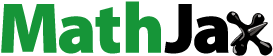
Abstract
Background and methods
Curcumin has extraordinary anticancer properties but has limited use due to its insolubility in water and instability, which leads to low systemic bioavailability. We have developed a novel nanoparticulate formulation of curcumin encapsulated in stearic acid-g-chitosan oligosaccharide (CSO-SA) polymeric micelles to overcome these hurdles.
Results
The synthesized CSO-SA copolymer was able to self-assemble to form nanoscale micelles in aqueous medium. The mean diameter of the curcumin-loaded CSO-SA micelles was 114.7 nm and their mean surface potential was 18.5 mV. Curcumin-loaded CSO-SA micelles showed excellent internalization ability that increased curcumin accumulation in cancer cells. Curcumin-loaded CSO-SA micelles also had potent antiproliferative effects on primary colorectal cancer cells in vitro, resulting in about 6-fold greater inhibition compared with cells treated with a solution containing an equivalent concentration of free curcumin. Intravenous administration of curcumin-loaded CSO-SA micelles marginally suppressed tumor growth but did not increase cytotoxicity to mice, as confirmed by no change in body weight. Most importantly, curcumin-loaded CSO-SA micelles were effective for inhibiting subpopulations of CD44+/CD24+ cells (putative colorectal cancer stem cell markers) both in vitro and in vivo.
Conclusion
The present study identifies an effective and safe means of using curcumin-loaded CSO-SA micelles for cancer therapy.
Introduction
Curcumin [bis(4-hydroxy-3-methoxyphenyl)-1,6-diene-3,5-dione] is the primary bioactive compound isolated from the rhizome of the Curcuma longa plant. It has been used for many clinical purposes, including as an antioxidant, antibacterial, anti-inflammatory agent, and anticancer compound, and exerts its effect mainly by influencing multiple signaling pathways.Citation1–Citation3 Inhibitory effects of curcumin lines have been demonstrated in various human cancer cell lines.Citation4,Citation5 In addition, a Phase I clinical trial showed that curcumin had low or no intrinsic toxicity in humans.Citation6 Despite these extraordinary properties, curcumin continues to have limited application in the treatment of cancer because of its considerable hydrophobicity, instability, and poor pharmacokinetics, which greatly hamper its efficacy in vivo.Citation7–Citation9
Nanoscale drug delivery systems are an innovative approach to overcoming these problems. Previous attempts at encapsulating curcumin in liposomes, phospholipid complexes, and other nanoparticle-based technologies have been reported, which appear to provide better water dispersibility and a longer circulation time.Citation10–Citation14 A key attribute of an optimal drug delivery system for cancer is the ability to treat tumors effectively with minimal side effects. In addition to having good solubility in water and stability, increased drug permeability and improved antitumor activity in vivo are also important factors. Recently, we have developed a novel stearic acid-g-chitosan oligosaccharide (CSO-SA) micelle which acts as a polymeric drug carrier due to its biocompatibility and biodegradability.Citation15 CSO-SA micelles, with their core-shell structure formed by self-aggregation from amphiphilic block or graft copolymers, have many advantages for delivering anticancer agents to tumor tissue. CSO-SA micelles are stable at room temperature or at 4°C with minimal change in particle size.Citation16 Moreover, these micelles accumulate well in tumor tissue via extravasation of the nanoparticulates in fenestrated tumor vasculature.Citation17,Citation18 After drug loading, these formulations show excellent tumor cell uptake characteristics. Importantly, the drug-loaded micelles have enhanced antitumor activity and overcome multidrug resistance in tumor tissue.Citation19,Citation20
Accumulating evidence continues to support the fact that many types of cancers, including colorectal cancer, are initiated and maintained by a minority cell population known as cancer stem cells. This cell subpopulation is responsible for tumor initiation and growth by continuous self-renewal and differentiation.Citation21,Citation22 Further, recent studies have shown that chemotherapy only destroys the most bulky cancer cells but lacks the ability to eradicate cancer stem cells. The survival of cancer stem cells leads to chemoresistance and tumor recurrence.Citation23,Citation24 Therefore, eradicating cancer stem cells may represent an effective chemotherapeutic strategy and thereby overcome tumor resistance.
In order to improve the outcome of cancer treatment, we designed and prepared curcumin-loaded CSO-SA micelles based on a polymeric nanosystem and evaluated their antitumor activity in primary colorectal cancer cells. We hypothesized that CSO-SA micelles would enhance the antitumor activity of curcumin by promoting its uptake in tumor tissue. Our findings provide the first evidence that curcumin-loaded CSO-SA micelles can increase antitumor efficacy and inhibit cancer stem cells both in vitro and in vivo, and suggest that they might be used as a novel therapeutic agent for cancer therapy in the future.
Materials and methods
Curcumin was purchased from Sigma-Aldrich, St Louis, MO. 3-(4,5-dimethylthiazol-2yl)-2,5-diphenyltetrazolium bromide (MTS) was obtained from Promega, Fitchburg, WI. Anti-human CD44-APC and CD24-FITC were purchased from BD Biosciences, San Diego, CA. Dulbecco’s Modified Eagle’s Medium and Ham’s F-12 medium (DMEM-F12 medium), RPMI 1640 medium, collagenase, and hyaluronidase were from Gibco BRL, Grand Island, NY. B27, epidermal growth factor, and basic fibroblast growth factor were sourced from Invitrogen, Carlsbad, CA. Fetal bovine serum was from Sijiqing Biologic, Hangzhou, China.
Synthesis and characteristics of CSO-SA
CSO-SA was prepared in the laboratory of FH (College of Pharmaceutical Sciences, Zhejiang University, China). As reported previously,Citation19 CSO-SA was synthesized by a coupling reaction between the carboxyl group of stearic acid (C18H36O2, Shanghai Chemical Reagent, Shanghai, China) and the amine groups of CSO (molecular weight 18 kDa) in the presence of a coupling agent, 1-ethyl-3-(3-dimethylaminopropyl) carbodiimide (EDC). EDC and stearic acid (EDC:SA 20:3, w/w) were dissolved in ethanol. After CSO (CSO:SA 2.4:1, w/w) solution was added, the mixture was stirred at 80°C for 4 hours, and then cooled down to room temperature. The reaction solution was dialyzed using a dialysis membrane with a molecular weight cutoff of 3.5 kDa against pure water for 3 days with successive exchange of fresh pure water in order to remove the ethanol. Finally, CSO-SA was obtained, and the critical micelle concentration was tested using a fluorescence method.Citation19 As described, the critical micelle concentration of CSO-SA was measured by fluorometry using pyrene as a probe (F-2500, Hitachi Co, Tokyo, Japan). The excitation wavelength was set at 337 nm and the slit at 2.5 nm. The emission intensities were monitored in the range of 360–450 nm. The emission intensity ratio of the first peak (I1, 374 nm) to the third peak (I3, 385 nm) was determined as the critical micelle concentration.
Preparation of curcumin-loaded CSO-SA micelles
Curcumin-loaded CSO-SA micelles were obtained using the following procedure. Blank CSO-SA micelle solution (4 mg/mL) was prepared by dissolution in 10 mL of pure water, with pH adjusted to 5.0 using 0.1 N HCl. The solution was subjected to probe-type ultrasonic treatment (400 W, 30 cycles with 2 seconds active, JY92-II, Scientz Biotechnology Co, Guangzhou, China), and stirred on a stir plate at 300 rpm. Curcumin dissolved in dimethylsulfoxide at a concentration of 10 mg/mL was then slowly added dropwise into the CSO-SA micelle solution (curcumin:CSO-SA 1:10, w/w). After stirring overnight at 4°C in the dark, the suspension was purified by dialysis (molecular weight cutoff 7 kDa) for 24 hours with successive exchange of fresh pure water (pH 5.0). Finally, the resulting product was centrifuged at 4000 rpm for 10 minutes, and the upper clear solution was then collected and stored at 4°C in the dark.
Physicochemical properties of curcumin-loaded CSO-SA micelles
The size, size distribution, and zeta potential of curcumin-loaded CSO-SA micelles were measured by dynamic light scattering with a Zetasizer (3000 HS, Malvern Instruments Ltd, Worcestershire, UK).
The surface appearance and shape of the curcumin-loaded CSO-SA micelles were observed by transmission electron microscopy (TEM, JEM-1230, JEOL, Tokyo, Japan). The samples were negatively stained with uranyl acetate solution (1%, w/v) and placed on copper grids (200 mesh) for viewing.
The encapsulation efficiency (EE) of curcumin-loaded CSO-SA micelles was determined using a fluorescence spectrophotometer (F-2500, Hitachi). In brief, a curcumin-loaded CSO-SA micelle solution was mixed with 10-fold dimethylsulfoxide, and the fluorescence intensity was then detected. The curcumin content was estimated by comparison with a standard curve obtained for an aqueous solution of curcumin and dimethylsulfoxide (dimethylsulfoxide:H2O, 9:1, v:v). The EE for curcumin was quantified using the following equation:
In vitro stability of curcumin-loaded CSO-SA micelles
To assess their in vitro stability, curcumin-loaded CSO-SA micelles were mixed with phosphate-buffered solution (pH 7.2) or phosphate-buffered solution (pH 7.2) supplemented with 10% (v/v) fetal bovine serum, resulting in a final curcumin concentration of 50 μg/mL. The mixture was then put into Eppendorf tubes giving five different samples for study at various time intervals, with each set of samples being tested in triplicate. The tubes were incubated at 37°C under gentle agitation. The concentration of curcumin in the tubes was quantified by fluorescence spectrophotometry (λex 420 nm and λem 540 nm). Curcumin retention was calculated using the following equation:
In vitro release studies
In vitro release of curcumin from the CSO-SA micelles was evaluated using a previously reported dialysis method.Citation25,Citation26 To avoid degradation, a curcumin-loaded CSO-SA micelle solution was diluted with phosphate-buffered solution (pH 5.0) to a final curcumin concentration of 50 μg/mL. A dialysis bag (molecular weight cutoff 7.0 kDa) containing 1 mL of curcumin-loaded CSO-SA micelles was then immersed in a plastic tube containing 10 mL of phosphate-buffered solution (pH 6.0). The release experiments were conducted in an incubator shaker at 37°C, with shaking at 60 rpm. At various time intervals, phosphate-buffered solution in the tube was collected and replaced with fresh medium. The amount of drug released was quantified by fluorescence spectrophotometry. All drug release tests were performed three times.
Cell culture
Primary colorectal cancer cells were obtained from tissue fragments of patients with colorectal adenocarcinoma as described elsewhere,Citation27 in accordance with the ethical standards of the Institutional Committee on Human Experimentation. Cancer tissues were minced and digested for one hour at 37°C with collagenase (1.25 mg/mL) and hyaluronidase (1.5 mg/mL). The cancer cells were then cultured in DMEM-F12 medium (pH 7.2) at 37°C in a humidified atmosphere containing 5% CO2. The medium was supplemented with 10% (v/v) fetal bovine serum, penicillin 100 U/mL, and streptomycin 100 μg/mL.
Intracellular uptake of curcumin-loaded CSO-SA micelles
Round glass coverslips were placed in the wells of a 24-well plate (Corning, New York, NY) and colorectal cancer cells were seeded at densities of 1 × 105 per well. After being allowed to grow for 24 hours, the cells were incubated with free curcumin (0.5 μg/mL) or curcumin-loaded CSO-SA micelles (curcumin content 0.5 μg/mL) in growth medium (pH 7.2) for an additional one hour or 4 hours, respectively. Finally, the cells were washed with phosphate-buffered solution three times, and the coverslips with the cells were removed from the wells. Cellular uptake was visualized by confocal microscopy (Zeiss LSM 710, Carl Zeiss, Dublin, CA).
Cytotoxicity assay
Cytotoxicity was assessed by MTS assay using the CellTiter 96 Aqueous MTS. Briefly, colorectal cancer cells were cultured at a density of 2 × 104 to 5 × 104 cells per well in 96-well plates. After 24 hours, the cells were exposed to a series of concentrations of free curcumin (dissolved in dimethylsulfoxide) and curcumin-loaded CSO-SA micelles (dispersed in 100 μL/well DMEM-F12 medium at pH 7.2 with 10% serum), and incubated for a further 48 hours. The final concentration of dimethylsulfoxide in the culture medium was less than 0.1%. Cell viability was measured by MTS according to the manufacturer’s instructions.
Detection of cancer stem cell markers by flow cytometry
Expression of surface markers was determined with flow cytometry. Single cells obtained from cell cultures or xenograft tumors were labeled with mouse anti-human CD44-APC and CD24-FITC at concentrations recommended by the manufacturer. Mouse IgG was used as an isotype control. A typical antibody staining procedure was performed according to the standard protocol. The cells were then analyzed using a FACSCalibur machine (Becton Dickinson, Franklin Lakes, NJ).
Spheroid assay
Colorectal cancer stem cells are enriched in spheroid of cancer cells, based on one of the characteristics of cancer stem cells is their ability to form spheroid in serum-free medium, whereas differentiated cells fail to survive under the same condition.Citation28,Citation29 Spheroid culture was done in DMEM-F12 basal serum-free medium supplemented with B27, 20 ng/mL epidermal growth factor, 10 ng/mL basic fibroblast growth factor, 2 mg/mL bovine serum albumin, 100 U/mL penicillin, and 100 μg/mL streptomycin. Single cells prepared from enzymatic dissociation were plated in 96-well ultralow attachment plates (Corning) at a density of 100–200 cells/well. The potential for spheroid formation was assessed after incubation with the trial agents for 14 days without changing the medium.
Antitumor activity of curcumin-loaded CSO-SA micelles in vivo
All animal experiments were conducted in accordance with the standard protocol approved by the Zhejiang Chinese Medicine University Institutional Animal Care and Use Committee. Colorectal cancer cells were injected subcutaneously into the flank of 5-week-old nude mice. When tumor size reached a mean diameter of about 7–9 mm, the mice were randomized into three treatment groups (n = 6 per group), ie, phosphate-buffered solution alone, empty vehicle, and curcumin-loaded CSO-SA micelles (curcumin content 2 mg/kg). In this study, a native curcumin group was not included because curcumin is practically insoluble in water and unstable in both phosphate-buffered solution and 0.9% saline solution. Each group was treated once a day for two successive weeks (for a total of 14 treatments) by intravenous injection through the tail vein. The tumor was measured with digital calipers and the volume was calculated using the formula:
where a is the smallest diameter and b is the largest diameter.Citation30
At the end of treatment, the tumors were harvested from the mouse xenografts, and tissue samples were used for analysis of curcumin biodistribution, whereby tumors were sliced into frozen tissue sections 10 mm thick, and randomly chosen fields in the section were then examined at 200× using a confocal microscope. The samples were also used to investigate cancer stem cell surface marker expression. Tumor tissues were dissociated mechanically, and enzymatic digestion was then performed using collagenase-hyaluronidase in RPMI 1640 medium at 37°C for 1–2 hours. A single-cell suspension was obtained through a 40 μm mesh, and used for flow cytometry.
Statistical analysis
The data were expressed as the mean of three independent experiments, and statistical significance was determined by one-way analysis of variance. A P value < 0.05 was considered to be statistically significant.
Results and discussion
Preparation and characteristics of curcumin-loaded CSO-SA micelles
In this work, the synthesized CSO-SA copolymer could easily self-assemble to form micelles in aqueous medium. The critical micelle concentration was about 0.14 mg/mL, which is consistent with our previous results.Citation16 The low critical micelle concentration enabled CSO-SA to retain a spatial structure with a multihydrophobic core in aqueous medium even under highly diluted conditions. The average size of the CSO-SA micelles as measured by Zetasizer was 25.4 nm and the zeta potential was 29.9 mV in pure water (). After curcumin was loaded, the size increased to 114.7 nm and the zeta potential decreased to 18.5 mV, indicating entrapment of curcumin within the micelle network. In addition, the EE of curcumin-loaded CSO-SA micelles was about 29.9 wt% with the preparation method used in this study.
Table 1 Characteristics of CSO-SA and curcumin-loaded CSO-SA micelles
The size distribution and spherical morphology of CSO-SA and curcumin-loaded CSO-SA micelles was detected by dynamic light scattering and TEM, respectively (). The size of the micelles observed in the TEM images corresponded to that shown in . We have previously reported that hydrophobic drugs become partitioned within hydrophobic domains and situated inside the core portion of the CSO-SA micelles, resulting in increased particle size.Citation19,Citation31 Accordingly, we speculated that the CSO-SA micelles entrapping curcumin had similar properties. It should be noted that the size of the polymeric drug carriers played a vital role in interaction with the cells and also in toxicity, given that the smaller-sized particles enhanced cellular interactions and therefore might possess increased intrinsic toxicity. In addition, a size less than 200 nm is desirable for nanoparticles to increase their accumulation in tumor tissue via the fenestrated tumor vasculature, and to reduce their recognition and entrapment by the reticular endothelial system.Citation32,Citation33 Therefore, we can reasonably assume that the size of the curcumin-loaded CSO-SA micelles was small enough to have high extravasation efficiency.
Figure 1 Characterization of curcumin-loaded CSO-SA micelles. (A) Size distribution of curcumin-loaded CSO-SA micelles. (B) Transmission electron microscopic images of curcumin-loaded CSO-SA micelles. (C) Release profile of curcumin from curcumin-loaded CSO-SA micelles in phosphate-buffered solution at 37°C.
Note: Data are presented as the mean ± standard deviation (n = 3).
Abbreviations: Cur, curcumin; CSO-SA, stearic acid-g-chitosan oligosaccharide.

To prevent any significant degradation of curcumin as a result of the long exposure time at neutral pH, the drug-release profile of curcumin-loaded CSO-SA micelles was assessed in phosphate-buffered solution (pH 6.0). Over 48 hours, more than 40% of the total amount of curcumin was released from the micelles at 37°C (). The release curves show that the drug-release process was stable without any explosive release.
Improved solubility and stability of curcumin-loaded CSO-SA micelles
Curcumin is poorly soluble in water and subject to rapid hydrolytic degradation at physiological pH. To avoid degradation, curcumin was loaded into the CSO-SA micelles at pH 5.0. The drug was hidden in the hydrophobic groups forming the internal core of the micelles and the curcumin-loaded CSO-SA micelles could easily disperse from the hydrophilic groups on the outside into the aqueous medium with a natural color, while free curcumin was poorly soluble leading to precipitation of particles on the bottom of the tube ().
Figure 2 Solubility and stability of CSO-SA/Cur micelles. (A) Free curcumin is poorly soluble in aqueous medium in tube (a). In contrast, CSO-SA/Cur micelles are completely dispersed in aqueous medium in tube (b). (B) Stability test of curcumin in PBS (pH 7.2) and PBS containing 10% FBS.
Note: Data are presented as the mean ± standard deviation (n = 3).
Abbreviations: Cur, curcumin; CSO-SA, stearic acid-g-chitosan oligosaccharide; FBS, fetal bovine serum; PBS, phosphate-buffered solution.
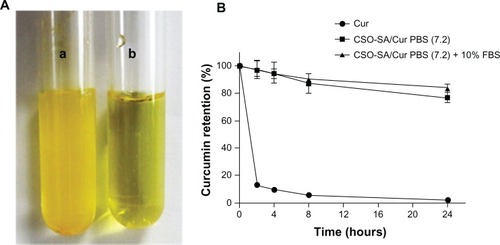
To confirm the stability of our formulation, curcumin-loaded CSO-SA micelles were assessed in phosphate-buffered solution at neutral pH or in phosphate-buffered solution supplemented with 10% fetal bovine serum. We found that the encapsulated curcumin was relatively stable (80%) at neutral pH (), whereas free curcumin underwent rapid degradation in phosphate-buffered solution (pH 7.2), with less than 10% of curcumin remaining after 24 hours, which is consistent with the findings of a previous study.Citation7,Citation34 Our results indicate that curcumin-loaded CSO-SA micelles could increase the stability of curcumin in phosphate-buffered solution at neutral pH by protecting the encapsulated curcumin against hydrolysis and biotransformation.
Cellular uptake studies
Cellular uptake tests of curcumin-loaded CSO-SA micelles were performed by visualizing the intrinsic green fluorescence of curcumin using confocal microscopy. Images of the cells incubated with encapsulated and free curcumin showed green fluorescence under similar conditions (). Interestingly, more intense and homogeneously dispersed fluorescence was apparent in the cytoplasm and nucleus after 4 hours of incubation with curcumin-loaded CSO-SA micelles. It was observed that uptake of curcumin by cells increased when curcumin was encapsulated in CSO-SA micelles. This could be a consequence of interaction between cationic CSO-SA micelles and the negatively charged cell surface. Further, uptake of curcumin-loaded CSO-SA micelles might be via endocytosis, whereby free curcumin became internalized into tumor cells via a molecular diffusion mechanism.Citation35 Loading of curcumin into CSO-SA micelles could provide an efficient route for transporting the drug across the cell membrane, especially for drug-resistant tumor cells. These observations indicate that the CSO-SA micelles designed in this study could act as an effective intracellular drug delivery system.
In vitro antitumor activity
The cytotoxicity of free curcumin, the empty vector, and the curcumin-loaded CSO-SA micelles was investigated by MTS assay using colorectal cancer cells. The free curcumin was dissolved in dimethylsulfoxide, and the final concentration of dimethylsulfoxide was less than 0.1% in cell culture. This concentration of dimethylsulfoxide had little effect on cell growth. The dose-response curves showed that the cytotoxicity of curcumin-loaded CSO-SA micelles was about 6-fold higher than that of a solution containing an equivalent concentration of free curcumin ().
Figure 4 (A) Cytotoxicity of free curcumin and curcumin-loaded CSO-SA micelle solution toward primary colorectal cancer cells. (B) Results for colorectal cancer cells treated with free curcumin solution included as a control.
Note: Data are presented as the mean ± standard deviation (n = 3).
Abbreviations: Cur, curcumin; CSO-SA, stearic acid-g-chitosan oligosaccharide.

However, blank CSO-SA micelles showed only slight cytotoxicity in cancer cells after 48 hours of incubation at concentrations up to 240 μg/mL (equivalent to drug-loaded CSO-SA micelles containing 8 μg/mL curcumin, ). This indicates that the CSO-SA micelles have low toxicity and are safe as drug carriers. In contrast, cell viability decreased by over 50% on exposure to curcumin-loaded CSO-SA micelles containing 6-fold lower concentrations of free curcumin. Considering that micelles increased the stability of curcumin in solution and promoted uptake by cells in this colorectal cancer model, the greater cytotoxicity of the curcumin-loaded CSO-SA micelles in comparison with that of free curcumin is understandable. Overall, these findings provide evidence that CSO-SA micelles can improve the anticancer activity of curcumin.
Cancer stem cell surface marker expression
There is recent evidence suggesting that cancer stem cells drive tumor growth and recurrence.Citation36 Although there are several putative markers for cancer stem cells, coexpression of the CD44+/24+ surface marker in colorectal cancer cells suggests stemness with enhanced spheroid-forming ability in vitro and increased tumorigenicity in vivo.Citation37,Citation38 In order to evaluate whether curcumin-loaded CSO-SA micelles can inhibit cancer stem cells, we examined the expression of colorectal cancer stem cell surface markers after treatment with free curcumin (0.25 μg/mL), curcumin-loaded CSO-SA micelles (curcumin content 0.25 μg/mL), and oxaliplatin (1 μg/mL) for 7 days. We observed that the proportion of CD44+/CD24+ cells significantly decreased after exposure to curcumin-loaded CSO-SA micelles compared with the untreated and oxaliplatin groups (P < 0.05, ). These findings are consistent with those of previous studies showing that curcumin can eliminate colorectal cancer stem cells.Citation39,Citation40 Moreover, there is evidence showing that failure of conventional chemotherapy, such as oxaliplatin, is closely related to enrichment of cancer stem cells, which are more resistant to anticancer drugs than are bulk cancer cells, and that cancer stem cells drive chemoresistance.Citation41 Our results suggest that curcumin-loaded CSO-SA micelles are very suitable for overcoming these problems in vitro.
Figure 5 Characterization of cell surface marker expression in primary colorectal cancer cells. (A) Ratio of CD44+/CD24+ cell subpopulation in total cancer cells. Data are presented as the mean ± standard deviation (*P < 0.05, all others versus untreated group). (B) Representative flow cytometry plots for the experiments.
Note: Q2 covers the region of the CD44 and CD24 double-positive cells (isotype control profiles on all channels).
Abbreviations: Cur, curcumin; CSO-SA, stearic acid-g-chitosan oligosaccharide.
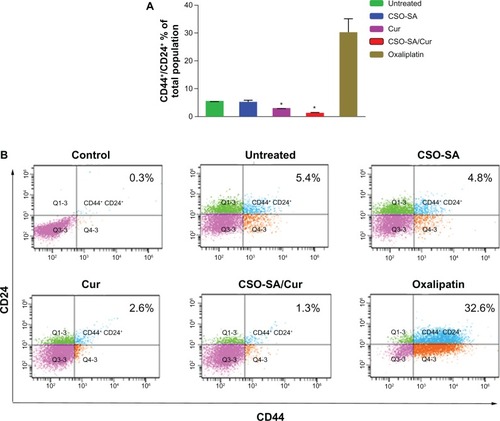
Spheroid formation
To evaluate whether curcumin-loaded CSO-SA micelles could influence formation of spheroids, colorectal cancer cells were incubated with curcumin (0.25 μg/mL), curcumin-loaded CSO-SA micelles (curcumin content 0.25 μg/mL), CSO-SA micelles, or phosphate-buffered solution alone for 14 days under spheroid-forming conditions. The curcumin-loaded CSO-SA micelles were added at a concentration of 0.25 μg/mL based on dose-response experiments (data not shown). The results show that curcumin-loaded CSO-SA micelles could decrease spheroid formation compared with colorectal cancer cells that were untreated or exposed to empty drug carriers (). It was worth noting that the concentration of curcumin-loaded CSO-SA micelles capable of suppressing spheroid formation was 6-fold lower than that required for antiproliferative effects in the MTS assay. The data indicate that curcumin-loaded CSO-SA micelles could greatly decrease the potential for spheroid formation in colorectal cancer cells.
Figure 6 Effect of curcumin-loaded CSO-SA micelles on growth of primary colorectal cancer cells and formation of spheroids.
Note: The size of the spheroids was visualized by microscopy after the cancer cells were exposed to the experimental treatments for 14 days.
Abbreviations: Cur, curcumin; CSO-SA, stearic acid-g-chitosan oligosaccharide.

In vivo antitumor activity
The antitumor efficacy of curcumin-loaded CSO-SA micelles was assessed in an orthotopic colorectal tumor-bearing nude mouse model. In our study, we determined that a well tolerated dose of curcumin-loaded CSO-SA micelles was 2 mg/kg. We did not include a free curcumin group because of its insolubility in water and instability in phosphate-buffered solution. After intravenous injection for 14 days, curcumin-loaded CSO-SA micelles only marginally inhibited colorectal tumor growth (P < 0.05, ). In contrast, the empty vehicle had no effect. Moreover, the absence of any obvious reduction in body weight in any of the treatment groups suggested that the doses chosen were within the safe range (). These observations were corroborated by our in vitro cytotoxicity results.
Figure 7 In vivo antitumor activity of curcumin-loaded CSO-SA micelles after intravenous injection via the tail vein in tumor-bearing nude mice. (A) Mice tumor volume changes during the experiment. (B) Changes in mouse body weight during the experiment. (C) Scanning confocal images of curcumin-loaded CSO-SA micelles at 48 hours after the last treatment in xenograft tumor tissues.
Note: Curcumin shows intrinsic green fluorescence.
Abbreviations: Cur, curcumin; CSO-SA, stearic acid-g-chitosan oligosaccharide; PBS, phosphate-buffered solution.
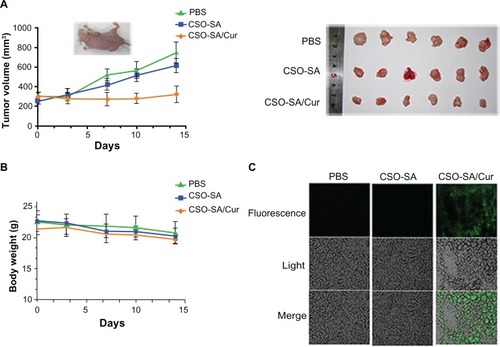
It is well known that curcumin administered orally has poor distribution in tumors due to high first-pass metabolism.Citation42 Therefore, we identified the distribution of curcumin-loaded CSO-SA micelles in xenograft tumor tissues by confocal microscopy at the end of the treatment period. The results demonstrated that curcumin-loaded CSO-SA micelles achieved a high level of accumulation in tumor tissue (). We speculate that curcumin-loaded CSO-SA micelles might increase the bioavailability of curcumin, improve its accumulation at the tumor target site via fenestrated tumor vasculature, and maintain effective therapeutic concentrations for a relatively long time.
We further examined the effects of each experimental treatment on the CD44+/CD24+ cell subpopulation in tumor tissue, and found that curcumin-loaded CSO-SA micelles decreased this subpopulation of cells significantly compared with the phosphate-buffered solution and empty vehicle groups (P < 0.05, ). Importantly, we have previously observed that tumor growth can be delayed in patients while receiving chemotherapy, but is often accelerated after cessation of treatment. This is consistent with the hypothesis that cancer stem cells are more resistant to drug treatment than are other cancer cells, leading to rapid tumor regrowth. Our previous results have confirmed this theory, showing that colorectal cancer stem cells are more resistant than bulk cancer cells to oxaliplatin, and are even enriched after drug administration in xenograft tumors.Citation16 This suggests that one highly desirable strategy to address this concern would be to identify agents that eliminate all types of cancer cells within tumors, including cancer stem cells.Citation43 In our study, we found that treatment with curcumin-loaded CSO-SA micelles not only reduced tumor size, but also inhibited the CD44+/CD24+ cell population. This suggests that curcumin-loaded CSO-SA micelles could eliminate cancer stem cells as well as bulk cancer cells, providing significant advantages in cancer therapy.
Figure 8 CD44+/CD24+ cell subpopulation in xenograft tumors. (A) Ratio of CD44+/CD24+ cell subpopulation in tumors. The data are presented as the mean ± standard deviation of four tumors per group. *P < 0.05. (B) Representative flow cytometry plots for the experiments.
Note: Q2 covers the region of the CD44 and CD24 double-positive cells (isotype control profiles on all channels).
Abbreviations: Cur, curcumin; CSO-SA, stearic acid-g-chitosan oligosaccharide; PBS, phosphate-buffered solution.
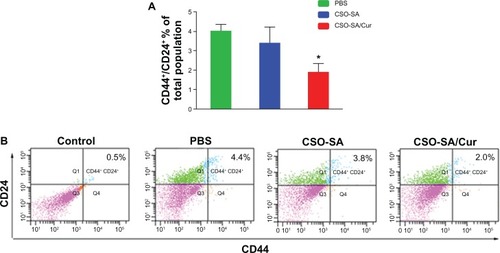
Conclusion
Curcumin-loaded CSO-SA micelles were prepared successfully in this study. The synthesized curcumin-loaded CSO-SA copolymer could self-assemble to form nanosized micelles in aqueous medium with excellent internalization ability. The results clearly demonstrate that curcumin-loaded CSO-SA micelles can increase antitumor efficacy and eliminate colorectal cancer stem cells in vitro. Moreover, intravenous administration of curcumin-loaded CSO–SA micelles marginally suppressed tumor growth and inhibited cancer stem cell enrichment. The enhanced therapeutic efficacy of curcumin-loaded CSO-SA micelles might be attributable to the increased stability of curcumin in solution, and an improved drug carrier for transportation to cancer cells and subsequent cell death. Overall, our study suggests that curcumin-loaded CSO-SA micelles are highly beneficial in the treatment of cancer.
Acknowledgements
This work was supported by funds from the Zhejiang Provincial Natural Science Foundation of China (Z2100366 and Y2090386), the Joint Project between Zhejiang Province and the Ministry of Health (WKJ20092024), the Zhejiang Provincial Program for the Cultivation of High-level Innovative Health Talents (HJ), the 151 Talent Project of Zhejiang Province (HJ), Zhejiang Medical Research Program (2012ZZ010) and the Doctoral Fund of the Ministry of Education of China (20100101110124).
Disclosure
The authors declare no conflicts of interest in relation to this paper.
References
- AggarwalBBShishodiaSMolecular targets of dietary agents for prevention and therapy of cancerBiochem Pharmacol200671101397142116563357
- AggarwalBBSungBPharmacological basis for the role of curcumin in chronic diseases: an age-old spice with modern targetsTrends Pharmacol Sci2009302859419110321
- ShishodiaSChaturvediMMAggarwalBBRole of curcumin in cancer therapyCurr Probl Cancer200731424330517645940
- RaoCVRivensonASimiBReddyBSChemoprevention of colon carcinogenesis by dietary curcumin, a naturally occurring plant phenolic compoundCancer Res19955522592667812955
- SinghSKharABiological effects of curcumin and its role in cancer chemoprevention and therapyAnticancer Agents Med Chem20066325927016712454
- ChengALHsuCHLinJKPhase I clinical trial of curcumin, a chemopreventive agent, in patients with high-risk or pre-malignant lesionsAnticancer Res2001214B2895290011712783
- WangYJPanMHChengALStability of curcumin in buffer solutions and characterization of its degradation productsJ Pharm Biomed Anal19971512186718769278892
- TonnesenHHMassonMLoftssonTStudies of curcumin and curcuminoids. XXVII. Cyclodextrin complexation: solubility, chemical and photochemical stabilityInt J Pharm20022441–212713512204572
- ShobaGJoyDJosephTMajeedMRajendranRSrinivasPSInfluence of piperine on the pharmacokinetics of curcumin in animals and human volunteersPlanta Med19986443533569619120
- TangHMurphyCJZhangBAmphiphilic curcumin conjugate-forming nanoparticles as anticancer prodrug and drug carriers: in vitro and in vivo effectsNanomedicine (Lond)20105685586520735222
- DuanJZhangYHanSSynthesis and in vitro/in vivo anti-cancer evaluation of curcumin-loaded chitosan/poly(butyl cyanoacrylate) nanoparticlesInt J Pharm20104001–221122020813175
- KimTHJiangHHYounYSPreparation and characterization of water-soluble albumin-bound curcumin nanoparticles with improved antitumor activityInt J Pharm20114031–228529121035530
- DasRKKasojuNBoraUEncapsulation of curcumin in alginate-chitosan-pluronic composite nanoparticles for delivery to cancer cellsNanomedicine20106115316019616123
- TakahashiMUechiSTakaraKAsikinYWadaKEvaluation of an oral carrier system in rats: bioavailability and antioxidant properties of liposome-encapsulated curcuminJ Agric Food Chem200957199141914619757811
- HuFQRenGFYuanHDuYZZengSShell cross-linked stearic acid grafted chitosan oligosaccharide self-aggregated micelles for controlled release of paclitaxelColloids Surf B Biointerfaces20065029710316759840
- WangKLiuLZhangTOxaliplatin-incorporated micelles eliminate both cancer stem-like and bulk cell populations in colorectal cancerInt J Nanomedicine201163207321822238509
- HuFQWuXLDuYZYouJYuanHCellular uptake and cytotoxicity of shell crosslinked stearic acid-grafted chitosan oligosaccharide micelles encapsulating doxorubicinEur J Pharm Biopharm200869111712517997293
- YouJHuFQDuYZYuanHPolymeric micelles with glycolipid-like structure and multiple hydrophobic domains for mediating molecular target delivery of paclitaxelBiomacromolecules2007882450245617661518
- HuFQLiuLNDuYZYuanHSynthesis and antitumor activity of doxorubicin conjugated stearic acid-g-chitosan oligosaccharide polymeric micellesBiomaterials200930366955696319782395
- HuFQMengPDaiYQPEGylated chitosan-based polymer micelle as an intracellular delivery carrier for anti-tumor targeting therapyEur J Pharm Biopharm200870374975718620050
- O’BrienCAKresoADickJECancer stem cells in solid tumors: an overviewSemin Radiat Oncol2009192717719249644
- HermannPCBhaskarSCioffiMHeeschenCCancer stem cells in solid tumorsSemin Cancer Biol2010202778420371287
- GangemiRPaleariLOrengoAMCancer stem cells: a new paradigm for understanding tumor growth and progression and drug resistanceCurr Med Chem200916141688170319442140
- DeanMFojoTBatesSTumour stem cells and drug resistanceNat Rev Cancer 0055427528415803154
- GouMMenKShiHCurcumin-loaded biodegradable polymeric micelles for colon cancer therapy in vitro and in vivoNanoscale2011341558156721283869
- WuWShenJBanerjeePZhouSWater-dispersible multifunctional hybrid nanogels for combined curcumin and photothermal therapyBiomaterials201132259860920933280
- WangYKZhuYLQiuFMActivation of Akt and MAPK pathways enhances the tumorigenicity of CD133+ primary colon cancer cellsCarcinogenesis20103181376138020530554
- CammareriPLombardoYFrancipaneMGBonventreSTodaroMStassiGIsolation and culture of colon cancer stem cellsMethods Cell Biol20088631132418442654
- Ricci-VitianiLLombardiDGPilozziEIdentification and expansion of human colon-cancer-initiating cellsNature2007445712311111517122771
- FeldmannGDharaSFendrichVBlockade of hedgehog signaling inhibits pancreatic cancer invasion and metastases: a new paradigm for combination therapy in solid cancersCancer Res20076752187219617332349
- HuFQZhaoMDYuanHYouJDuYZZengSA novel chitosan oligosaccharide-stearic acid micelles for gene delivery: properties and in vitro transfection studiesInt J Pharm20063151–215816616632285
- LukyanovANGaoZMazzolaLTorchilinVPPolyethylene glycol-diacyl lipid micelles demonstrate increased accumulation in subcutaneous tumors in micePharm Res200219101424142912425458
- AlexisFPridgenEMolnarLKFarokhzadOCFactors affecting the clearance and biodistribution of polymeric nanoparticlesMol Pharm20085450551518672949
- MohantyCSahooSKThe in vitro stability and in vivo pharmacokinetics of curcumin prepared as an aqueous nanoparticulate formulationBiomaterials201031256597661120553984
- VenkatesanPPuvvadaNDashRThe potential of celecoxib-loaded hydroxyapatite-chitosan nanocomposite for the treatment of colon cancerBiomaterials201132153794380621392822
- ReyaTMorrisonSJClarkeMFWeissmanILStem cells, cancer, and cancer stem cellsNature2001414685910511111689955
- VermeulenLTodaroMde Sousa MelloFSingle-cell cloning of colon cancer stem cells reveals a multi-lineage differentiation capacityProc Natl Acad Sci U S A200810536134271343218765800
- YeungTMGandhiSCWildingJLMuschelRBodmerWFCancer stem cells from colorectal cancer-derived cell linesProc Natl Acad Sci U S A201010783722372720133591
- YuYKanwarSSPatelBBNautiyalJSarkarFHMajumdarAPElimination of colon cancer stem-like cells by the combination of curcumin and FOLFOXTransl Oncol20092432132819956394
- LinLLiuYLiHTargeting colon cancer stem cells using a new curcumin analogue, GO-Y030Br J Cancer2011105221222021694723
- RichJNBaoSChemotherapy and cancer stem cellsCell Stem Cell20071435335518371369
- SharmaRAStewardWPGescherAJPharmacokinetics and pharmacodynamics of curcuminAdv Exp Med Biol200759545347017569224
- GuptaPBOnderTTJiangGIdentification of selective inhibitors of cancer stem cells by high-throughput screeningCell2009138464565919682730