants employed, Span® 60 and Pluronic® F-68, were obtained from Sigma-Aldrich (St Louis, MO). Acetone PRS was purchased from Panreac Química (Barcelona, Spain); propylene glycol (PPG) and glycerol were obtained from Acofarma Distribución SA (Barcelona, Spain).
The reversed phase high-performance liquid chromatography (RP-HPLC) analysis was carried out on a Hitachi LaChrom® Series HPLC system (D-7000; Hitachi Ltd, Tokyo, Japan) equipped with an L-7200 automatic injector, an interphase D-7000 and a quaternary pump (model L-7100), and DAD UV–VIS detector (model L-7455). A Waters Corp Spherisorb ODS2 column (10 μm, 4.6 mm × 250 mm; Milford, MA), kept at 40.0°C ± 0.1°C (Elite LaChrom L-2350 column oven; Hitachi Ltd), was used in this analysis. Data collection and calculation were done by using HSM D-7000 LaChrom® software (Hitachi, Ltd).
Preparation of CB13-loaded PLGA NPs
The NPs were prepared by the nanoprecipitation method with modification.Citation11 Briefly, a weighed amount of PLGA was codissolved with Span 60 in acetone to reach a 1.5% w/v concentration. Then, 5 mL of such solution was added dropwise at different rates (30, 15, and 5 mL/min) using a syringe pump (Harvard Apparatus Ltd, Edenbridge, UK) into 15 mL Pluronic F68 aqueous solution (0.5% w/v) under magnetic stirring. The acetone was then evaporated at room temperature (RT) for 4 hours.
The particle suspension was centrifuged over a glycerol bed (100 μL) at 10,000 rpm for 15 minutes at 4°C to collect the NPs. After washing twice, the NPs were resuspended in the cryoprotectant solution and then frozen in liquid nitrogen and lyophilized (Criodos-50; Telstar Industrial SL, Terrassa, Spain), at −80.0°C ± 0.5°C, and 0.057 mbar, to obtain a fine powder. All the formulations were prepared in triplicate (n = 3).
For CB13-PLGA loaded NPs, the polymer and drug were codissolved in acetone at different concentrations (6, 10, and 20% w/w).
Characterization methods
The mean diameter and size distribution of CB13 loaded-PLGA NPs were measured at 25.0°C ± 0.5°C by a laser scattering technique based on Mie theory (Partica LA-950V2; Horiba Ltd, Kyoto, Japan). An aliquot of 1 mL of recently prepared particles was diluted in a 12 mL cell. Measurements were carried out under continuous magnetic agitation. The influences of (1) organic phase addition rate into the aqueous phase, (2) the type of polymer, and (3) drug loading were studied.
NP aspect and morphology were studied by scanning and transmission electron microscopy (SEM and TEM). The shape and morphology characteristics of the NPs were determined by SEM (XL-30; Royal Philips Electronics, Amsterdam, The Netherlands) after coating lyophilized samples with a gold thin film. For TEM analysis (CM-10; Philips), a sample of lyophilized particles was suspended in distilled water. An aliquot of this suspension was dropped on a grid and dried at RT.
NPs surface charge was determined by zeta potential (ZP) measurements. The ZP of the particles was determined by laser Doppler (Zetamaster 300; Malvern Instruments Ltd, Malvern, UK). ZP measurements were carried out in triplicate after washing the NPs with distilled water at RT. The effects of polymer type and drug loading were studied.
Chromatographic conditions
The introduction of new HPLC methods for routine quality control of pharmaceutical preparations starts with the establishment of the optimal measurement conditions and provides the maximum relevant information by analyzing the experimental data.Citation12–Citation14
The chromatographic conditionsCitation15,Citation16 were determined using a column C18 (Spherisorb® 5 μm ODS2, 4.6 × 250 mm Analytical Column; Waters Corp). The mobile phase consisted of two solvents:
Solvent A: acetonitrile:water:acetic acid (75:23.7:1.3 v/v)
Solvent B: acetonitrile
These solvents were maintained in a flow rate of 1.000 mL/min: 70%:30% (A:B). The mobile phase was filtered through a 0.22 μm nitrocellulose-membrane filter (Merck Millipore, Billerica, MA) and degassed under vacuum prior to use. A calibration curve was constructed using standard CB13 solutions in acetone. The calibration curve was linear between 2.5 and 750 μg/mL (y = 17,268 ·x + 19,0397, R2 = 0.9900, F = 583.78, P = 3.25 ·10−3). The limits of detection and quantification were 0.5 μg/mL and 1.25 μg/mL, respectively.
The detection wavelength UV was 230 nm and the injection volume was 10 μL. The operating temperatures were maintained at RT, but the oven was heated at 40°C to favor the mobile phase flow through the column.
Determination of Pluronic F68 residuals
The most popular stabilizer for the production of PLGA-based NPs is poly(vinyl alcohol), which is difficult to remove from the NP surface and has been reported to be potentially toxic.Citation17,Citation18 In the present work, Pluronic F68 was employed to stabilize the NPs and could be considered as a valuable alternative to poly(vinyl alcohol) and a promising FDA-approved surface active agent for clinical use.Citation19
To determine the residual amounts of Pluronic F68 on PLGA NPs, 1H-NMR (500 MHz) spectra were recorded at 303 K in CDCl3, on a Brüker Avance 500 spectrometer (Brüker AXS Inc, Madison, WI). Chemical shifts were given in ppm, using the residual protonated solvent signal as reference.
Differential scanning calorimetry (DSC)
The physical status of CB13 inside the drug-loaded PLGA NPs was investigated by DSC. Thermograms of CB13, Pluronic F68, PLGA 50:50, and NPs (6% and 20% w/w drug/polymer) were recorded on a DSC (Setaram 131; Setaram Instrumentation, Caluire, France). Samples (5 mg weighed to a precision of 0.005 mg, Model CP 225D; Sartorius AG, Goettingen, Germany) were placed in aluminum pans, and the lids were crimped using a Shimadzu crimper. Thermal behavior of the samples was investigated at a scanning rate of 10°C/minute covering a temperature range of 25°C–300°C.
Stability study of NPs
It is well known that NPs made of hydrolytic degradable polymers will degrade over time. The effects of pH and temperature have a crucial effect on long-term stability.Citation20 In order to evaluate the stability of PLGA NPs, a brief study was carried out. Samples of blank and loaded NPs were incubated in Milli-Q water, phosphate-buffered saline (PBS) (pH 7.4), and Dulbecco’s modified Eagle medium (DMEM) (Sigma-Aldrich), at 4°C and 37°C. Samples were collected after prefixed incubation periods and centrifuged for 5 minutes at 4000 rpm in order to precipitate the aggregates. Finally, the mean particle size of the remaining nonaggregated NPs was determined by laser scattering.Citation21
Freeze-drying process
Freeze-drying is a widely used process for drying and improving the stability of various pharmaceutical agents. As this process is relatively slow and expensive, it is usually only used for products with a high added value, which is the case for most types of NPs containing expensive actives, such as CB13. The resulting freeze-dried NPs should have certain desirable characteristics: the preservation of the initial characteristics of the product, short reconstitution time and an acceptable suspension, low or unmodified particle size distribution of the NPs suspensions, and unchanged activity of encapsulated drug.
There are different factors than can affect the final quality of the product: formulation, use of cryoprotectant, and the storage conditions. Freezing is the first step of freeze-drying and generates many stresses. During this step, the liquid suspension is cooled, and ice crystals of pure water form – the crystallization of ice may exercise a mechanical stress on NPs, leading to their destabilization.
In the present work, the use of two commonly employed cryoprotectants at different concentrations was evaluated: propylene glycol, a low molecular weight alcohol; and mannitol, a sugar. Both were added directly to the NPs suspension before freezing with liquid nitrogen. After this, NPs were defrosted at RT. To evaluate the effect of each cryoprotectant, NP diameters were measured by laser scattering.
Determination of drug incorporation
CB13 content of the NPs was assessed directly from the extraction of the drug from NPs. The lyophilized NPs (about 5 mg) were accurately weighed using a high-precision analytical balance (d = 0.01 mg; Model CP 225D; Sartorius). Then, 1 mL acetonitrile was added, and the mixture was accurately vortexed to dissolve the particles in the organic phase. After this, 10 μL of the solution was filtered (Millex® GV syringe filter, 0.22 μm; Merck Millipore) and injected into the HPLC system for CB13 detection.
The drug content was expressed as EE (%) and drug loading (%) following EquationEquations 1(1) and Equation2
(2) :
In vitro drug release
NP samples were suspended in phosphate buffer (pH 7.4), maintained at 37°C, and stirred mechanically (100 rpm) during the release experiments (Unitronic Vaivén; Selecta S.A., Barcelona, Spain). Aliquots (500 μL) were withdrawn at fixed time intervals and filtered upon centrifugation at 8000 rpm. The filtered sample (Millex GV) (10 μL) was injected into the HPLC apparatus for the evaluation of CB13.
Cell culture and in vitro cytotoxicity
Cytotoxicity assays were realized using human normal colonic CCD-18Co cell lines and the human carcinoma T-84 cell lines obtained from American Type Culture Collection (ATCC, Manassas, VA). CCD-18Co cells were grown in Eagle’s minimal essential medium (MEM) (Sigma-Aldrich) with Earle’s balanced salt solution (BSS) and supplemented with 10% fetal bovine serum (FBS), 2 mM L-glutamine, 1 mM sodium pyruvate, and 14 mM NaHCO3. T-84 cells were grown in DMEM, supplemented with 10% FBS, 2 mM L-glutamine, 15 mM HEPES, 14 mM NaHCO3. Gentamicin (40 μg/mL) and ampicillin (500 μg/mL) (Antibióticos SA, Madrid, Spain) were used in both mediums. Cells were maintained in monolayer culture at 37°C in an atmosphere containing 5% CO2. The cytotoxicity of CB13 loaded-PLGA NPs (Resomer 502) was evaluated using a proliferation assay; a study of blank NPs and free CB13 toxicity was included. Cells were seeded in a 24-well plate (25 × 103 cells per well) and treated with CB13 loaded-PLGA NPs, blank NPs, and free CB13 at a wide range of concentrations. At 24 and 48 hours, cells were fixed with 10% trichloroacetic acid for 60 minutes at 4°C and stained with 0.4% sulforhodamine B/1% acetic acid by incubating for 10 minutes with constant shaking. Cells previously washed with 0.1% acetic acid were left in 10 mM Trizma® (T6066-100G; Sigma-Aldrich) for 15 minutes at room temperature with constant shaking. The optical density (OD) at 492 nm was determined using a Titertek Multiscan MC (Flow Laboratories Ltd., Irvine, Ayrshire, Scotland). To determine the percentage of relative cell viability (%RCV) we used the formula %RCV = (treated cells OD/untreated cells OD) × 100.
Results and Discussion
Characterization of the NPs
The size and size distribution of the NPs was measured by laser scattering and the results are reported in . The data are expressed as the mean diameter value and standard deviation (SD) obtained from independent experiments.
Table 1 Particle mean diameter, size distribution, and ZP values obtained as a function of polymer used (n = 6)
The analysis showed that the samples had a particle size 90–300 nm in diameter regardless of the initial conditions in the preparation, with a coefficient of variation (CV) about 30%. The effect of polymer type, surfactant concentration, and initial drug amount were studied. Results obtained showed that the main influence on particle size was the type of polymer employed during the particle production. It can be seen that for Resomer 502 and 502H, particles were 300 nm in diameter, and for 504, 504H, and 752S, particles were around 100 nm in diameter.
The particle size of NPs prepared with Resomers decreased with increase in molecular weight from 12,000 to 48,000 Da (502 vs 504). These results can be explained by the hydrophobicity of the polymer employed. At high molecular weight, higher hydrophobicity and smaller particle size were obtained due to longer aliphatic chains.Citation22,Citation23 No differences in size were found due to the presence of terminal carboxyl groups in both cases.Citation22 Comparing results obtained for R502 and R752S (similar molecular weight), smaller diameters were obtained when R752S was used. In this case, the lactic acid portion increased up to 75%.
The reproducibility of the procedure was also evaluated. Interlot CV% was in all cases less than 10%, which points to a reliable procedure for NP production.
The ZP values of the NPs shown in indicate the negative charges on the NP bare surface, which is due to the overall negative charges of functional groups on PLGA in Milli-Q water. The high negative surface change is an important indication for the stability of a colloidal system in medium. The repulsion among the highly negatively charged NPs provides extra stability. The ZP of four of the types of NPs was measured below −24 mV, proving the high stability of the NPs suspended in aqueous medium. When CB13-PLGA NPs were evaluated, a lower ZP value was obtained (−20 mV; data not shown).
The use (or not) of a surfactant on the organic phase also strongly affected the particle size of NPs elaborated with Resomer 502 and 502H (see ). It can be seen that when no surfactant was employed or its concentration was too low, particle diameter increased up to 600 nm, and there was a higher degree of polydispersity.
Table 2 Particle mean diameter and size distribution of the nanoparticles as a function of surfactant concentration assayed (n = 3)
The initial amount of CB13 was also evaluated. No influence on particle size or particle size distribution was found (see ).
Table 3 Size and size distribution of the nanoparticles as a function of initial amount of CB13 employed (polymer used: Resomer 502) (n = 3)
As an example, shows a SEM microphotograph of the PLGA NPs. The picture revealed a regular and symmetrical morphology, and after processing the SEM image, the size of the resulting particles was between 170 and 230 nm (by laser scattering analysis).
Figure 1 (A) Typical size distribution for CB13-PLGA NPs (polymer used: Resomer 502, loading: 10% w/w). (B) SEM photograph of the same formulation.
Abbreviations: CB13, 1-Naphthalenyl[4-(pentyloxy)-1-naphthalenyl]methanone; PLGA, poly(lactic-co-glycolic acid); NPs, nanoparticles; SEM, scanning electron microscope.
![Figure 1 (A) Typical size distribution for CB13-PLGA NPs (polymer used: Resomer 502, loading: 10% w/w). (B) SEM photograph of the same formulation.Abbreviations: CB13, 1-Naphthalenyl[4-(pentyloxy)-1-naphthalenyl]methanone; PLGA, poly(lactic-co-glycolic acid); NPs, nanoparticles; SEM, scanning electron microscope.](/cms/asset/ad5b3cfa-6003-4173-bcd6-b3a38ab9cc37/dijn_a_34633_f0001_c.jpg)
Drug loading
The capacity for drug loading of the NPs is an important factor in their formulation since high loading implies smaller amounts of the NPs are needed for a given dose of the treatment. The CB13 loading (%w/w) as well as the EE% are reported in .
Table 4 Drug loading and encapsulation efficiency of NPs (n = 6)
In terms of EE%, high values were achieved for all NPs prepared due to the poor solubility of CB13 in the external aqueous phase,Citation24 and highest values were achieved using Resomer 502. Panyam et alCitation25 demonstrated that when hydrophobic drugs are used, NPs drug loading is closely matched with the respective solid-state drug–polymer solubility, that is, the ability of the polymeric matrix to entrap drug in the dispersed state. The solid-state solubility of the drug in the polymer increased with an increase in the lactide content in the polymer and with a decrease in the molecular weight. Moreover, the presence of free acid end groups in the polymer resulted in a decrease in the drug’s solubility in the polymer. This can be explained on the basis of the increasing hydrophobicity of the polymer with increasing lactide content or with ester end groups, resulting in better solid-state solubility of the hydrophobic drug in the hydrophobic polymer matrix.
So, in our study this hypothesis is true. NPs elaborated with Resomer 502 showed the highest values in drug loading due to their lower molecular weight and the presence of the esterified end groups. On the other hand, NPs elaborated with Resomer 504H showed the lowest values due to their higher molecular weight and the presence of free acid end groups. Finally, NPs elaborated with Resomer 752S showed similar drug loading values to those obtained using Resomer 502, where the effects of molecular weight and lactide content would be balanced.
In relation to the initial amount of CB13 employed (6, 10, or 20%w/w), EE decreases when CB13 initial concentration increases. This means that there is a maximum quantity of drug that can be entrapped in the polymer matrix in the dispersed state; that is, there is a limit of drug miscibility in the polymer.Citation26 The remainder of the drug can migrate to the surrounding emulsifier aqueous phase. As was indicated by Panyam et al,Citation25 if the surfactant utilized to stabilize the emulsion is present at a concentration greater than its critical micellar concentration, surfactant micelles can solubilize the drug, resulting in a loss of encapsulation by the NPs. In our case, the concentration of surfactant-used, Pluronic F68 (0.06 mM), was slightly above its critical micellar concentration (0.04 mM), which may explain this phenomenon.
In relation to the CB13 loading (%w/w), this seems to increase with the initial amount of drug employed during the preparation of the NPs. Several studies showed that drug loading can be enhanced by increased initial amount of drug. However, EE does not necessarily increase with higher initial amount of drug. Once the maximum loading capacity of NPs is reached, further increase in initial amount of drug can even decrease the EE.Citation27
Differential scanning calorimetry (DSC)
The physical states of the drug in the NPs that could influence the in vitro and in vivo release of the drug from the system was investigated by DSC.Citation28 shows the DSC thermograms of pure CB13 powder, CB13-loaded PLGA NPs, and blank PLGA NPs. Pluronic F68 and PPG thermograms were also analyzed.
Figure 2 DSC curves of Resomer 502, free CB13, loaded-PLGA NPs (20% w/w), Pluronic® F68, PPG, and blank-PLGA NPs.
Abbreviations: DSC, differential scanning calorimetry; CB13, 1-Naphthalenyl[4-(pentyloxy)-1-naphthalenyl]methanone; PLGA, poly(lactic-co-glycolic acid); NPs, nanoparticles; PPG, propylene glycol.
![Figure 2 DSC curves of Resomer 502, free CB13, loaded-PLGA NPs (20% w/w), Pluronic® F68, PPG, and blank-PLGA NPs.Abbreviations: DSC, differential scanning calorimetry; CB13, 1-Naphthalenyl[4-(pentyloxy)-1-naphthalenyl]methanone; PLGA, poly(lactic-co-glycolic acid); NPs, nanoparticles; PPG, propylene glycol.](/cms/asset/29b04db7-57f5-4d4f-a124-67ed4e7823e6/dijn_a_34633_f0002_c.jpg)
The melting endothermic peak of pure CB13 appeared at 69.55°C (ΔH = 64,651 J/g). However, no melting peak was detected for both NP formulations. Resomer 502 and Pluronic F68 presented endothermic peaks at 45.90°C and 53.07°C, respectively. A Pluronic F68 peak was not detected for NPs. Thus, it can be concluded that CB13 inside the NPs was either in an amorphous, disordered crystalline phase or in the solid solute.Citation19,Citation29
Residual amount of Pluronic F68
There are several methods to detect residual amounts of surfactants. One of the most popular is a colorimetric method developed by Childs,Citation30 employed also to quantify residual amounts of Pluronic F68. The colorimetric method is based on the formation of a colored complex between two hydroxyl groups of Pluronic F68, Ba2+, and an iodine molecule. Then, the complex is evaluated by measuring it spectrophotometrically at 540 nm.
In the present work, the determination of the residual amounts of Pluronic F68 was carried out by 1H NMR spectroscopy.Citation31 The 1H NMR spectrum of CB13, PluronicF68, Resomer 502, and blank and loaded PLGA NPs are shown in and .
Figure 3 1H NMR spectrum of the CB13 (A), Resomer 502 (B), and Pluronic F68 (C).
Abbreviation: CB13, 1-Naphthalenyl[4-(pentyloxy)-1-naphthalenyl]methanone.
![Figure 3 1H NMR spectrum of the CB13 (A), Resomer 502 (B), and Pluronic F68 (C).Abbreviation: CB13, 1-Naphthalenyl[4-(pentyloxy)-1-naphthalenyl]methanone.](/cms/asset/95346d91-693f-45dd-bbeb-3a13fd49ae3e/dijn_a_34633_f0003_c.jpg)
![Figure 3 1H NMR spectrum of the CB13 (A), Resomer 502 (B), and Pluronic F68 (C).Abbreviation: CB13, 1-Naphthalenyl[4-(pentyloxy)-1-naphthalenyl]methanone.](/cms/asset/fb78976d-1f77-4a60-9512-c88d1d679cfe/dijn_a_34633_f0003b_c.jpg)
The cleaned NPs were collected by centrifugation and lyophilized. The powder obtained was dissolved in CDCl3 for 1H NMR analysis. The residual Pluronic F68 adsorbed onto particles was experimentally estimated according to the following equation:Citation31
Additionally, 1H-NMR spectra were analyzed to quantify the amounts of CB13 present in NPs, following EquationEquation 4(4) . Results were expressed as Q (w/w%):
These results accorded with HPLC results for CB13 loading in Resomer 502 NPs (see ).
Freeze-drying
NP integrity after the freezing process in liquid nitrogen was studied under different experimental conditions in order to determine the optimal concentration of cryoprotectant. Cryoprotectants are typically small, highly hydroxylated molecules, such as monosaccharides or low molecular weight alcohols, that can be added to the suspension prior to lyophilization to preserve the morphological integrity of a nanomaterial during freeze-drying. We therefore went on to explore an optimal concentration of two of these substances, propylene glycol and mannitol. The amounts of the cryoprotective agent were varied from 0 to 50 w/w%, by dissolving the agent directly into the NP suspension immediately prior to freeze-drying.
As can be seen in , the suspension without cryoprotectant formed sticky gels during freeze drying. These lyophilized gels could not be resuspended in Milli-Q water, PBS, nor DMEM under any of the conditions tried (including ultrasonic agitation).
Figure 5 SEM image of sticky gels without cryoprotectant.
Abbreviation: SEM, scanning electron microscopy.

The particle size after the freezing process was evaluated measuring mean particle diameter by laser scattering. shows the effect of the addition of cryoprotectant on NPs size and size distribution.
Figure 6 Effect of addition of cryoprotectants on NP size and size distribution.
Initial diameter;






Abbreviations: NP, nanoparticle; PPG, propylene glycol.

Results obtained for mannitol, suggested that the optimal concentration was not reached and that NP diameters were decreasing with increasing mannitol concentration. When propylene glycol was used, the amount of 100 μL of propylene glycol was found to be optimal (NPs diameter and CV% were close to initial particle diameter).
NP stability
For a better understanding of the in vitro release profiles, NP stability in different incubation media at 37°C was analyzed. Conversely, to determine the optimal storage conditions, stability studies at 4°C were also carried out. shows the NP size distribution at different times in Milli-Q water, PBS, and DMEM at 4°C or 37°C.
Figure 7 NP size after incubation under different conditions.
Initial particle size;


Abbreviations: NP, nanoparticle; PBS, phosphate-buffered solution.
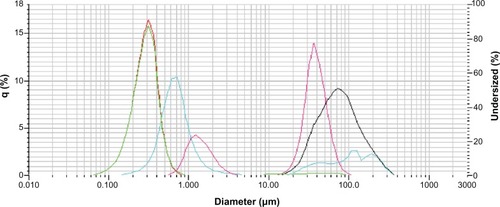
As reference, the green (and red) line indicates the initial particle size (300 ± 102 nm; t = 0 hours). After incubation in PBS at 37°C for 6 hours (black line), NP size increased to up to 100 μm in diameter. In PBS at 4°C for 6 hours (blue line), a bimodal particle size distribution was obtained. A small fraction of NPs were up to 100 μm in diameter, but the major % was around 700 nm in diameter. These results point out a slower degradation of NPs in PBS at 4°C vs 37°C. Nevertheless, comparing this result with stability in Milli-Q water at 4°C, the result is exactly the contrary. A bimodal particle size distribution was obtained, with a small fraction of NPs at around 1 μm. In DMEM at 37°C incubation, results were similar to those obtained in PBS at 37°C (data not shown).
In vitro release
The two main release mechanisms associated with drug release from PLGA-based delivery systems are diffusion and degradation/erosion. The release rate is often said to be diffusion controlled initially and degradation/erosion controlled during the final stage of the release period. However, many factors influence the rate of drug diffusion and the degradation kinetics, for example, polymer–drug interactions, drug–drug interactions, water absorption, and pore closure. An extensive review focusing on the mechanisms of drug release from PLGA-based delivery systems has been realized by Fredenberg et al.Citation32
The effect of type of polymer on the CB13 release profile from NPs is presented in . As can be seen, there was an inverse relationship between release rate and NP size. This has been explained by other authors, such as Berkland et al;Citation33 they verified that large microspheres degrade more quickly than small microspheres, probably because of an increased accumulation of the acidic products of polymer hydrolysis in large microspheres. Hydrolysis occurring on PLGA-based systems (scission of ester bonds and subsequent decrease in molecular weight) starts immediately upon contact with water. Hydrolysis creates acids, which catalyze hydrolysis. This autocatalytic process is known to cause faster degradation at the center of the PLGA matrix than at the surface. This effect becomes more pronounced with increasing dimensions of the delivery system.Citation32
Figure 8 Drug release profiles from NPs elaborated using different types of Resomer and with 20% (w/w) theoretical amount of the drug.
Abbreviation: NPs, nanoparticles.

On the other hand, low molecular weight and low lactide content result in a less hydrophobic polymer with increased rates of water absorption, hydrolysis, and erosion. Lactic acid is more hydrophobic than glycolic acid and, therefore, the PLGA copolymer becomes more hydrophobic with increase in its lactic acid content:Citation34 CB13 release was faster from Resomer 502 (PLA:polyglicolic acid [PGA] = 50:50) in comparison with Resomer 752 (PLA:PGA = 75:25).
Drug release can be obviously affected by hydrophobic interactions between the drug and the polymer. The interactions between drugs and PLGA delivery systems and their impact on release profiles have been widely discussed previously.Citation10,Citation35,Citation36 Consequently, the slowest release rate of CB13 from NPs obtained with Resomer 752 could be attributed to a favorable hydrophobic interaction between the drug and this specific copolymer. So, by increasing the polymer hydrophobicity, it is possible to reduce the initial burst and to extend the period of release.
The glass-transition temperature of the Resomer polymers decreases with decreasing lactide content. Resomer RG 502 has the weakest interactions with the drug and, therefore, this is probably the most important reason for faster drug release.Citation37,Citation38
In vitro cytotoxicity assays
Despite the advantages PLGA polymers offer as nanocarriers, significant cytotoxicity can be observed at high NP concentrationsCitation39 or when some surfactants are employed during particle synthesis.Citation40 In our study, concentrations assayed did not significantly affect cell viability, and this was unaffected by the length of exposure (24–48 hours). Moreover, the residual amounts of Pluronic F68 found on NP surfaces did not affect cell viability.
As shown in , the cytotoxic assay results indicate that there were no significant differences between the percentage of relative cell viability in the CCD-18Co and T-84 cells treated with CB13 loaded-PLGA NPs or blank NPs for 24 and 48 hours and those of the parental cells (100%). Thus, NP formulations showed no cytotoxicity and may be considered to be appropriate for drug-delivery purposes. In addition, assays with a solution of CB13 in acetonitrile at different concentrations (80, 100, and 150 μM) showed no modulation of CCD-18Co and T-84 cell proliferation (data not shown).
Figure 9 Cytotoxic effect of CB13 loaded-PLGA NPs and blank NPs on human normal colonic CCD-18Co (A), and human carcinoma T-84 (B) cell proliferation.
Notes: Cells were seeded in triplicate onto 96-well plates, treated with each compound at a wide range of concentrations, and cell viability measured by sulforhodamine method and presented as percent cell viability in relation to untreated cells (n = 3).
Abbreviations: CB13, 1-Naphthalenyl[4-(pentyloxy)-1-naphthalenyl]methanone; NPs, nanoparticles.
![Figure 9 Cytotoxic effect of CB13 loaded-PLGA NPs and blank NPs on human normal colonic CCD-18Co (A), and human carcinoma T-84 (B) cell proliferation.Notes: Cells were seeded in triplicate onto 96-well plates, treated with each compound at a wide range of concentrations, and cell viability measured by sulforhodamine method and presented as percent cell viability in relation to untreated cells (n = 3).Abbreviations: CB13, 1-Naphthalenyl[4-(pentyloxy)-1-naphthalenyl]methanone; NPs, nanoparticles.](/cms/asset/7d31c803-95c0-441a-abc9-7d247952c793/dijn_a_34633_f0009_b.jpg)
Conclusion
Successful CB13-loaded PLGA NPs were produced using the nanoprecipitation method. NPs obtained with different types of Resomer showed adequate properties in relation to particle size, drug content, in vitro release, and cell culture cytotoxicity. Results showed that the main influence on particle size was the hydrophobicity of the polymer: the higher the hydrophobicity, the lower the particle size. In relation to drug content, highest values were achieved using Resomer 502. These results indicate that when hydrophobic drugs are used, drug loading is closely matched with the respective solid-state drug–polymer solubility; that is, the ability of the polymeric matrix to entrap drug in dispersed state. Moreover, the cytotoxic assay results indicated that these formulations showed no cytotoxicity and initially, may be considered to be appropriate for CB13 loaded-nanocarrier delivery purposes.
Acknowledgements
LMB is especially grateful to Junta de Andalucía (Spain) for financial support (Project Nr P09-CTS5029). MDL thanks the University of Seville for a grant from IV Research Plan of University of Seville. The authors are also grateful for the microscopy and NMR Services (CITIUS, University of Seville) technical support.
Disclosure
The authors report no conflicts of interest in this work.
References
- AcharyaSSahooSKPLGA nanoparticles containing various anti-cancer agents and tumour delivery by EPR effectAdv Drug Deliv Rev201163317018320965219
- HolgadoMAÁlvarez-FuentesJFernández-ArévaloMAriasJLPossibilities of poly(D,L-lactide-co-glycolide) in the formulation of nanomedicines against cancerCurr Drug Targets20111281096111121443478
- MishraBPatelBBTiwariSColloidal nanocarriers: a review on formulation technology, types and applications toward targeted drug deliveryNanomedicine20106192419447208
- DouroumisDFahrANano- and micro-particulate formulations of poorly water-soluble drugs by using a novel optimized techniqueEur J Pharm Biopharm200663217317516621482
- ChenHKhemtongCYangXChangXGaoJNanonization strategies for poorly water-soluble drugsDrug Discov Today2011167–835436020206289
- MarcatoPDDuránNNew aspects of nanopharmaceutical delivery systemsJ Nanosci Nanotechnol2008852216222918572633
- FasinuPPillayVNdesendoVMdu ToitLCChoonaraYEDiverse approaches for the enhancement of oral drug bioavailabilityBiopharm Drug Dispos201132418520921480294
- AhlinPKristlJKristlAVrecerFInvestigation of polymeric nanoparticles as carriers of enalaprilat for oral administrationInt J Pharm20022391–211312012052696
- GardinAKucherKKieseBAppel-DingemanseSCannabinoid receptor agonist 13, a novel cannabinoid agonist: first in human pharmacokinetics and safetyDrug Metab Dispos200937482783319144772
- BudhianASiegelSJWineyKIHaloperidol-loaded PLGA nanoparticles: systematic study of particle size and drug contentInt J Pharm2007336236737517207944
- FessiHPuisieuxFDevissaguetJPAmmouryNBenitaSNanocapsule formation by interfacial polymer deposition following solvent displacementInt J Pharm1989551R1R4
- GarcíaCVBreierARSteppeMSchapovalEEOppeTPDetermination of dexamethasone acetate in cream by HPLCJ Pharm Biomed Anal200331359760012615249
- Pérez-LozanoPGarcía-MontoyaEOrriolsAMiñarroMTicóJRSuñé-NegreJMDevelopment and validation of a new HPLC analytical method for the determination of alprazolam in tabletsJ Pharm Biomed Anal2004341197998715019032
- ZaxariouMPanderiIDevelopment and validation of a high-performance liquid chromatographic method for the determination of buspirone in pharmaceutical preparationsJ Pharm Biomed Anal2004351415015030878
- VollnerLBieniekDKorteFReview of analytical methods for identification and quantification of cannabis productsRegul Toxicol Pharmacol1986643483583027768
- HolgadoMACózar-BernalMJSalasSAriasJLÁlvarez-FuentesJFernández-ArévaloMProtein-loaded PLGA microparticles engineered by flow focusing: physicochemical characterization and protein detection by reversed-phase HPLCInt J Pharm20093801–214715419635540
- Garay-JimenezJCYoungAGergeresDGreenhalghKTurosEMethods for purifying and detoxifying sodium dodecyl sulfate-stabilized polyacrylate nanoparticlesNanomedicine2008429810518472305
- NehillaBJBergkvistMPopatKCDesaiTAPurified and surfactant-free coenzyme Q10-loaded biodegradable nanoparticlesInt J Pharm20083481–210711417692482
- SannaVRoggioAMPosadinoAMNovel docetaxel-loaded nanoparticles based on poly(lactide-co-caprolactone) and poly(lactide-co-glycolide-co-caprolactone) for prostate cancer treatment: formulation, characterization, and cytotoxicity studiesNanoscale Res Lett20116126021711774
- AbdelwahedWDegobertGStainmesseSFessiHFreeze-drying of nanoparticles: Formulation, process and storage considerationsAdv Drug Deliv Rev200658151688171317118485
- PregoCTorresDFernandez-MegiaENovoa-CarballalRQuiñoáEAlonsoMJChitosan-PEG nanocapsules as new carriers for oral peptide delivery: effect of chitosan pegylation degreeJ Control Release2006111329930816481062
- PalacioJOrozcoVHLópezBLEffect of the molecular weight on the physicochemical properties of poly(lactic acid) nanoparticles and on the amount of ovalbumin adsorptionJ Braz Chem Soc2011221223042311
- HuhKMChoYWParkKPLGA-PEG Block copolymers for drug formulationsDrug Dev Deliv [serial on the internet]200335 Available from: http://www.drugdeliverytech.com/ME2/dirmod.asp?sid=&nm=&type=Publishing&mod=Publications%3A%3AArticle&mid=8F3A7027421841978F18BE895F87F791&tier=4&id=BB85E8579021481EACBC7C3F0674348F. Accessed October 7, 2012.
- HomarMSuligojDGasperlinMPreparation of microcapsules with self-microemulsifying core by a vibrating nozzle methodJ Microencapsul2007241728117438943
- PanyamJWilliamsDDashALeslie-PeleckyDLabhasetwarVSolid-state solubility influences encapsulation and release of hydrophobic drugs from PLGA/PLA nanoparticlesJ Pharm Sci20049371804181415176068
- BudhianASiegelSJWineyKIControlling the in vitro release profiles for a system of haloperidol-loaded PLGA nanoparticlesInt J Pharm20083461–215115917681683
- JudefeindAde VilliersMMDrug loading into and in vitro release from nanosized drug delivery systemsde VilliersMMAramwitPKwonGSNanotechnology in Drug DeliveryNew YorkSpringer2009129162
- MaYZhengYLiuKNanoparticles of poly(lactide-co-glycolide)-d-a-tocopheryl polyethylene glycol 1000 succinate random copolymer for cancer treatmentNanoscale Res Lett2010571161116920596457
- MusumeciTVenturaCAGiannoneIPLA/PLGA nanoparticles for sustained release of docetaxelInt J Pharm20063251–217217916887303
- ChildsCEThe determination of polyethylene glycol in gamma globulin solutionsMicrochem J1975202190192
- TrimailleTPichotCElaïssariAFessiHBriançonSDelairTPoly(d,l-lactic acid) nanoparticle preparation and colloidal characterizationColloid Polym Sci20032811211841190
- FredenbergSWahlgrenMReslowMAxelssonAThe mechanisms of drug release in poly(lactic-co-glycolic acid)-based drug delivery systems – a reviewInt J Pharm20114151–2345221640806
- BerklandCKimKPackDWPLG microsphere size controls drug release rate through several competing factorsPharm Res20032071055106212880292
- ZilbermanMGrinbergOHRP-loaded bioresorbable microspheres: effect of copolymer composition and molecular weight on microstructure and release profileJ Biomater Appl200822539140717494966
- GasparMMBlancoDCruzMEAlonsoMJFormulation of L-asparaginase-loaded poly(lactide-co-glycolide) nanoparticles: influence of polymer properties on enzyme loading, activity and in vitro releaseJ Control Release1998521–253629685935
- HolgadoMAAriasJLCózarMJAlvarez-FuentesJGañán-CalvoAMFernández-ArévaloMSynthesis of lidocaine-loaded PLGA microparticles by flow focusing. Effects on drug loading and release propertiesInt J Pharm20083581–2273518372128
- ArpagausCSchafrothNSpray dried biodegradable polymers for controlled drug delivery systemsEuropean Industrial Pharmacy2011111013
- YeoYParkKControl of encapsulation efficiency and initial burst in polymeric microparticle systemsArch Pharm Res200427111214969330
- KatsikariAPatronidouCKiparissidesCArsenakisMUptake and cytotoxicity of poly(d,l-lactide-co-glycolide) nanoparticles in human colon adenocarcinoma cellsMat Sci Eng B2009165160164
- SahooSKPanyamJPrabhaSLabhasetwarVResidual polyvinyl alcohol associated with poly (D,L-lactide-co-glycolide) nanoparticles affects their physical properties and cellular uptakeJ Control Release200282110511412106981