Abstract
Nasopharyngeal carcinoma (NPC) is a common malignant tumor of the head and neck with a high incidence rate worldwide, especially in southern China. Phototheranostics in combination with nanoparticles is an integrated strategy for enabling simultaneous diagnosis, real-time monitoring, and administration of precision therapy for nasopharyngeal carcinoma (NPC). It has shown great potential in the field of cancer diagnosis and treatment owing to its unique noninvasive advantages. Many Chinese and international research teams have applied nano-targeted drugs to optical diagnosis and treatment technology to conduct multimodal imaging and collaborative treatment of NPC, which has become a hot research topic. In this review, we aimed to introduce the recent developments in phototheranostics of NPC based on a nanoplatform. This study aimed to elaborate on the applications of nanoplatform-based optical imaging strategies and treatment modalities, including fluorescence imaging, photoacoustic imaging, Raman spectroscopy imaging, photodynamic therapy, and photothermal therapy. This study is expected to provide a scientific basis for further research and development of NPC diagnosis and treatment.
Introduction
Along with the rapid progress of modern society, greater attention and efforts have been dedicated to the health problems of human beings, particularly for cancer. Cancer is a serious public health concern that has not been well addressed worldwide.Citation1 Nasopharyngeal carcinoma (NPC) is a malignant tumor that arises from the epithelium of the nasopharyngeal mucosa and it is common in the head and neck. It has a high incidence rate in Southeast Asia, Southern China, North Africa, and Alaska.Citation2,Citation3 In recent years, imaging has played a key role in the screening, diagnosis, staging, and monitoring of therapeutic interventions for NPC. Comprehensive analysis of various imaging examinations has great significance for the treatment of patients with NPC. Currently, the main diagnostic methods for NPC include flexible fiberoptic nasopharyngoscopy pathological biopsy, blood EB-DNA testing, computed tomography (CT), magnetic resonance imaging (MRI), emission computed tomography (ECT), and positron emission computed tomography (PET).Citation4–Citation9 Although these traditional clinical imaging techniques can offer deep tissue penetration and high spatial resolution, they often fail to meet comprehensive clinical needs. In contrast, optical imaging technologies are cost-effective, rapid, easy to use, safe from ionizing radiation, and can be readily applied to study disease processes and biology in vivo, especially in oncology, which compensates for the drawbacks of traditional clinical imaging techniques.Citation10,Citation11 Comparisons between traditional medical imaging techniques and optical imaging technologies are summarized in . Common optical imaging techniques for studying NPC include fluorescence imaging (FLI), photoacoustic imaging (PAI), and Raman spectroscopy imaging (RSI). These optical techniques result from the coalescence of technologies from chemistry, physics, and biology disciplines.
Table 1 Comparison Between Optical Imaging Technologies and Traditional Clinical Imaging Modalities in Clinical Application of Tumor Disease
In addition, treatment methods of NPC mainly include surgery, radiotherapy, chemotherapy and biotherapy, immunotherapy, etc.Citation12–Citation14 However, these clinical therapy methods encounter various difficulties, such as no targeted effect, poor systemic distribution, large toxicity and side effects, and poor prognosis. Therefore, improving the accuracy of therapy in NPC is urgently needed, and the advent of nano-targeted drugs and optical therapy technologies, such as photodynamic therapy (PDT) and photothermal therapy (PTT), offers the opportunity to overcome these problems accordingly ().Citation15–Citation20 summarizes the specific differences between the optical therapy and other traditional methods.
Table 2 Comparison Between Optical Therapy Modalities and Traditional Clinical Treatments in Clinical Application of Tumor Disease
Figure 1 Schematic illustration of multi-modal optical imaging and combined phototherapy of nasopharyngeal carcinoma based on a nanoplatform.
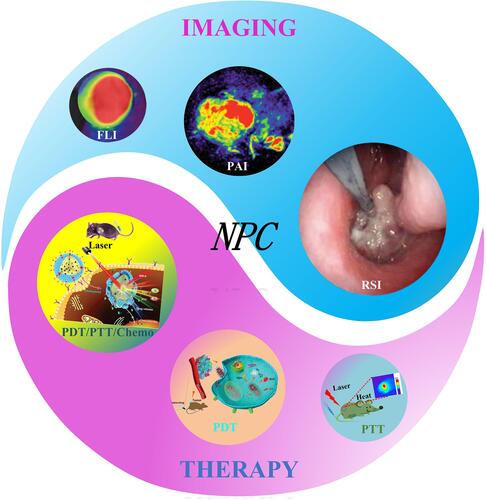
Nanomaterials Used for the Diagnosis and Therapy of NPC
Nanotechnology has achieved remarkable progress in biology and medicine and has been extensively applied in the therapy and diagnosis of oncology in recent decades.Citation21–Citation25 With the application of nanotechnology for tumor imaging diagnosis and treatment, the therapeutic effects of nano-targeted drugs on NPC have been continuously investigated in China and abroad. The surface of nanomaterials can be easily modified, their particle size and surface charge regulated, and the high porosities and large specific surface areas of nanoparticles highlight many advantages of nanomaterials in the treatment of NPC,Citation26,Citation27 namely (1) precise targeting of tumor, overcoming drug resistance, improving the curative effect, and reducing the toxic and side effects of drugs on the body; (2) controllable stability, solubility, cyclic half-life, drug load, and other biological characteristics of nanoparticles; (3) promoting the biological delivery of macromolecules in cells; (4) assisting a drug delivery system to successfully cross the dense epithelial and endothelial barriers; (5) combination with imaging in promoting imaging sensitivity and diagnostic efficiency; (6) combination of therapeutic drugs and imaging methods in monitoring the delivery point of drugs in the body, real-time feedback of therapeutic drugs’ in vivo efficacy, and (7) providing new methods for the development of synthetic vaccines. The construction of new nano-targeted drugs is expected to reduce the resistance of NPC to traditional chemotherapy drugs and kill tumor cells more accurately. However, currently, all relevant studies are only in the laboratory stage, and the results have not been transformed into clinical ones. Further research is needed to determine the ultimate role of nanomaterials in NPC treatment.
Optical Diagnostic Strategies for NPC
Fluorescence Imaging (FLI)
The advent of FLI technology has resulted in unprecedented breakthroughs in cell biology, molecular biology, and biotechnology.Citation28–Citation30 The FLI technology depends mainly on the use of fluorophores. When these fluorophores are bound to a targeted structure of interest, photons emitted from the fluorophore can be utilized to visualize the same.Citation31 The benefits of FLI are that the targeted structures are illuminated, whereas the untargeted regions of the sample have little or no fluorescence, allowing for easy targeting and imaging;Citation32 this is conducive to the extensive application of FLI in NPC. FLI can only simply display cells on the culture dish or slide at the beginning and reveal the complete organisms in the natural state in vivo. However, in vivo fluorescence imaging has technical challenges. Scientists have developed fluorescence imaging probes through continuous research and a combination of organic, inorganic, and biological fluorescent nanoparticles. Therefore, the fields of in vivo fluorescence imaging, imaging strategies, and reporter gene technology have achieved great progress in recent years.Citation33–Citation37 In 2020, Zhu et al developed imaging or therapeutic agents for NPC by targeting specific molecules to identify the tumor-targeting ability of ZLMP2A-N affibodies (ZLMP2A-N85, ZLMP2A-N110, and ZLMP2A-N252) in tumor-bearing nude mice. As shown in , they focused on the biodistribution and imaging characteristics of DyLight 755-labelled affibody molecules in vivo using FLI. The results indicated that high-contrast fluorescence signals of the tumor locations were observed in the CNE-2Z xenograft models (). As expected, no visible fluorescence signal was observed at the tumor location in A375 xenografts (). In conclusion, these findings provide strong evidence that the three novel EBV LMP2A N-terminal domain-binding affibody molecules have great potential for utilization and development as molecular probes for both molecular imaging and targeted therapy of EBV-related NPC.Citation38
Figure 2 Tumor’s imaging in model mice by using fluorescence-labelled affibody molecules. Tumor-bearing nude mice (arrows) were generated with cell lines CNE-2Z (A), and A375 (B). NIR-based imaging was performed at different time points p.i. with DyLight 755-labelled ZLMP2A-N affibodies. DyLight 755-labelled ZWT affibody was used as a negative control. Adapted from Zhu J, Kamara S, Cen D et al. Generation of novel affibody molecules targeting the EBV LMP2A N-terminal domain with inhibiting effects on the proliferation of nasopharyngeal carcinoma cells. Cell death \and disease. 2020, 11(4): 1–16. Creative Commons license and disclaimer available from: http://creativecommons.org/licenses/by/4.0/legalcode.Citation38
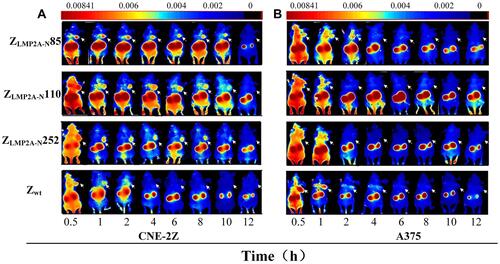
Notably, the combination of nanoprobes and FLI technology has great potential for noninvasive molecular imaging for detecting diseases, monitoring target-specific drug delivery, and evaluating therapeutic efficacy. Further advances in molecular probes in combination with FLI modalities and targeting of novel disease-related biomarkers will offer powerful image-guided therapeutic tools for the diagnosis, prognosis, and treatment of diseases in different clinical settings.
Photoacoustic Imaging (PAI)
PAI is a forthcoming biomedical imaging modality that offers the advantages of non-invasiveness, high optical contrast, fine spatial resolution, and deep tissue penetration, and widely utilized in clinical tumor diagnosis.Citation39,Citation40
In recent years, researchers have continuously overcome the limitations of traditional PAI to develop high-resolution and high-precision imaging systems, such as photoacoustic endoscopy and ultra-high-resolution imaging systems, and to enhance the effect of photoacoustic imaging by studying contrast agents. The combination of photoacoustic imaging and nanoparticles was found to provide high-contrast and high-resolution images for the early detection of NPC and also effectively monitor the treatment process and treatment course of NPC.Citation41,Citation42 In 2018, Ding et al designed an exosome-like nanozyme vesicle for in vivo H2O2-responsive NPC to solve the problem of few tumors catalytic/responsive PAI strategies.Citation43 As shown in , the PA signal at the tumor site was detected after the injection of both FA-RM:GQDzyme/ABTS and RM:GQDzyme/ABTS; the signal was clearly visualized and it gradually increased in the CNE-2 tumor. By comparing the signal results of the two compounds, FA modification was confirmed to enhance NPC targeting ability. In vivo animal experiments demonstrated that this exosome-like nanozyme vesicle effectively accumulated in NPC and that it selectively triggered catalytic PAI. Together, these results demonstrate that the GQDzyme/ABTS-based exosome-like nanozyme vesicle is an ideal nanoplatform for developing deep-tissue tumor-targeted catalytic PAI in vivo.
Figure 3 In vivo H2O2-responsive photoacoustic imaging (PAI) for nasopharyngeal carcinoma detection with exosome-like nanozyme vesicles. (A) PAI of the tumor in nude mice before and 2, 4, and 8 h intravenous post injections of nanozyme vesicles. (B) Average photoacoustic signal intensity of the tumor tissues of panel a. Error bars represented the standard deviations (n = 3). **p < 0.01, ***p < 0.001, ****p < 0.0001. Reprinted from Ding H, Cai Y, Gao L et al. Exosome-like nanozyme vesicles for H2O2-responsive catalytic photoacoustic imaging of xenograft nasopharyngeal carcinoma. Nano Lett. 2018, 19(1): 203–209. Copyright (2018) American Chemical Society.Citation43
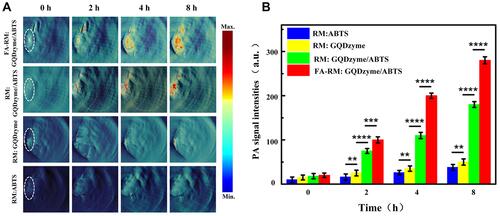
Raman Spectroscopy Imaging (RSI)
The RSI is a unique optical analytical tool with many advantages over conventional optical detection techniques, such as high sensitivity, low water interference, and high signal-to-noise ratio. With the improvement of detection technology and the reduction of the requirements for tested samples, RSI has been widely used in physics, chemistry, medicine, industry, and other fields, and an increasing number of researchers are focusing on the same. Especially in recent years, the emergence of laser Raman probes has brought good news to the research on early NPC detection.Citation44–Citation47 Prior to 2011, researchers who used RSI to detect saliva, plasma, and other samples of normal people and NPC patients, found that the spectra of samples in the two populations were different, and finally verified that RSI could be used as an imaging technology for the detection of NPC. Furthermore, after persistent exploration, scientists found that RSI combined with nanoprobes improved the detection rate of NPC.Citation48–Citation50 In 2012, Chen et al developed a highly specific and sensitive methodology using 4-MBA labelled Au/Ag core-shell nanoparticles combined with sensitive surface-enhanced Raman scattering (SERS) for rapid analysis of LMP1 expression in NPC tissues.Citation51 The LMP1 of normal and NPC tissue sections was detected by SERS, as shown in . The results indicated a typical SERS spectrum of the NPC tissue antibody. As can be seen in , normal nasopharyngeal epithelial tissue was almost black everywhere except for a few dark red spots, which were most likely due to nonspecific binding of the LMP1 antibody, indicating that there was no expression of LMP1. As shown in , cancer cells appeared yellow and white, indicating a high expression of LMP1 in NPC cells. This result is in good agreement with the expected LMP1 expression patterns in normal and cancer tissues. The results demonstrate the feasibility and promise of developing this new technology as a clinical tool for the differential diagnosis of NPC as well as monitoring the recurrence and progression of patients with the disease. Furthermore, this method has the potential to simultaneously detect multiple antigens in tissue samples.
Figure 4 (A) Representative spectra obtained from the LMP1-SERS probe in nasopharyngeal carcinoma (NPC) tissue (red), normal nasopharyngeal tissue (blue), and glass (black), as well as non-targeted surface-enhanced Raman scattering (SERS) probes in the NPC tissue (green). The SERS was acquired by scanning the laser beam to cover the white boxes in (B and C), where black represents the lowest intensity and white represents the highest intensity. Reproduced from with permission from Dove Medical Press Ltd. Chen Y, Zheng X, Chen G et al. Immunoassay for LMP1 in nasopharyngeal tissue based on surface-enhanced Raman scattering. Int J Nanomed. 2012, 7: 73–82.Citation51
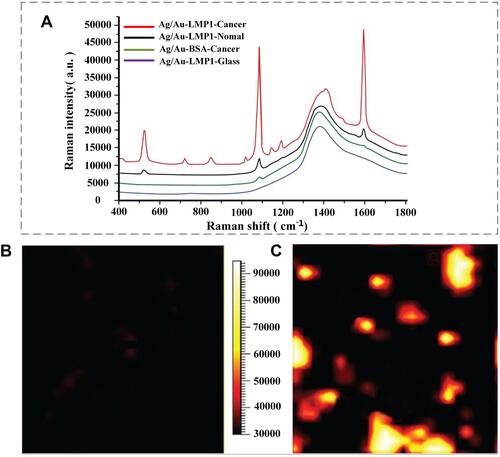
Soon afterwards, researchers found that usage of metal nanoparticles as SERS-active nanostructures and nano-biosensors based on SERS technology combined with multivariate statistical analysis after in-depth research could screen high-throughput NPC cells, identify different cell lines, and distinguish different T stages of NPC.Citation52–Citation54 These studies hence, have a great clinical value.
Optical Treatment Strategies for NPC
Photothermal Therapy (PTT)
PTT is a novel and promising therapeutic strategy. The principle of PTT is to utilize a photosensitizer agent to convert the light photon energy to heat, which can destroy cancer cells.Citation55–Citation57 Compared with traditional therapeutic modalities, PTT has unique advantages in tumor therapy, including minimal invasiveness, high specificity, and precise spatiotemporal selectivity.Citation58,Citation59 In recent decades, the potential application of PTT in treating cancer metastasis has been explored by many research teams.Citation60,Citation61 The results indicate that PTT can directly root out the cancer cells in the primary tumor or local metastasis to fight the initial stage of cancer metastasis and also be combined with current therapeutic methods to eliminate cancer cells in metastatic sites.Citation62–Citation65 PTT can be used alone, guided by multimodal imaging, or combined with currently available therapies to carry out their therapeutic outcomes in the clinic.Citation66–Citation68 To date, PTT or its combination therapy has been investigated in animal models with lung, bone, or lymph metastasis of many types of cancers and has shown promising therapeutic efficiency.
Recently, gold nanomaterials have been widely used in cancer therapy, owing to their unique advantages. Since 2006, to find methods with better targeted therapeutic effects on NPC, many researchers have used a combination of different gold nanorod compounds and PTT to selectively kill NPC cells, and the results have achieved good curative effect.Citation69–Citation71 In 2021, Liu et al synthesized a polyethylene glycol-coated ultrasmall superparamagnetic iron oxide nanoparticle-coupled sialyl Lewis X (USPIO-PEG-sLex) nanotheranostic platform using high-temperature pyrolysis.Citation14 As shown in , the results indicated that the temperature of the tumor tissue in the tail vein injection group was higher than that in the control group ( and ). Changes in tumor pre- and post-treatment between the tail vein injection and control groups were also recorded accordingly (). The results revealed that the tumor volume in the tail vein injection group was smaller than that in the control group after five treatments. Therefore, USPIO-PEG-sLex nanoparticles can significantly inhibit tumor progression and serve as a nanothermal platform for PTT.
Figure 5 Tail vein injection group (A) and Control group (B), “+” is the highest temperature area which is measured by the NIR imager. (a) Tumor before treatment, (b) Tumor after five treatments, (c) The maximum temperature is 33.8 °C and 34.0 °C (non-tumor tissue temperature) before treatment, (d) The maximum temperature after 10 min of treatment reached 43.7 °C (injection group) and 41.8 °C (Control group) 10 min (tumor tissue temperature). (C) Tail vein injection group and Control group. (The first column was the tumor pre-treatment, and the last column was the tumor after five photothermal therapy (PTT) sessions. Reproduced from Liu Q, Liu L, Mo C et al. Polyethylene glycol-coated ultrasmall superparamagnetic iron oxide nanoparticles-coupled sialyl Lewis X nanotheranostic platform for nasopharyngeal carcinoma imaging and photothermal therapy. J. Nanobiotechnology. 2021, 19(1): 1–14. Creative Commons license and disclaimer available from: http://creativecommons.org/licenses/by/4.0/legalcode.Citation14
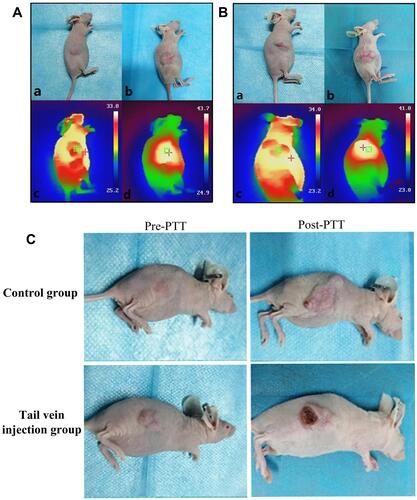
Photodynamic Therapy (PDT)
Photodynamic therapy (PDT) is photochemistry-based modality wherein the photosensitizing agent can be selectively centralized in the malignant tissue and excited by laser light, and the subsequent photodynamic reaction could generate cytotoxic substances and lead to cancer cells death while sparing surrounding healthy tissue cells. Successful treatment of NPC with PDT was reported in clinical trials prior to the 1890s.Citation72–Citation76 In the early 20th century, to optimize the use of PDT in the treatment of NPC and obtain better curative effects, scientific researchers carried out more in-depth research on the mechanism of PDT killing NPC cells, developed excellent photosensitizers, and achieved countless gratifying results.Citation77–Citation81 The efficacy of PDT in the treatment of NPC has further improved with the maturity of nano-photosensitizers. For example, in 2018, Akbarzadeh et al discovered that 5-aminolevulinic acid and folic acid-conjugated bismuth oxide nanoparticles could significantly increase the cell-killing effect of PDT.Citation82 However, NPC therapy is usually associated with various side effects, since its treatment options have not yet been completely resolved. Combination therapy of near-infrared (NIR) laser light-mediated PTT and PDT could be a promising strategy for NPC treatment in the future. In 2018, Ryu et al fabricated PCM-based nanoparticles containing Ce6 and NDs and combined PTT and PDT for the treatment of NPC.Citation83 These results successfully demonstrated the synergistic effect of PDT and PTT in NPC therapy. In 2021, Naveen et al developed acidic surface-charge transformable nanoclusters (NCs) composed of indocyanine green (ICG), Fe3O4, and palmitoyl ascorbic acid with pH-responsive PEG-b-PAEMAPDMA for enhanced synergistic PDT/PTT.Citation84 The results revealed that NCs could be used as “all in one” nanotheranostic agent for enhanced PDT/PTT of NPC therapy. Zhong et al successfully synthesized an efficient multifunctional nanocluster (NPs FA-PEG/CBT@ SPION-ICG) and enhanced the targeting and aggregation efficiency for PTT and PDT.Citation85 As displayed in , the outcomes indicated that FA-PEG/CBT@SPION-ICG combined with PTT/PDT had a good therapeutic effect on nude mice bearing HNE-1 tumors compared with the control-treated groups. Furthermore, FA-PEG/CBT@SPION-ICG is biologically safe and is expected to be a complementary treatment model for HNE-1 NPC.
Figure 6 Thermal imaging of the mice after treatment with different formulations with NIR or MHT (A). Imaging was performed before and on day 2 after administering the different treatments (B). Reproduced from Zhong Y, Bejjanki NK, Miao X et al. Synthesis and Photothermal Effects of Intracellular Aggregating Nanodrugs Targeting Nasopharyngeal Carcinoma. Frontiers in bioengineering and biotechnology. 2021: 847. Creative Commons license and disclaimer available from: http://creativecommons.org/licenses/by/4.0/legalcode.Citation85
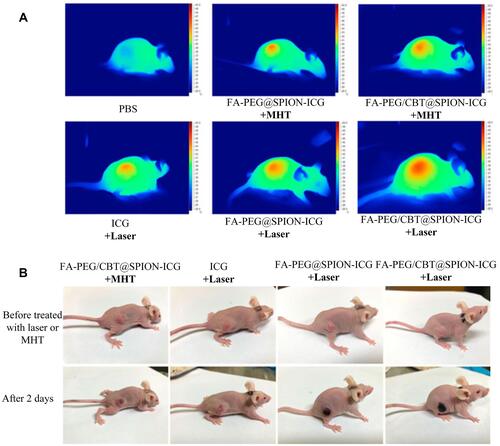
Discussion and Conclusion
As is well known, the effectiveness of NPC treatment depends on optimum usage of the best imaging method and the appropriate treatment strategy. However, common clinical imaging methods, such as radiographs, CT, MRI, and PET, have obvious limitations, such as radiation damage, insensitivity, and non-targeting. Radiotherapy and chemotherapy are the primary treatment methods for NPC. However, these methods have many serious irreversible complications. Notably, optical imaging technology (FLI, RSI, PAI) and the joint treatment strategy have been further developed and has recently received considerable attention in bio-related or clinical imaging fields especially in NPC. It now facilitates an optical imaging platform due to the advantage of the significant advancement of exogenous imaging agents (nanoparticles), which is imperative for contemporary theragnosis, a combination of precise diagnosis and well-timed therapy. Therefore, scientists have committed to the goal of simultaneous diagnosis, noninvasive monitoring, and accurate treatment of NPC. In recent decades, Chinese and international research teams are committed to improving the efficacy of PDT, PTT, PDT/chemotherapy, PTT/chemotherapy, and PDT/PTT/chemotherapy on NPC through the joint treatment strategy of optical imaging technology (FLI, RSI, PAI) and PDT, PTT/chemotherapy, in order to overcome the inherent shortcomings of a single treatment mode, to realize noninvasive diagnosis, and provide ideal synergistic anti-cancer effects. Meanwhile, to lay a solid or stable foundation for the further development and optimization of optical endoscopy technology and the improvement of its post-processing procedure. In addition, it provides a basis and direction for further optimization of nano-targeting platform and combination immunotherapy and other combination therapy research.
This review systematically introduces the latest developments and progress in phototheranostics strategies of NPC-based nanomaterials for the rational incorporation of photoimaging with phototherapy, including FLI, RSI, PAI, PDT, PTT, and PDT/PTT. To provide a basis and reference for the rapid development of nanotechnology and the promotion of optical techniques, it will be conducive to advance molecular imaging, improve the targeting property of the nanotheranostic platform, and facilitate the preclinical investigation of biological processes in living subjects in the future. The therapeutic effect on NPC has greatly improved in recent times. Phototheranostics strategies not only provide direction for the prospective development of diagnosis and treatment technology of nasopharyngeal carcinoma, but also will become new strategies for the treatment of NPC.
Acknowledgments
This research is supported by the Guangzhou Science and Technology Plan Project (202102020140), Guangdong Basic and Applied Basic Research Foundation (2020A1515011104).
Disclosure
The authors declare that there are no conflicts of interest related to this article.
References
- Siegel RL, Miller KD, Fuchs HE, Jemal A. Cancer statistics, 2021. CA Cancer J Clin. 2021;71(1):7–33. doi:10.3322/caac.21654
- Farhat K, Hassen E, Bouzgarrou N, Gabbouj S, Bouaouina N, Chouchane L. Functional IL-18 promoter gene polymorphisms in Tunisian nasopharyngeal carcinoma patients. Cytokine. 2008;5:4–6. doi:10.1016/j.cyto.2008.05.004
- Wang D, Gao L, Liu X, Yuan C, Wang G. Improved antitumor effect of ionizing radiation in combination with rapamycin for treating nasopharyngeal carcinoma. Oncol Lett. 2017;14(1):1105–1108. doi:10.3892/ol.2017.6208
- Khan RZ, Iqbal A, Saqulain G. Diagnostic accuracy of fiber optic laryngoscopy. J Islamabad Med\Dental College. 2021;10(2):101–104. doi:10.35787/jimdc
- Liu M, Lin J, Qiu S, et al. Label-free classification of a nasopharyngeal carcinoma tissue test at different stages based on Raman spectroscopy. J Aoac Int. 2017;100(2):429–433. doi:10.5740/jaoacint.16-0191
- King AD. MR imaging of nasopharyngeal carcinoma. Magn Reson Imaging Clin. 2022;30(1):19–33. doi:10.1016/j.mric.2021.06.015
- Ng S, Chan S, Yen T, et al. Staging of untreated nasopharyngeal carcinoma with PET/CT: comparison with conventional imaging work-up. Eur J Nucl Med Mol I. 2009;36(1):12–22. doi:10.1007/s00259-009-1080-6
- Razek A, King A. MRI and CT of nasopharyngeal carcinoma. Am J Roentgenol. 2012;198(1):11–18. doi:10.2214/AJR.11.6954
- Wu L, Liu F, Liu S, Xu X, Liu Z, Sun X. Perfluorocarbons-based 19F magnetic resonance imaging in biomedicine. Int J Nanomed. 2020;15:7377–7395. doi:10.2147/IJN.S255084
- He X, Wang K, Cheng Z. In vivo near‐infrared fluorescence imaging of cancer with nanoparticle-based probes. WIRES NANOMED NANOBI. 2010;2(4):349–366. doi:10.1002/wnan.85
- Lee HM, Okuda KS, Gonz A, Lez F, Patel V. Current perspectives on nasopharyngeal carcinoma. Human Cell Trans. 2019;11–34. doi:10.1007/978-3-030-22254-3-2
- Zhang W, Zhang Y, Ke S, et al. Multi-modality imaging to determine the cellular heterogeneity of nasopharyngeal carcinoma components. Oncotarget. 2014;5(8):2221. doi:10.18632/oncotarget.1894
- Huang P, Bao L, Zhang C, et al. Folic acid-conjugated silica-modified gold nanorods for X-ray/CT imaging-guided dual-mode radiation and photo-thermal therapy. Biomaterials. 2011;32(36):9796–9809. doi:10.1016/j.biomaterials.2011.08.086
- Liu Q, Liu L, Mo C, et al. Polyethylene glycol-coated ultrasmall superparamagnetic iron oxide nanoparticles-coupled sialyl Lewis X nanotheranostic platform for nasopharyngeal carcinoma imaging and photothermal therapy. J Nanobiotechnol. 2021;19(1):1–14. doi:10.1186/s12951-021-00918-0
- Liu J, Li Q, Zhang J, et al. Safe and effective reversal of cancer multidrug resistance using sericin-coated mesoporous silica nanoparticles for lysosome-targeting delivery in mice. Small. 2017;13(9):1602567. doi:10.1002/smll.201602567
- Shu C, Zheng W, Lin K, et al. Label-free follow-up surveying of post-treatment efficacy and recurrence in nasopharyngeal carcinoma patients with fiberoptic Raman endoscopy. Anal Chem. 2021;93(4):2053–2061. doi:10.1021/acs.analchem.0c03778
- Wu RWK, Chu ESM, Yuen JWM, et al. Comparative study of FosPeg® photodynamic effect on nasopharyngeal carcinoma cells in 2D and 3D models. J Photochem Photobiol B. 2020;210:111987. doi:10.1016/j.jphotobiol.2020.111987
- Zhou B, Ma Y, Li L, et al. Pheophorbide co-encapsulated with cisplatin in folate-decorated PLGA nanoparticles to treat nasopharyngeal carcinoma: combination of chemotherapy and photodynamic therapy. Colloids Surf B Biointerfaces. 2021;208:12100. doi:10.1016/j.colsurfb.2021.112100
- Wu RWK, Chu ESM, Huang Z. P20-1 enhanced foslip® photodynamic therapy anti-tumor effect in nasopharyngeal carcinoma via fractionated light irradiation. Ann Oncol. 2021;32:S339. doi:10.1016/j.annonc.2021.05.709
- Wu N, Tu Y, Fan G, et al. Enhanced photodynamic therapy/photothermotherapy for nasopharyngeal carcinoma via a tumour microenvironment-responsive self-oxygenated drug delivery system. Asian J Pharm Sci. 2022;17:253–267. doi:10.1016/j.ajps.2022.01.002
- Hu J, Zhang X, Wen Z, et al. Asn-gly-arg-modified polydopamine-coated nanoparticles for dual-targeting therapy of brain glioma in rats. Oncotarget. 2016;7(45):73681. doi:10.18632/oncotarget.12047
- Chen X, Liu Z, Parker SG, et al. Light-induced hydrogel based on tumor-targeting mesoporous silica nanoparticles as a theranostic platform for sustained cancer treatment. ACS Appl Mater Interfaces. 2016;8(25):15857–15863. doi:10.1021/acsami.6b02562
- Shi J, Kantoff PW, Wooster R, et al. Cancer nanomedicine: progress, challenges and opportunities. Nat Rev Cancer. 2017;17(1):20–37. doi:10.1038/nrc.2016.108
- Han X, Xu K, Taratula O, Farsad K. Applications of nanoparticles in biomedical imaging. Nanoscale. 2019;11(3):799–819. doi:10.1039/C8NR07769J
- Li Volsi A, Scialabba C, Vetri V, Cavallaro G, Licciardi M, Giammona G. Near-infrared light responsive folate targeted gold nanorods for combined photothermal-chemotherapy of osteosarcoma. ACS Appl Mater Interfaces. 2017;9(16):14453–14469. doi:10.1021/acsami.7b03711
- Thakur S, Saini RV, Singh P, Raizada P, Thakur VK, Saini AK. Nanoparticles as an emerging tool to alter the gene expression: preparation and conjugation methods. Mater Today Chem. 2020;17:100295. doi:10.1016/j.mtchem.2020.100295
- Li XD, Gao JY, Yang Y, et al. Nanomaterials in the application of tumor vaccines: advantages and disadvantages. Oncotargets Ther. 2013;6:629. doi:10.2147/OTT.S41902
- Lichtman JW, Conchello JEA. Fluorescence microscopy. Nat Methods. 2005;2(12):910–919. doi:10.1038/nmeth817
- Lichtenegger A, Gesperger J, Kiesel B, et al. Revealing brain pathologies with multimodal visible light optical coherence microscopy and fluorescence imaging. J Biomed Optics. 2019;24(6):066010. doi:10.1117/1.JBO.24.6.066010
- Llopis PM, Senft RA, Ross-Elliott TJ, et al. Best practices and tools for reporting reproducible fluorescence microscopy methods. Nat Methods. 2021:1–14. doi:10.1038/s41592-021-01156-w
- Drummen GP. Fluorescent probes and fluorescence (microscopy) techniques—illuminating biological and biomedical research. Molecules. 2012;17(12):14067–14090. doi:10.3390/molecules171214067
- Reynaud EG, Kržič U, Greger K, Stelzer,EHK. Light sheet-based fluorescence microscopy: more dimensions, more photons, and less photodamage. HFSP J. 2008;2(5):266–275. doi:10.2976/1.2974980
- Lin LS, Yang FW, Xie SS. Extracting autofluorescence spectral features for diagnosis of nasopharyngeal carcinoma. Laser Phys. 2012;22(9):1431–1434. doi:10.1134/S1054660X12090095
- Yow C, Chen JY, Mak NK, Cheung NH, Leung A. Cellular uptake, subcellular localization and photodamaging effect of temoporfin (mTHPC) in nasopharyngeal carcinoma cells: comparison with hematoporphyrin derivative. Cancer Lett. 2000;157(2):123–131. doi:10.1016/S0304-3835(00)00453-5
- Zhang P, Wang P, Yan L, Liu L. Synthesis of gold nanoparticles with Solanum xanthocarpum extract and their in vitro anticancer potential on nasopharyngeal carcinoma cells. Int J Nanomed. 2018;13:7047. doi:10.2147/IJN.S180138
- Bae PK, Jung J, Lim SJ, Kim D, Kim S, Chung BH. Bimodal perfluorocarbon nanoemulsions for nasopharyngeal carcinoma targeting. Mol Imaging Biol. 2013;4(15):401–410. doi:10.1007/s11307-013-0622-2
- Ramaswamy B, Manivasager V, Chin WW, Soo KC, Olivo M. Photodynamic diagnosis of a human nasopharyngeal carcinoma xenograft model using the novel chlorin e6 photosensitizer Fotolon®. Int J Oncol. 2005;26(6):1501–1506. doi:10.3892/ijo.26.6.1501
- Zhu J, Kamara S, Cen D, et al. Generation of novel affibody molecules targeting the EBV LMP2A N-terminal domain with inhibiting effects on the proliferation of nasopharyngeal carcinoma cells. Cell Death Dis. 2020;11(4):1–16. doi:10.1038/s41419-020-2410-7
- Mallidi S, Luke GP, Emelianov S. Photoacoustic imaging in cancer detection, diagnosis, and treatment guidance. Trends Biotechnol. 2011;29(5):213–221. doi:10.1016/j.tibtech.2011.01.006
- Jung D, Park S, Lee C, et al. Recent progress on near-infrared photoacoustic imaging: imaging modality and organic semiconducting agents. Polymers. 2019;11(10):1693. doi:10.3390/polym11101693
- Dong W, Tang ZL, Wu YB. Application of Anti-EGFR/Au conjugates for photoacoustic molecular imaging of nasopharyngeal carcinoma cells. World Sci. 2016;450–458. doi:10.1142/9789814719810-0059
- Ding H, Cai Y, Chen J, et al. Cryodesiccation-driven crystallization preparation approach for zinc (II)-phthalocyanine nanodots in cancer photodynamic therapy and photoacoustic imaging. Microchim Acta. 2019;186(4). doi:10.1007/s00604-019-3286-z
- Ding H, Cai Y, Gao L, et al. Exosome-like nanozyme vesicles for H2O2-responsive catalytic photoacoustic imaging of xenograft nasopharyngeal carcinoma. Nano Lett. 2018;19(1):203–209. doi:10.1021/acs.nanolett.8b03709
- Qiu S, Xu Y, Huang L, et al. Non-invasive detection of nasopharyngeal carcinoma using saliva surface-enhanced raman spectroscopy. Oncol Lett. 2016;11(1):884–890. doi:10.3892/ol.2015.3969
- Kimura-Suda H, Ito T. Bone quality characteristics obtained by Fourier transform infrared and raman spectroscopic imaging. J Oral Biosci. 2017;591:142–145. doi:10.1016/j.job.2017.04.002
- Lan M, Hsu Y, Lan M, Chen J, Lu Y. Polyethylene glycol-coated graphene oxide loaded with erlotinib as an effective therapeutic agent for treating nasopharyngeal cancer cells. Int J Nanomed. 2020;15:7569. doi:10.2147/IJN.S265437
- Zuvela P, Lin K, Shu C, et al. Fiber-optic raman spectroscopy with nature-inspired genetic algorithms enhances real-time in vivo detection and diagnosis of nasopharyngeal carcinoma. Anal Chem. 2019;91(13):8101–8108. doi:10.1021/acs.analchem.9b00173
- Feng S, Lin J, Cheng M, et al. Gold nanoparticle based surface-enhanced raman scattering spectroscopy of cancerous and normal nasopharyngeal tissues under near-infrared laser excitation. Appl Spectrosc. 2009;63(10):1089–1094. doi:10.1366/000370209789553291
- Feng S, Chen R, Lin J, et al. Nasopharyngeal cancer detection based on blood plasma surface-enhanced raman spectroscopy and multivariate analysis. Biosens Bioelectron. 2010;25:2414–2419. doi:10.1016/j.bios.2010.03.033
- Yu Y, Lin J, Wu Y, et al. Optimizing electroporation assisted silver nanoparticle delivery into living C666 cells for surface-enhanced raman spectroscopy. Spectroscopy-Us. 2011;25(1):13–21. doi:10.3233/SPE-2010-0493
- Chen Y, Zheng X, Chen G, et al. Immunoassay for LMP1 in nasopharyngeal tissue based on surface-enhanced Raman scattering. Int J Nanomed. 2012;7:73. doi:10.2147/IJN.S26854
- Lin D, Pan J, Huang H, et al. Label-free blood plasma test based on surface-enhanced Raman scattering for tumor stages detection in nasopharyngeal cancer. Sci Rep-Uk. 2014;4(1):1–8. doi:10.1038/srep04751
- Feng S, Li Z, Chen G, et al. Ultrasound-mediated method for rapid delivery of nano-particles into cells for intracellular surface-enhanced Raman spectroscopy and cancer cell screening. Nanotechnology. 2015;26(6):065101. doi:10.1088/0957-4484/26/6/065101
- Chen W, Xu S, Wang X, et al. Single cell detection using intracellularly-grown-Au-nanoparticles based surface-enhanced Raman scattering spectroscopy for nasopharyngeal cell lines classification. Anal Methods-Uk. 2021;13:3147–3153. doi:10.1039/D1AY00554E
- Shanmugam V, Selvakumar S, Yeh CS. Near-infrared light-responsive nanomaterials in cancer therapeutics. Chem Soc Rev. 2014;43:6254–6287. doi:10.1039/C4CS00011K
- Alkilany AM, Thompson LB, Boulos SP, Sisco PN, Murphy CJ. Gold nanorods: their potential for photothermal therapeutics and drug delivery, tempered by the complexity of their biological interactions. Adv Drug Deliver Rev. 2012;64:190–199. doi:10.1016/j.addr.2011.03.005
- Zhang Z, Wang J, Chen C. Near-infrared light-mediated nanoplatforms for cancer thermo-chemotherapy and optical imaging. Adv Mater. 2013;25:3869–3880. doi:10.1002/adma.201301890
- Hu S-H, Fang R-H, Chen Y-W, Liao B-J, Chen IW, Chen S-Y. Photoresponsive protein-graphene-protein hybrid capsules with dual targeted heat-triggered drug delivery approach for enhanced tumor therapy. Adv Funct Mater. 2014;24:4144–4155. doi:10.1002/adfm.201400080
- Ma Y, Liang X, Tong S, Bao G, Ren Q, Dai Z. Gold nanoshell nanomicelles for potential magnetic resonance imaging, light-triggered drug release, and photothermal therapy. Adv Funct Mater. 2013;23:815–822. doi:10.1002/adfm.201201663
- Zhang Z, Wang J, Nie X, et al. Near infrared laser-induced targeted cancer therapy using thermoresponsive polymer encapsulated gold nanorods. J Am Chem Soc. 2014;136:7317–7326. doi:10.1021/ja412735p
- Okuno T, Kato S, Hatakeyama Y, et al. Photothermal therapy of tumors in lymph nodes using gold nanorods and near-infrared laser light. J Control Release. 2013;172:879–884. doi:10.1007/s12274-015-0884-x
- He X, Bao X, Cao H, et al. Tumor-penetrating nanotherapeutics loading a near-infrared probe inhibit growth and metastasis of breast cancer. Adv Funct Mater. 2015;25:2831–2839. doi:10.1002/adfm.201500772
- Wang D, Xu Z, Yu H, et al. Treatment of metastatic breast cancer by combination of chemotherapy and photothermal ablation using doxorubicin-loaded DNA wrapped gold nanorods. Biomaterials. 2014;35:8374–8384. doi:10.1016/j.biomaterials.2014.05.094
- Zhou F, Li X, Naylor MF, et al. InCVAX–a novel strategy for treatment of late-stage, metastatic cancers through photoimmunotherapy induced tumor-specific immunity. Cancer Lett. 2015;359:169–177. doi:10.1016/j.canlet.2015.01.029
- Chen Q, Liang C, Wang C, Liu Z. An imagable and photothermal “Abraxane-Like” nanodrug for combination cancer therapy to treat subcutaneous and metastatic breast tumors. Adv Mater. 2015;27:903–910. doi:10.1002/adma.201404308
- Cai X, Jia X, Gao W, et al. A versatile nanotheranostic agent for efficient dual-mode imaging guided synergistic chemo-thermal tumor therapy. Adv Funct Mater. 2015;25:2520–2529. doi:10.1002/adfm.201403991
- Wang Y, Yang T, Ke H, et al. Smart albumin-biomineralized nanocomposites for multimodal imaging and photothermal tumor ablation. Adv Mater. 2015;27:3874–3882. doi:10.1002/adma.201500229
- Chen Q, Wang C, Zhan Z, et al. Near-infrared dye bound albumin with separated imaging and therapy wavelength channels for imaging-guided photothermal therapy. Biomaterials. 2014;35:8206–8214. doi:10.1016/j.biomaterials.2014.06.013
- Shao J, Tang J, Ji J, et al. Therapy effects of gold nanorods on the CNE-1 nasopharyngeal carcinoma cell line. Drug Des Devel Ther. 2012;6:297. doi:10.2147/DDDT.S36706
- Zhou W, Shao J, Jin Q, Wei Q, Tang J, Ji J. Zwitterionic phosphorylcholine as a better ligand for gold nanorods cell uptake and selective photothermal ablation of cancer cells. Chem Commun. 2010;46(9):1479–1481. doi:10.1039/B915125G
- Movahedi MM, Mehdizadeh A, Koosha F, et al. Investigating the photo-thermo-radiosensitization effects of folate-conjugated gold nanorods on KB nasopharyngeal carcinoma cells. Photodiagn Photodyn. 2018;24:324–331. doi:10.1016/j.pdpdt.2018.10.016
- Gondivkar SM, Gadbail AR, Choudhary MG, et al. Photodynamic treatment outcomes of potentially-malignant lesions and malignancies of the head and neck region: a systematic review. J inv Clin Dent. 2018;9(1):e12270. doi:10.1111/jicd.12270
- Civantos FJ, Karakullukcu B, Biel M, et al. A review of photodynamic therapy for neoplasms of the head and neck. Adv Ther. 2018;35(3):324–340. doi:10.1007/s12325-018-0659-3
- Wildeman M, Nyst HJ, Karakullukcu B, et al. Photodynamic therapy in the therapy for recurrent/persistent nasopharyngeal cancer. Head Neck Oncol. 2009;1(1):1–6. doi:10.1186/1758-3284-1-40
- Stoker SD, Indrasari SR, Herdini C, et al. Photodynamic therapy as salvage therapy for patients with nasopharyngeal carcinoma experiencing local failures following definitive radiotherapy. Photodiagn Photodyn. 2015;12(3):519–525. doi:10.1016/j.pdpdt.2015.04.005
- Wu RWK, Chu ESM, Yow CMN, et al. Photodynamic effects on nasopharyngeal carcinoma (NPC) cells with 5-aminolevulinic acid or its hexyl ester. Cancer Lett. 2006;242(1):112–119. doi:10.1016/j.canlet.2005.10.048
- Li B, Chen Z, Liu L, et al. Differences in sensitivity to HMME-mediated photodynamic therapy between EBV+C666-1 and EBV-CNE2 cells. Photodiagn Photodyn. 2010;7(3):204–209. doi:10.1016/j.pdpdt.2010.05.001
- Zhou Q, Olivo M, Lye KYK, Moore S, Sharma A, Chowbay B. Enhancing the therapeutic responsiveness of photodynamic therapy with the antiangiogenic agents SU5416 and SU6668 in murine nasopharyngeal carcinoma models. Cancer Chemoth Pharm. 2005;56(6):569–577. doi:10.1007/s00280-005-1017-0
- Yee KKL, Soo KC, Olivo M. Anti-angiogenic effects of Hypericin-photodynamic therapy in combination with Celebrex® in the treatment of human nasopharyngeal carcinoma. Int J Mol Med. 2005;16(6):993–1002. doi:10.3892/ijmm.16.6.993
- Li L, Luo R, Liao W, Zhang M, Luo Y, Miao J. Clinical study of Photofrin photodynamic therapy for the treatment of relapse nasopharyngeal carcinoma. Photodiagn Photodyn. 2006;3(4):266–271. doi:10.1016/j.pdpdt.2006.09.004
- Bhuvaneswari R, Gan YY-Y, Yee KKL, Soo KC, Olivo M. Effect of hypericin-mediated photodynamic therapy on the expression of vascular endothelial growth factor in human nasopharyngeal carcinoma. Int J Mol Med. 2007;20(4):421–428. doi:10.3892/ijmm.20.4.421
- Akbarzadeh F, Khoshgard K, Arkan E, Hosseinzadeh L, Hemati Azandaryani A. Evaluating the photodynamic therapy efficacy using 5-aminolevulinic acid and folic acid-conjugated bismuth oxide nanoparticles on human nasopharyngeal carcinoma cell line. Artif Cells, Nanomed Biotechnol. 2018;46(sup3):S514–S523. doi:10.1080/21691401.2018.1501376
- Ryu T, Baek S, Kang R, Jeong K, Jun D, Choi S. Photodynamic and photothermal tumor therapy using phase-change material nanoparticles containing chlorin e6 and nanodiamonds. J Control Release. 2018;270:237–245. doi:10.1016/j.jconrel.2017.12.008
- Bejjanki NK, Zhong Y, Liu J, et al. Surface charge transition nano-theranostics based on ultra-small Fe3O4 nanoparticles for enhanced photodynamic and photothermal therapy against nasopharyngeal carcinoma. Biochem Bioph Res Co. 2021;557:240–246. doi:10.1016/j.bbrc.2021.03.168
- Zhong Y, Bejjanki NK, Miao X, et al. Synthesis and photothermal effects of intracellular aggregating nanodrugs targeting nasopharyngeal carcinoma. Front Bioeng biotechnol. 2021:847. doi:10.3389/fbioe.2021.730925
- Ulaner GA, Sobol NB, O'Donoghue JA, et al. CD38-targeted Immuno-PET of Multiple Myeloma: From Xenograft Models to First-in-Human Imaging. Radiology. 2020:295(3).
- Chen M, Tang S, Guo Z, et al. Core–Shell Pd@Au Nanoplates as Theranostic Agents for In-Vivo Photoacoustic Imaging, CT Imaging, and Photothermal Therapy. Advanced Materials. 2014;26(48):8210-8216.
- Lin K, Zheng W, Lim CM, Huang Z. Real-time In vivo Diagnosis of Nasopharyngeal Carcinoma Using Rapid Fiber-Optic Raman Spectroscopy. Theranostics. 2017;7(14):3517-3526.
- Qiu T, Lan Y, Wei Z, et al. In vivo Multi-scale Photoacoustic Imaging Guided Photothermal Therapy of Cervical Cancer based on Customized Laser System and Targeted Nanoparticles. Int J Nanomedicine. 2021;16:2879-2896.
- Zhang H, Gao X, Xu K, et al. Spatiotemporally Synchronous Oxygen Self-Supply and Reactive Oxygen Species Production on Z-Scheme Heterostructures for Hypoxic Tumor Therapy. Advanced Materials. 2020;32(11):1908109.
- Wang Q, Dai Y, Xu J, et al. All-in-One Phototheranostics: Single Laser Triggers NIR-II Fluorescence/Photoacoustic Imaging Guided Photothermal/Photodynamic/Chemo Combination Therapy. Advanced Functional Materials. 2019;29(31):1901480.