Abstract
Chronic wound caused by diabetes is an important cause of disability and seriously affects the quality of life of patients. Therefore, it is of great clinical significance to develop a wound dressing that can accelerate the healing of diabetic wounds. Nanoparticles have great advantages in promoting diabetic wound healing due to their antibacterial properties, low cytotoxicity, good biocompatibility and drug delivery ability. Adding nanoparticles to the dressing matrix and using nanoparticles to deliver drugs and cytokines to promote wound healing has proven to be effective. This review will focus on the effects of diabetes on wound healing, introduce the properties, preparation methods and action mechanism of nanoparticles in wound healing, and describe the effects and application status of various nanoparticle-loaded dressings in diabetes-related chronic wound healing.
Introduction
As a chronic disease characterized by hyperglycemia, diabetes affects 10% adults worldwide.Citation1 About 15% of patients with diabetes experience diabetic limb ulcers, which are the leading cause of hospitalization and amputation.Citation2 The treatment of diabetic ulcer is a complex process, which requires de-trauma, drainage and anti-inflammatory treatment on the basis of blood glucose control. Among them, the treatment of the wound surface plays a very important role in the process of wound healing. Normal wound healing process is a continuous dynamic process, in this process, a variety of cells orderly migrate to the wound site under the change of wound local microenvironment and growth factors to play their functions, successively multiply and secrete synthetic materials such as collagen protein and polysaccharide required for wound healing, and finally complete the wound healing.Citation3,Citation4 Diabetes is based on the changes of blood glucose, and the main pathological changes are microvascular changes, which then lead to nutrient supply disorders, abnormal inflammatory responses and other changes, and ultimately lead to delayed healing or even delayed healing of wounds.Citation5 In addition to strictly controlling blood glucose level, it is also very important to improve wound local microenvironment and provide favorable conditions for wound healing.Citation6
Wound dressing can provide protection to the wound. For a wound dressing, the ideal wound dressing should have good biocompatibility, good water absorption and water retention.Citation7 In addition, the wound dressing should have good air permeability and antibacterial properties to ensure gas exchange in the wound while avoiding infection.Citation7 Besides, the economy of dressings is one of the factors to be considered. In order to meet the needs of wound dressings, hydrogel, electrospinning, biofilm and other forms and types of wound dressings have been developed.Citation8 At the same time, antibiotics, growth factors, cells and other substances are added to wound dressings, and compound wound dressings are constructed to meet the requirements as much as possible.Citation9
Nanoparticle is a kind of material which has been widely used in recent years. Its small diameter endows nanoparticle with more characteristics. Different kinds of nanoparticles have different properties. The antibacterial properties, drug loading capacity, sensitization effect, good biocompatibility and low toxicity of nanoparticles make them very suitable for the preparation and modification of wound dressings.Citation10 Adding nanoparticles to the dressing matrix and using nanoparticles to deliver drugs and cytokines to promote wound healing has proven to be effective.Citation11 This review will introduce the effect of diabetes on wound healing and the properties of ideal dressing from the characteristics of wound healing (). On this basis, the preparation, properties and action mechanism of nanoparticles are introduced. Finally, the effects and applications of nanoparticles in promoting wound healing are described in detail, and some problems and application prospects of nanoparticles in chronic wound healing are discussed.
Characteristics of Skin Wound Healing
Pathophysiological Changes in Normal Skin Wound Healing
Skin is the largest organ of the human body. It isolates the internal environment of the body from the outside world and prevents harmful factors in the external environment from directly entering the body and causing internal environmental disorders. Skin is an important inherent immune barrier of the human body. At the same time, the skin can also perform functions such as excretion, temperature regulation and external stimulation through the abundant hair follicles and nerve endings on its surface.Citation12,Citation13 A tight, intact skin structure is essential for proper skin function. Normal skin tissue consists of the outer epidermis and the inner dermis. The epidermis is divided into five layers, the innermost of which are the basal cells, which are capable of self-proliferation and differentiation. Basal cells complement the number of spinous cells by proliferating and differentiating into spinous cells, and maintain the stability of the number of epidermal cells, thus playing a role of self-renewal.Citation14,Citation15 Dermis is the dense connective tissue located below the epidermis, which is divided into the papillary layer rich in capillaries and the reticular layer mainly composed of collagen fibers.Citation16 The papillary layer provides various nutrients for the epidermis through abundant capillary structure, and a large number of collagen fibers in the reticulated layer interweave into a network structure, providing the skin with toughness and elasticity.Citation17 In the physiological state, the basal cells in the basal layer of the epidermis get nutrients from the capillaries in the dermis, and partly differentiate into spinous cells while proliferating to supplement the number of spinous cells. The outer layers of epidermal cells change over time into more outer layers until the outermost keratinocytes are shed.Citation18
The normal continuous dense structure of skin can protect the body from external harmful stimulation and harmful substances, which plays an important role in the innate immunity of human body.Citation19 However, the protective effect of skin has a certain limit. When external injury factors exceed a certain range, the normal structure of skin will be destroyed, the continuity of skin will be interrupted, and the protective effect on the body will be weakened or disappear.Citation20 Or the normal structure of the skin changes under the influence of diseases and drugs, so that the skin’s resistance to external stimulation and damage is weakened.Citation21 At this time, even if the slight stimulation or damage that can be resisted under normal conditions can lead to the destruction of the skin structure, causing the skin to fail to play its function normally.Citation22 Skin healing is a continuous and complex dynamic process. The healing process can be roughly divided into hemostatic/inflammatory stage, proliferative stage and remodeling stage according to the main changes at different stages.Citation23 After injury, the exposed intravascular subcutaneous matrix attracts platelets from the blood to bind to basement membrane collagen, allowing platelets to adhere and activate. Besides, activated platelets activate endogenous and exogenous clotting cascades by releasing ADP and TXA2, which in turn encourage platelets to aggregate on vascular walls and form platelet thrombus.Citation24 Platelet α particles contain a large number of cytokines and growth factors. When platelet thrombus is degraded, cytokines and growth factors such as IL-1β, TNF-α, FGF and PDGF in platelets are released, attracting neutrophils and macrophages in blood to gather in the damaged area.Citation25 In the early stage of injury, neutrophils first accumulate in the wound area, while phagocytosis and release of ROS, antimicrobial peptides, proteolytic enzymes and other substances to remove necrotic tissue and pathogens. Mast cells and macrophages then arrive at the damaged area to remove remaining cell debris and neutrophils.Citation26
Under normal conditions, the hemostatic/inflammatory stage of the wound lasts about 72–96 hours, the cell debridement is basically completed, and the wound healing begins to enter the proliferative stage.Citation27 During the proliferation stage, the tissue metabolism of the wound changes from catabolism to anabolism. Fibroblasts and keratinocytes proliferate continuously under the stimulation of PFGF, FGF and EGF, and at the same time synthesize large amounts of type III collagen, proteoglycan and fibronectin, providing the skeleton structure for cell migration, proliferation and growth to the damaged area.Citation28 At the same time, endothelial cells migrate to the wound site under the action of VEGF and form a new capillary network to provide nutrients for other cells in the injured area and remove metabolic waste.Citation29 The main changes during wound remodeling were the remodeling and recombination of collagen. Type III collagen is mainly formed during the proliferation stage of injury repair, while type I collagen is the main component in normal skin tissue.Citation30 During the remodeling phase, fibroblasts continuously synthesize type I collagen to reduce the proportion of type III collagen in the wound area.Citation31 In the meanwhile, through the rearrangement of collagen fibers, the parallel collagen structure in the early stage of the wound is transformed into a lattice network structure, improving the strength and toughness of the skin tissue in the injured area, and finally reaching a level close to or consistent with normal skinCitation32,Citation33 ().
Figure 1 Stages of wound healing and pathophysiological changes in different stages.Citation33
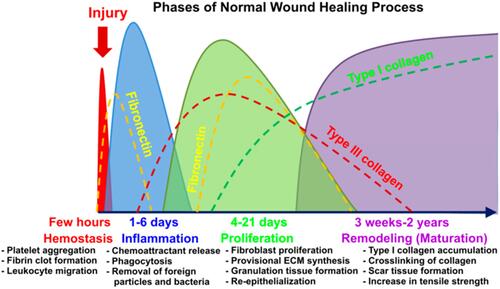
Causes and Pathophysiological Changes of Delayed Skin Wound Healing
Wound healing is a dynamic and continuous complex process, from the emergence of the wound to the complete healing process requires a variety of cells, the joint action of cytokines and growth factors, but also the local wound to maintain a good environment conducive to growthCitation34(). Therefore, any factors that can affect the normal function of cells, interfere with the normal synthesis and secretion of growth factors and affect the local microenvironment of wound will cause wound healing disorders, resulting in delayed wound healing or non-healing. These influencing factors can be roughly divided into local factors and systemic factors according to the scope of influence (). Local factors directly affect the wound site and affect the normal healing process of the wound. Common local factors include abnormal local pressure, impaired vascular perfusion, direct stimulation of physical and chemical factors, and local nerve defects in wound. Local infection caused by bacteria or fungi is an important factor affecting wound healing.Citation35 A large number of bacteria will release endotoxin in the process of removal, causing necrosis and inflammation of local tissues.Citation36 Due to the increase of pro-inflammatory cytokines, the number of growth factors released decreased, which inhibited the growth of cells in the proliferation stage, resulting in delayed wound healing or unhealed ().
In addition to local factors, systemic factors also play an important role in wound healing. Individual basic conditions such as age, nutritional status, genetic susceptibility and other factors have a certain impact on the wound healing processCitation37 (). Personal habits such as smoking, alcohol consumption and hormone drug use also delay the healing process to varying degrees.Citation38 Finally, the health status of the body has an important influence on the healing process of the wound. Some chronic diseases such as diabetes and uremia will seriously affect the normal healing of wounds.Citation39 When the wound cannot heal within the normal healing time range under the influence of various adverse factors, and the wound still does not heal or has no tendency to heal after more than a month, this kind of wound is called chronic wound.Citation40 The normal wound healing process begins at the hemostatic/inflammatory stage and is completed by the proliferative and remodeling stagesCitation33(). Chronic wounds are in a continuous inflammatory stage under the action of local and systemic destructive factorsCitation41 (). The large release of pro-inflammatory cytokines leads to excessive synthetic release of metalloproteinases and inhibits the production of protease inhibitors.Citation42 This inhibits the formation of new matrix and basal cell-mediated epithelialization, while the matrix hydrolyzes massively under the action of protease and cell death. The cell debris further stimulates the accumulation of inflammatory cells in the wound area, creating a vicious cycle.Citation43
Effects of Diabetes on Wound Healing
Common causes of chronic wounds include diabetes, venous ulcers of the lower extremities, and pressure ulcers. Diabetic foot ulcers occur in about 15% of people with diabetes and account for 80% of all lower limb amputations.Citation2 Diabetes can affect wound healing in many ways and stages (). The hyperglycemia associated with diabetes can lead to microvascular disease, a narrowing or even occlusion of the capillaries that carry nutrients to the wound.Citation44 At the same time, hemoglobin glycosylation and changes in the structure of erythrocyte membrane led to insufficient oxygen and nutrient supply in the injured area, which affects wound healing.Citation45 Bone marrow cells represented by macrophages and neutrophils play an important role in the inflammatory stage during wound healing, while oxidative stress and hyperglycemia caused by local hypoxia change the expression of related genes that lead to macrophage polarization and its regulatory changes, leading to macrophage polarization disorder.Citation46 Overactivation of macrophages leads to over release of pro-inflammatory cytokines, and the continued inflammatory phase leads to abnormal apoptosis of fibroblasts and keratinocytes, interrupted the normal transition from the inflammatory phase to the proliferative phase of the woundCitation47 ().
Figure 3 Differences of wound healing between diabetic and non-diabetic patients.
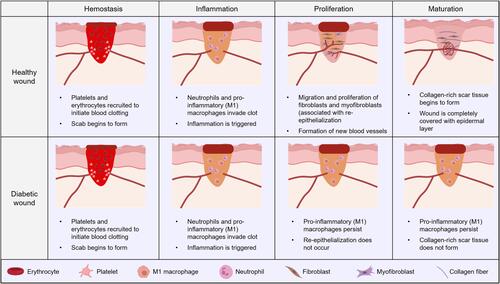
Oxidative stress caused by free radicals and reactive oxygen species has adverse effects on wound healing in diabetic patients. ROS is a regulator in the process of wound healing. Low levels of ROS can promote the aggregation of inflammatory cells in the inflammatory stage and inhibit the colonization and growth of bacteria on the wound surface.Citation48 However, in diabetic patients, activated polyol pathway, hexosamine pathway, activated AGE pathway, PKC pathway and other pathways stimulate the excessive production of reactive oxygen species in mitochondria.Citation49–Citation51 A large number of ROS triggers a severe local oxidative stress response, resulting in excessive accumulation of inflammatory cells and prolonged inflammatory period.Citation52 At the same time, excessive ROS affects the normal synthesis and reepithelialization of collagen in fibroblasts and keratinocytes, leading to the failure of the wound to enter the normal proliferation and remodeling stagesCitation53,Citation54 ().
Growth factors also play an important role in wound healing. IGF-1 is involved in cell granulation and epithelial regeneration, and stimulates chemotaxis of endothelial cells and proliferation of keratinocytes and fibroblasts. TGF-β can recruit fibroblasts and keratinocytes in the wound area, stimulate the synthesis and release of growth factors, and accelerate the formation of damaged local blood vessels and ECM formation.Citation55 PDGF can promote the synthesis of glycosaminoglycan, proteoglycan and collagen in fibroblasts, and play an important role in the migration and proliferation of fibroblasts and the formation of ECM.Citation56 The decreased expression levels of IGF-1, TGF-β and PDGF in wound tissue of diabetic patients slowed down the proliferation and differentiation of fibroblasts and the formation of ECM, and prolonged wound healing time.Citation57 VEGF is the main growth factor promoting angiogenesis and angiogenesis. The decreased VEGF mRNA expression level and increased VEGFR-1 level in diabetic patients lead to delayed angiogenesis and are not conducive to wound healing.Citation58 Under the combined action of the above-mentioned factors, the diabetic wound healing disorder is easily developed into chronic wound prolonged without healing.
Deficiencies of Existing Materials and Properties of Ideal Wound Excipients
Available Wound Dressings and Characteristics
After the wound was formed, various wound dressings covering the wound were noticed and soon used in treatments to promote wound healing (). With the passage of time, the development of science and technology and the deepening of people’s understanding of the mechanism of wound healing, the functions of wound dressings are constantly changing and updating. The traditional wound dressing is mainly made of gauze, cotton and polyester fiber. These wound dressing materials are easy to obtain, easy to prepare, and can fully absorb the local exudation of the wound to help the wound drainage.Citation59,Citation60 However, the deficiency of traditional wound dressing limits its protection and healing effect. Traditional wound dressings lack moisture, and excessive water absorption makes the wound exude and absorb quickly. After drying, wound dressings stick to the wound, which can easily cause secondary injury when dressing is replaced.Citation8 Traditional excipients can only provide a physical barrier to the wound surface, but their large pore structure cannot isolate the direct contact between bacteria in the environment and the wound surface.Citation61 At the same time, these traditional dressings also lack antibacterial and anti-inflammatory ability, which cannot effectively create an appropriate aseptic environment for wound healing.Citation62 Because of the disadvantages mentioned above, traditional excipients are gradually being replaced by more advantageous modern wound dressings.
Figure 4 Development of wound dressings and characteristics. The advantages and disadvantages of different dressings are shown in green and red squares, respectively.
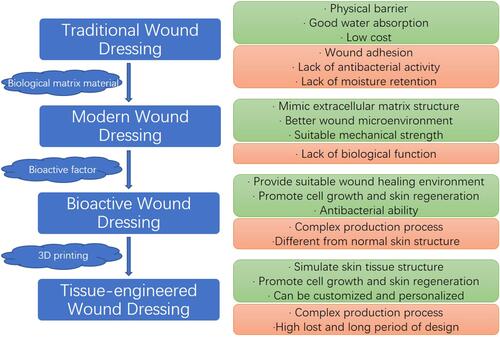
Modern wound dressings are constructed using substrate materials with superior properties (). These constructed wound dressings can mimic the structure of extracellular matrix to some extent and promote wound healing by inducing cell growth.Citation63,Citation64 At the same time, all kinds of matrix materials themselves have a certain promoting effect on wound healing. Alginate, for example, has properties such as stopping bleeding, moisturizing and allowing gas exchange.Citation65 In addition, by preparing wound dressings with different forms of matrix materials, different properties and functions can be given to the dressings, thus providing a better microenvironment for wound healing.Citation66 On this basis, various bioactive substances were added to the matrix material to improve the properties and functions of wound dressings, endowing them with anti-inflammatory, antibacterial, proliferation and differentiation biological functions. These wound dressings are called bioactive dressings, which promote the growth and healing of wound surface cells by loading them with bioactive substancesCitation7 (). Bioactive dressings with biological functions can obviously promote skin wound healing, but the final healing effect is still not as good as that of autologous skin grafting. In order to better simulate the structure and state of autologous skin grafting, tissue engineering dressings begin to receive attention (). Tissue-engineered dressings use different kinds of polymers or 3D printing technology to simulate dermal tissue structure consistent with normal skin tissue, thereby promoting cell migration, adhesion and proliferation and speeding up the repair process.Citation8
Properties of Ideal Wound Excipients
At present, all existing wound dressings can promote wound healing and repair to a certain extent, but due to the limitations of material properties and technology, there is no perfect wound dressing product that can be applied in clinical treatment. From the original gauze, bandage and cotton to the latest tissue-engineered dressings and smart dressings, the level and properties of dressings continues to advance. The original traditional dressing can only play a certain physical barrier and drainage and absorption function to the wound, and it is easy to stick to the wound and cause secondary injury due to its lack of anti-inflammatory and antibacterial ability.Citation8,Citation61 On this basis, a variety of modern dressings based on natural materials such as chitosan and sodium alginate and synthetic materials such as polyurethane and PNIPAAm have been developed.Citation67–Citation69 By preparing these matrix materials into hydrogel, hydrocolloid and other forms, the moisturizing and water absorption of the dressing can be significantly improved, which can maintain appropriate fitting with the wound and provide certain humidity for the wound, which is conducive to wound healing.Citation7 However, the properties of a single biological matrix material are relatively limited, unable to meet the needs of wound anti-inflammatory, antibacterial, hemostatic and growth promotion. On this basis, the dressing was further modified by adding bioactive substances into functional matrix materials such as chitosan and hyaluronic acid to enrich the function of the dressing.Citation70,Citation71 Adding silver ions to the matrix, for example, can improve the antibacterial ability of the dressing, improve the immune response, and speed wound healing.Citation72 The addition of NGF to matrix stimulates the release of nerve cells, immune cells and other cytokines, and promotes the migration and growth of fibroblasts.Citation73 Although bioactive materials can provide a more suitable microenvironment for wound healing, their structure is still different from that of normal skin. Therefore, 3D printing or a variety of materials have been used to construct tissue-engineered dressings that more closely resemble normal skin tissue structure, providing a better skeletal structure for cell growth and skin remodeling. However, its relatively high cost limits the application of this dressing.Citation74
In summary, the ideal skin wound dressing should have the following properties.Citation75 (1) good moisturizing effect, (2) good water absorption ability, (3) good air permeability, (4) appropriate mechanical strength, (5) good attachment to wound, but not sticky with the wound, (6) antimicrobial properties, (7) good biocompatibility, (8) relieve wound pain, (9) low economic cost, (10) simulate skin tissue structure, promote cell growth and skin regeneration. For diabetic wounds, more factors are needed. For example, the pathological changes at different stages in diabetic chronic wounds, the emergence of multiple drug-resistant bacteria, and the effects of some other treatments and drugs on wound healing.Citation76,Citation77
Characteristics and Preparation of Nanoparticles
The Types of Nanoparticles
Nanoparticles, also known as ultra-microparticles, range in size from 1 to 1000nm, with diameters in the transitional region between the cluster of atoms and the macroscopic object.Citation78 The small particle diameter of nanoparticles gives them very high specific surface area, quantum size effect and macroscopic tunneling effect.Citation79 These characteristics make the material in the state of nano particles have significant improvement in photoelectric, thermodynamic and magnetic physical properties. At the same time, the high specific surface area of nanoparticles makes them have a good application prospect in the fields of reaction catalysis, drug delivery and biotherapy.Citation80 Nanoparticles can be roughly divided into inorganic nanoparticles and organic nanoparticles according to their raw materials. Inorganic nanoparticles can be further divided into metal nanoparticles and non-metal nanoparticles.
Preparation Method of Nanoparticles
Inorganic Nanoparticles
Different kinds of nanoparticles have different preparation methods. According to the size of the starting material, inorganic nanoparticles can be roughly divided into bottom-up and top-down methods.Citation81 The basic principle of top-down is to reduce the size of the starting material by mechanical means or laser ablation to achieve the purpose of preparing nanoparticles. The specific methods mainly include mechanical milling, laser ablation and ion sputtering.Citation82–Citation84 Mechanical milling is the use of ball mill, bulk raw materials and grinding ball together in the container, by adjusting grinding parameters and to the reaction system to add different kinds of proportion of raw materials to achieve the particle size reduction of raw materials, and the use of chemical reaction between different raw materials to achieve the purpose of modification.Citation82 This preparation method can produce high purity nanoparticles on a large scale and is simple. However, the grinding process requires a long time and a high energy supply, and the materials of the grinding sphere may also be mixed into nanoparticles during the grinding process to reduce the purity of the product.Citation85 Laser ablation method uses high energy laser beam to irradiate the target material, causing the fragmentation of raw materials in the form of nanoparticles.Citation86 The nanoparticles generated after fragmentation form colloid with the solution of soaking raw materials. This method can prepare nanoparticles in suspension state. Nanoparticles with different parameters can be obtained by adjusting the duration and wavelength of laser pulse.Citation87 However, due to the product forming colloid and dispersing in the solution, the contact between laser and raw material is hindered, which limits the overall yield.Citation88 Another preparation method is ion sputtering, in which the solid surface is vaporized by an inert gas ion beam to produce nano-diameter particles.Citation89 This method is especially suitable for the preparation of refractory metals and intermetallic compounds, but its application is limited by complex preparation conditions.Citation90
The bottom-up approach starts with smaller molecules that contain raw materials and eventually forms nanoparticles through physical or chemical reactions. According to the state of matter in the preparation process of nanoparticles can be roughly divided into solid method, liquid method and gas phase method.Citation91–Citation93 The solid-state method excites or breaks the raw materials into nanoparticles by means of pulse laser and thermoactive chemical vapor deposition, and then redeposits them as films or particles on the surface of the collection plate.Citation91 Liquid synthesis methods mainly include sol-gel method, chemical reduction method and solvothermal method.Citation92 Different kinds of nanoparticles are prepared by redox reaction and gel formation. The gas phase method is to spray the precursor solution into the reactor to form atomized steam, which forms nanoparticles under the action of photothermal and is finally collected in the collection system. According to the different reaction chamber can be divided into spray pyrolysis, laser pyrolysis and flame pyrolysis.Citation94,Citation95 In addition to the above methods, the green synthesis method using microorganisms or plants has been increasingly widely used in recent years. The desired nanoparticles are obtained by applying different microorganisms and enzymes, isolates and extracts of plant products.Citation96 This preparation method does not involve the use of high temperature and high pressure and toxic chemicals, and is an economical, efficient and environmentally friendly preparation method. After solving the safety risk of microorganism and the purity of plant extraction, it will have excellent application prospect.Citation97
Organic Nanoparticles
The preparation methods of organic nanoparticles are different from those of inorganic nanoparticles. Due to the limitations of organic compounds, most of the preparation methods are completed in liquid phase.Citation98 It can be divided into monomer polymerization and polymer dispersion according to top-down and bottom-up synthesis methods. The classical methods to obtain polymer nanoparticles by polymerization of monomers are emulsion polymerization and solution-like polymerization.Citation99,Citation100 By limiting the polymer monomer and initiator system to a small space, the size of the product is controlled by controlling the micellar size, so that the diameter of the product is controlled at the nanometer level. Common methods include conventional emulsion polymerization, soap-free emulsion polymerization, fine emulsion polymerization and microemulsion polymerization.Citation101–Citation104 Monomer polymerization technology is mature and suitable for the preparation of large-scale nanoparticles. However, the products prepared by this method often have residues of unreacted monomers and initiators, which affect the purity of the products to a certain extent.Citation105 At the same time, various adjuvants and surfactants in the reaction system may have certain toxicity, which limits the application of the product in the biological field.Citation104
The top-down method is to prepare small-sized polymer nanoparticles by dispersing and emulsifying large-sized polymer materials. The specific methods include emulsification-solvent evaporation method, emulsification-solvent diffusion method, solvent substitution method, supercritical fluid technology and nano precipitation method.Citation106–Citation110 Compared with monomer synthesis, these methods are easier to operate and require milder conditions than monomer polymerization, so they are more suitable for the preparation of biological related nanomaterials.Citation111 In the dispersion process, drugs or other substances can also be mixed to prepare drug-carrying nanoparticles or modified nanoparticles, which enriches the function and effect of nanoparticles.Citation112 Although this preparation method solves the problem of monomer and toxic substance residues, but the control effect of product size is not good, and further research is needed.
The Mechanism of Nanoparticles
Wound healing is a continuous and dynamic process, in addition to the body’s own internal healing process, a good external environment and various beneficial interventions for wound healing can accelerate the healing process. In this process, nanoparticles play a role in promoting wound healing by virtue of their two characteristics. The first kind of nanoparticles are used in wound dressings by virtue of their biological functions. For example, the metal nanoparticles represented by silver nanoparticles can attach to the bacterial cell membrane to promote the destruction of cell membrane structure and inhibit the activity of bacterial enzymes, thus producing good antibacterial effect.Citation113 The other kind of nanoparticles are mainly used in the construction of wound dressings by virtue of their physical and chemical properties. These nanoparticles become carriers of some drugs or growth factors through the adsorption capacity of their high specific surface area or the ability of their surface chemical structure to bind to drugs. For example, when PLGA nanoparticles are loaded with VEGF, the exogenous local delivery of VEGF can be realized, which can promote the formation of neovascularization and reepithelialization of wound tissue, and accelerate the formation of granulation tissue.Citation114
Effects of Nanoparticles on Wound Healing
The biological function and physical characteristics of high specific surface area of nanoparticles make them have a very good application prospect in promoting wound healing. According to the different types of nanoparticles, we will introduce the representative inorganic and organic nanoparticles in the field of wound healing.
Inorganic Metal Nanoparticles
AgNPs
The antibacterial ability of Ag in nature has long been noted, so AgNPs is one of the earliest nanoparticles used in skin healing. Solid silver has no antibacterial properties and only has its biological effects when it is in its soluble form.Citation115 Silver can interfere with the cytochrome in the bacterial respiratory chain, blocking the microbial respiratory chain.Citation116 At the same time, silver can also change the membrane potential, damage the membrane function of microorganisms.Citation117 In addition, silver can also bind to the genetic material of microorganisms, inhibiting the normal replication, transcription and translation in microbial cells.Citation118 Silver also promotes wound healing by regulating the levels of cytokines in the wound to reduce inflammation, reduce lymphocyte infiltration, and enhance epithelial reformation.Citation119 In addition to its own antibacterial effect, AgNPs can also enhance the antibacterial ability of silver itself by combining with other materials or achieve antibacterial effect through other ways. Zhou et al found that the constructed Ag/AgCl/rGO nanomaterials could achieve bactericidal effect by promoting the generation of oxygen free radicals or oxidation free radicals.Citation120
While silver has proven antibacterial, antifungal, and antiviral properties, its direct application to the wound site alone poses a number of problems. Silver alone has a certain biological toxicity, and silver alone in the wound site cannot maintain a stable concentration of silver, easy to lead to local aggregation and adverse reactions, and the wound required a moist and breathable growth environment which is not AgNPs can provide.Citation118 Therefore, it is of great significance to modify AgNPs and equip them with other biological materials to prepare hydrogel, fiber membrane or scaffold and apply them in wound healing (). The existing modification applications are mainly developed from two aspects. One is to directly combine AgNPs with matrix materials with good biocompatibility or certain biological activity. Matrix materials play the role of carrier, and AgNPs plays a major role in dressing. For example, Chen et al prepared AgNPs using green deoxidizing agent egg white, and then added konjac glucomannan (KGM) powder to AgNPs solution to prepare KGM/AgNPs composite sponge.Citation121 The results showed that this wound dressing had good antibacterial activity, and could promote the growth of fibroblasts, accelerate wound epithelialization and wound healing. Another approach is to modify AgNPs, which is combined with other biologically active drugs, proteins or factors, and then loaded with the complex in the matrix material for promoting wound healing. In this combination, AgNPs and its bound drugs, proteins or factors often play a synergistic role, which enhances the biological effects of each other while retaining their own characteristics, so as to achieve better therapeutic effects. For example, the combined application of chitosan and AgNPs can enhance their own antibacterial effect and further improve the overall antibacterial effect.Citation122 Liu et al modified AgNPs with aloe vera, which not only improved the antibacterial ability of AgNPs, but also endowed the combined ability to promote proliferation and migration.Citation123 They applied the aloe vera in the hydrogel based on silk fibroin protein to treat full-layer skin wounds, and the results showed obvious healing effect.
Table 1 Different Kinds of Material Combinations of AgNPs and Their Therapeutic Effect
AuNPs
Gold nanoparticles have the same biocompatibility as silver and are currently used in drug delivery, photothermal therapy and tissue regeneration. When gold nanoparticles come into contact with bacteria, they form void structures in the cell walls of the bacteria, causing the loss of bacterial contents.Citation10 AuNPs can also cause bacterial metabolic dysfunction by interfering with ATP synthesis. In addition, AuNPs, like AgNPs, can interfere with the transcription and replication of genetic material and inhibit bacterial proliferation until death.Citation135 AuNPs also has significant antioxidant activity, which can reduce DPPH, OH and other reactive oxygen components by neutralizing free radicals.Citation136 The antibacterial and antioxidant effects of AuNPs can inhibit the accumulation of reactive oxygen species, reduce the accumulation of inflammatory cells, and maintain a sterile environment in the wound, thus promoting wound healing.Citation2 AuNPs also has sensitization effect. When AuNPs is combined with other antibacterial drugs or substances, the antibacterial effect can be significantly improved.Citation137 This feature also makes AuNPs have a wide prospect of combined application in the field of wound healing ().
Table 2 Different Kinds of Material Combinations of AuNPs and Their Therapeutic Effect
Chen et al constructed a wound dressing based on dextran/sericin, and carried AuNPs into the material to construct a wound dressing with significant antibacterial activity. Animal experimental results showed that wound healing was significantly accelerated and scar formation was significantly reduced.Citation138 Although AuNPs can inhibit bacterial growth and promote wound healing, more often AuNPs is used in combination with other antibacterial substances or particles. Li et al constructed a chitosan wound dressing embedded with Au-Ag nanoparticles, and compared with CS-Ag without Au, the antibacterial effect of CS-Au-Ag was significantly enhanced. In the presence of AuNPs, lower concentrations of silver nanoparticles can achieve the same antibacterial effect as CS-Ag. AuNPs also have similar effects to drugs with antibacterial properties.Citation139 Zou et al constructed a KGM/Gelatin@AuNPs/GS sponge by combining gentamicin sulfate (GS) with AuNPs. The results showed that the combined application of GS and AuNPs had significantly better antibacterial effect than AuNPs or AuNPs alone, which could effectively remove superbacteria, inhibit bacterial growth and promote wound healing.Citation140
CuNPs
In addition to AgNPs and AuNPs, two of the most widely used nanoparticles in wound healing, other metal nanoparticles have also been used in the design of wound healing dressings. CuNPs can release Cu2+, which changes the enzyme’s function by solidifying the protein structure, thus achieving its killing effect on bacteria.Citation147 Studies have found that copper has significant inhibitory effect on a variety of fungi and drug-resistant bacteria, which is very beneficial for chronic wounds of diabetes.Citation148,Citation149 As a cofactor of superoxide dismutase and cytochrome oxidase, copper can promote the formation of the enzymes.Citation150 Copper also boosts immunity by stimulating the production of interleukin 2. Copper also induces the expression of VEGF, which stimulates angiogenesis in the wound.Citation151 However, the antimicrobial effect of copper is dose-dependent, and high concentration of copper has certain toxicity to cells, which should be considered in dressing design.Citation152
CuNPs is also used in both direct and modified applications. Klinkajon et al constructed a copper alginate hydrogel using alginate, and the results showed that the effluent gel showed significant antibacterial activity against Escherichia coli, Staphylococcus aureus, methicillin-resistant Staphylococcus aureus (MRSA), Staphylococcus epidermidis and Streptococcus, which was proportional to the Concentration of Cu2+ ions.Citation160 Modified CuNPs can be used in wound dressings to obtain better therapeutic effect (). Xu et al constructed a composite hydrogel composed of PDA and Cu-Cs doped calcium silicate ceramic, and found that the hydrogel has a rapid and long-term inhibitory effect on methicillin-resistant Staphylococcus aureus and Escherichia coli, and can promote blood vessel formation and accelerate wound healing.Citation153
Table 3 Different Kinds of Material Combinations of CuNPs and Their Therapeutic Effect
Other Metal Nanoparticles
Metal nanoparticles can also play a role in promoting wound healing in the form of compounds in addition to acting as simple substances. ZnO can kill bacteria and fungi by accumulating ROS directly on the surface of bacteria and inducing ROS production.Citation161 As the main component of metalloproteinase, Zn can participate in the formation of ECM and promote wound healing.Citation162 Li et al constructed a ZnO/Ag/PVP/PCL nanofiber to enhance tissue regeneration and wound healing, and found that this composite structure had better anti-inflammatory, antibacterial, skin regeneration and wound healing effects than traditional drugs or single metal nanofibers.Citation163 In addition to ZnO, TiO2, CeO2, Fe2O3 and other emerging nanoparticles also play a certain role in skin wound healing. TiO2 has good biocompatibility and bioactivity, and also has good water retention effect, which can promote the healing of burn wounds.Citation164,Citation165 Iron oxide nanoparticles are easy to synthesize, have good degradation and low toxicity, and also have certain antibacterial effect.Citation166,Citation167 Cerium oxide nanoparticles have strong free radical scavenging ability, which can significantly improve the free radical aggregation of chronic diabetic wounds.Citation168,Citation169 Meanwhile, cerium oxide also has good biocompatibility,Citation170 which makes it have a good application prospect in the field of diabetic wound healing.
Inorganic Non-Metallic Nanoparticles
SiO2 Nanoparticles
Silicon dioxide, one of the non-metallic materials, has attracted much attention because of its good biological properties. Silica has good biocompatibility and does not cause additional immune responses in organisms, especially in wounds.Citation171,Citation172 The raw materials of silica are easy to obtain, and the processing and preparation methods are mature. Silica can be prepared into mesoporous nanoparticles, non-porous nanoparticles, silica gel and other structural forms.Citation173 These structures give silica good physical and chemical adsorption capacity and can be used as an ideal carrier for carrying a variety of drugs and proteins. Mirzahosseinipour et al constructed curcumin-silica nanoparticles by loading curcumin onto the surface of silica nanoparticles, taking advantage of silica’s good drug loading capability.Citation174 The composite nanoparticles showed photodynamic inactivation against Pseudomonas aeruginosa and Staphylococcus aureus. Ferenc et al constructed a SiO2-PVP composite gel that demonstrated significant ability to promote wound contraction and epithelialization, with the regenerated epidermis showing better differentiation and multilayer structure.Citation175 Jin et al constructed a mesoporous Silicon nanoparticles loaded with Ag/AgBr, which has a very strong killing effect on bacteria under the photocatalytic actionCitation176 (). Alvarez et al constructed a silica-collagen I nanocomposite hydrogel loaded with gentamicin and rifamycin. This hydrogel showed good continuous drug release ability and antibacterial activity, which is beneficial to the infection prevention of chronic diabetic wounds and promote wound healing.Citation177
Figure 5 The corresponding wound photographs (A) and the recovery progress of the wounds (B) of control group and different concentration Ag/AgBr/MSNs (**p<0.01; ***p<0.001).
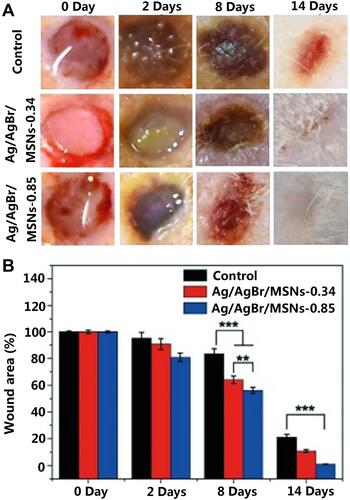
Carbon Nanoparticles
Carbon nanoparticles can be roughly divided into carbon dots, carbon nanotubes and graphene/GO according to the different combinations of carbon elements. The structure of carbon nanoparticles is beneficial to the growth of cells and tissues. Carbon nanotubes have a similar structure to collagen fibers and can affect cell adhesion, proliferation and differentiation.Citation11 Graphene’s unique base plane structure and shape allow it to be used for surface functionalization of drugs, biomolecules and other nanomaterials.Citation178 At the same time, the high surface area and adsorption capacity of graphene nanosheets make it conducive to cell adhesion and growth.Citation179 Carbon nanoparticles also have certain antibacterial activity and can enhance the antibacterial effect of other antibacterial substances. It was found that carbon dots, carbon nanotubes and graphene can kill bacteria in many ways, such as changing the surface charge environment of bacteria, mechanically destroying the cell wall of bacteria, and promoting ROS release.Citation180,Citation181 Cui et al constructed a carbon point release hydrogel with antibacterial activity, high biocompatibility and fluorescence properties. The results showed that carbon hydrogel has a long-term and broad-spectrum antibacterial abilityCitation182 (). Aslan et al constructed an antibacterial biofilm of carbon nanotubes assembled layer by layer with polypeptides, and the result shows a significant and rapid bactericidal effect.Citation183 Fan et al constructed a hydrogel based on silver and graphene polymers, which showed good bactericidal efficacy and accelerated wound healing.Citation184
Figure 6 Design strategy of the SCDs-AP hydrogel. (A) Synthesis of cationic antibacterial CDs starting from (B) assembly of the fluorescent antibacterial CDs hydrogel through pectin, acrylic acid, CDs, APS, and PEGDA. (C) Scheme of molecular structure and applications of fluorescent antibacterial hydrogel.
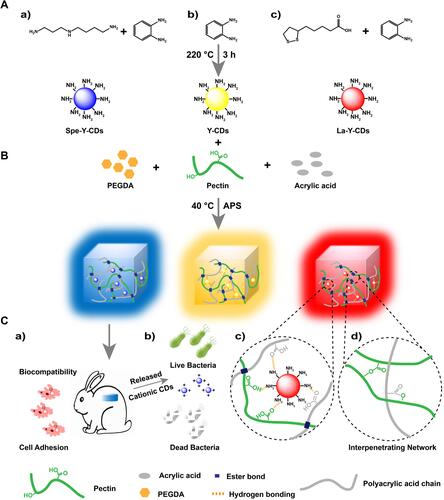
Organic Nanoparticles
Synthetic Polymer Nanoparticles
Biodegradable polymer materials with good biocompatibility are widely used in tissue bioengineering. Common synthetic polymers include but are not limited to PLGA, PCL, PU and polyethylene glycol. Nanoparticles prepared by synthetic polymer matrix have the following characteristics: There are various construction methods. Monomers or raw materials with different functional groups can be selected according to requirements to prepare nanoparticles that meet requirements. Rich functional groups can combine with a variety of drugs, growth factors or proteins to achieve the purpose of local drug delivery.Citation185 By adjusting the degradation rate and degradation environment of degradable polymer nanoparticles, the targeted drug or factor can be released at a fixed point or sustained release, so as to achieve better effect with a smaller dose.Citation2 Agel et al constructed a nanoparticle with PLGA, polyvinyl alcohol and chitosan as the matrix materials, and prepared a nanoparticle with good photodynamic effect and antibacterial performance on the surface loaded on the surface.Citation186 Hasan et al constructed a poly (lactic-co-glycolic acid)-polyethylenimine (PLGA-PEI) nanoparticles loaded with clindamycin, which can achieve continuous release of clindamycin and has a significant killing effect on MRSA.Citation187
Natural Polymer Nanoparticles
In addition to synthetic polymer nanoparticles, nanoparticles prepared from natural polymers also have a wide application prospect in wound healing (). Collagen, chitosan, hyaluronic acid, alginate and other natural polymers can be prepared into nanoparticles either alone or mixed with other drugs or particles in various ways. Compared with synthetic polymer, the structure of natural polymer is more similar to normal tissue, which is conducive to the formation of wound extracellular matrix.Citation188 At the same time, most natural polymers are polysaccharides or peptides, which have better hydrophilicity and adhesion, and are more suitable for cell adhesion growth on its surface.Citation9 In addition, some natural polymers have their own wound-healing properties. Chitosan itself has a certain inhibitory effect on the growth of bacteria, which can reduce the chance of wound infection.Citation189 Alginate can promote platelet aggregation and accelerate the early process of wound hemostasis.Citation190 Collagen itself as a protein, its degradation products can also be reused to promote wound cell growth and ECM formation.Citation191
Table 4 Different Kinds of Combinations of Natural Polymer and Their Therapeutic Effect
Although natural polymers are more often involved in the construction of wound dressings as matrix materials, their preparation as nanoparticles can give them more properties. Natural polymers also have some disadvantages. Some natural polymers may induce an immune response, and the rapid degradation rate of natural polymers is also an issue to be considered in wound dressing construction.Citation190,Citation199 Wang et al constructed a calcium alginate hydrogel supported by bioactive chitosan nanoparticles. This hydrogel shows significant antibacterial activity and promotes il-6 synthesis and secretion by regulating ROS production. Animal experiments showed that the gel could promote angiogenesis and accelerate wound healing.Citation192 Choudhary et al used silver nanoparticles as antibacterial agents, calcium alginate nanoparticles as hemostatic agents, serum to provide nutrients and growth factors, and chitosan as a matrix of composite hydrogels. The results showed that the combination had antibacterial effect, promoting proliferation, promoting angiogenesis and accelerating wound healing.Citation197
Future Perspectives
Compared with normal wound healing, chronic wound healing caused by diabetes has more complex healing process and more unfavorable self-condition environment. Long-term exposure to the external environment greatly increases the chance of infection. Meanwhile, the persistent inflammatory environment in chronic stage and the vascular barrier caused by hyperglycemia greatly delays the rate of tissue regeneration. On the basis of the antibacterial properties and good biocompatibility of the raw materials of nanoparticles, the high surface area, dispersion and adsorption brought by the nano-diameter particles give nanoparticles better delivery ability and binding performance.
Different kinds of nanoparticles have different advantages and disadvantages in the application of wound dressing. Metal nanoparticles are relatively easy to prepare and have better stability. At the same time, the ions brought by metal nanoparticles give it better antibacterial performance. However, the metabolism and accumulation of metal nanoparticles in vivo are also worthy of attention. The rich functional groups of organic nanoparticles can be combined with other drugs and growth factors to achieve better local delivery and sustained release effects. Some natural polymer-derived nanoparticles have better biocompatibility and are more suitable for cell adhesion. But the construction of organic nanoparticles is relatively more complex, so how to efficiently construct organic nanoparticles with uniform diameter also needs further exploration.
Using nanoparticles loaded with drugs and growth factors or using nanoparticles to modify traditional wound dressings to promote chronic wound healing has very definite therapeutic effects and advantages. But nanoparticles in application and diabetes chronic wound healing process there are still some problems need to consider: wound inflammatory environment in different stages of chronic wound healing has the opposite effect, how to make the nanoparticles on the inflammatory reaction in different stages of different degree of regulation is one of the problems that need to be solved in the future. The antibacterial properties of some nanoparticles showed obvious concentration dependence, but high concentration nanoparticles had certain cytotoxicity. How to balance antibacterial performance and biosafety is also an issue to be considered. The combination of multiple nanoparticles or nanoparticles with other drug factors may be a possible way to achieve a comprehensive therapeutic effect. Finally, it is also worth exploring how to improve the preparation process of nanoparticles and reduce the cost while ensuring purity and functionality, so that nanoparticles can be commercialized and applied in clinical practice. It is foreseeable that when these problems are solved, nanoparticles have unimaginable application prospects in chronic wound healing.
Author Contributions
All authors made a significant contribution to the work reported, whether that is in the conception, study design, execution, acquisition of data, analysis and interpretation, or in all these areas; took part in drafting, revising or critically reviewing the article; gave final approval of the version to be published; have agreed on the journal to which the article has been submitted; and agree to be accountable for all aspects of the work.
Acknowledgment
This work was supported by the National Natural Science Foundation of China Grants 31961133015, 21736002 to. F.W. and 31870791 to Z.M.
Disclosure
The authors declare that the research was conducted in the absence of any commercial or financial/non-financial relationships that could be construed as a potential conflict of interest.
References
- Zheng Y, Ley SH, Hu FB. Global aetiology and epidemiology of type 2 diabetes mellitus and its complications. Nat Rev Endocrinol. 2018;14(2):88–98. doi:10.1038/nrendo.2017.151
- Vijayakumar V, Samal SK, Mohanty S, Nayak SK. Recent advancements in biopolymer and metal nanoparticle-based materials in diabetic wound healing management. Int J Biol Macromol. 2019;122:137–148.
- Rodrigues M, Kosaric N, Bonham CA, Gurtner GC. Wound healing: a cellular perspective. Physiol Rev. 2019;99(1):665–706. doi:10.1152/physrev.00067.2017
- Childs DR, Murthy AS. Overview of wound healing and management. Surg Clin North Am. 2017;97(1):189–207. doi:10.1016/j.suc.2016.08.013
- Boniakowski AE, Kimball AS, Jacobs BN, Kunkel SL, Gallagher KA. Macrophage-mediated inflammation in normal and diabetic wound healing. J Immunol. 2017;199(1):17–24. doi:10.4049/jimmunol.1700223
- Lim JZ, Ng NS, Thomas C. Prevention and treatment of diabetic foot ulcers. J R Soc Med. 2017;110(3):104–109. doi:10.1177/0141076816688346
- Borda LJ, Macquhae FE, Kirsner RS. Wound dressings: a comprehensive review. Curr Dermatol Rep. 2016;5(4):287–297. doi:10.1007/s13671-016-0162-5
- Farahani M, Shafiee A. Wound healing: from passive to smart dressings. Adv Healthc Mater. 2021;10(16):e2100477.
- Boateng JS, Matthews KH, Stevens HN, Eccleston GM. Wound healing dressings and drug delivery systems: a review. J Pharm Sci. 2008;97(8):2892–2923. doi:10.1002/jps.21210
- Ampuero FO, Vidal A, Concha M. Nanoparticles for the treatment of wounds. Curr Pharmaceut Des. 2012;4(2):45–49.
- Yah CS, Simate GS. Nanoparticles as potential new generation broad spectrum antimicrobial agents. Daru-J Pharm Sci. 2015;23. doi:10.1186/s40199-015-0125-6
- Fore J. A review of skin and the effects of aging on skin structure and function. Ostomy Wound Manage. 2006;52(9):24–35; quiz 36–37.
- Blair MJ, Jones JD, Woessner AE, Quinn KP. Skin structure-function relationships and the wound healing response to intrinsic aging. Adv Wound Care. 2020;9(3):127–143. doi:10.1089/wound.2019.1021
- Wang PH, Huang BS, Horng HC, Yeh CC, Chen YJ. Wound healing. J Chin Med Assoc. 2018;81(2):94–101. doi:10.1016/j.jcma.2017.11.002
- Lee T, Friedman A. Skin barrier health: regulation and repair of the stratum corneum and the role of over-the-counter skin care. J Drugs Dermatol. 2016;15(9):1047–1051.
- Losquadro WD. Anatomy of the skin and the pathogenesis of nonmelanoma skin cancer. Facial Plast Surg Clin North Am. 2017;25(3):283–289. doi:10.1016/j.fsc.2017.03.001
- Madison KC. Barrier function of the skin: “la raison d’être” of the epidermis. J Invest Dermatol. 2003;121(2):231–241. doi:10.1046/j.1523-1747.2003.12359.x
- Gantwerker EA, Hom DB. Skin: histology and physiology of wound healing. Facial Plast Surg Clin North Am. 2011;19(3):441–453. doi:10.1016/j.fsc.2011.06.009
- Dąbrowska AK, Spano F, Derler S, Adlhart C, Spencer ND, Rossi RM. The relationship between skin function, barrier properties, and body-dependent factors. Skin Res Technol. 2018;24(2):165–174. doi:10.1111/srt.12424
- Angelova-Fischer I. Irritants and skin barrier function. Curr Probl Dermatol. 2016;49:80–89.
- Biggs LC, Kim CS, Miroshnikova YA, Wickström SA. Mechanical forces in the skin: roles in tissue architecture, stability, and function. J Invest Dermatol. 2020;140(2):284–290. doi:10.1016/j.jid.2019.06.137
- Lee SH, Jeong SK, Ahn SK. An update of the defensive barrier function of skin. Yonsei Med J. 2006;47(3):293–306. doi:10.3349/ymj.2006.47.3.293
- Takeo M, Lee W, Ito M. Wound healing and skin regeneration. Cold Spring Harb Perspect Med. 2015;5(1):a023267. doi:10.1101/cshperspect.a023267
- Blair P, Flaumenhaft R. Platelet alpha-granules: basic biology and clinical correlates. Blood Rev. 2009;23(4):177–189. doi:10.1016/j.blre.2009.04.001
- Locatelli L, Colciago A, Castiglioni S, Maier JA. Platelets in wound healing: what happens in space? Front Bioeng Biotechnol. 2021;9:716184. doi:10.3389/fbioe.2021.716184
- Golebiewska EM, Poole AW. Platelet secretion: from haemostasis to wound healing and beyond. Blood Rev. 2015;29(3):153–162. doi:10.1016/j.blre.2014.10.003
- Raja; Sivamani K, Garcia MS, Isseroff RR. Wound re-epithelialization: modulating keratinocyte migration in wound healing. Front Biosci. 2007;12:2849–2868. doi:10.2741/2277
- Pastar I, Stojadinovic O, Tomic-Canic M. Role of keratinocytes in healing of chronic wounds. Surg Technol Int. 2008;17:105–112.
- Murohara T, Horowitz JR, Silver M, et al. Vascular endothelial growth factor/vascular permeability factor enhances vascular permeability via nitric oxide and prostacyclin. Circulation. 1998;97(1):99–107. doi:10.1161/01.CIR.97.1.99
- Schäffer M, Becker HD. [Immune regulation of wound healing]. Chirurg. 1999;70(8):897–908. Dutch. doi:10.1007/s001040050740
- Witte MB, Barbul A. General principles of wound healing. Surg Clin North Am. 1997;77(3):509–528. doi:10.1016/S0039-6109(05)70566-1
- Diegelmann RF. Cellular and biochemical aspects of normal and abnormal wound healing: an overview. J Urol. 1997;157(1):298–302. doi:10.1016/S0022-5347(01)65364-3
- Przekora A. A concise review on tissue engineered artificial skin grafts for chronic wound treatment: can we reconstruct functional skin tissue in vitro? Cells. 2020;9(7):1622. doi:10.3390/cells9071622
- Janis JE, Harrison B. Wound healing: part i. basic science. Plast Reconstr Surg. 2016;138(3 Suppl):9s–17s. doi:10.1097/PRS.0000000000002773
- Li Z, Knetsch M. Antibacterial strategies for wound dressing: preventing infection and stimulating healing. Curr Pharm Des. 2018;24(8):936–951. doi:10.2174/1381612824666180213141109
- Edwards R, Harding KG. Bacteria and wound healing. Curr Opin Infect Dis. 2004;17(2):91–96. doi:10.1097/00001432-200404000-00004
- Brown KL, Phillips TJ. Nutrition and wound healing. Clin Dermatol. 2010;28(4):432–439. doi:10.1016/j.clindermatol.2010.03.028
- Wilkinson HN, Hardman MJ. The role of estrogen in cutaneous ageing and repair. Maturitas. 2017;103:60–64. doi:10.1016/j.maturitas.2017.06.026
- Beyene RT, Derryberry SL Jr, Barbul A. The effect of comorbidities on wound healing. Surg Clin North Am. 2020;100(4):695–705. doi:10.1016/j.suc.2020.05.002
- Wild T, Rahbarnia A, Kellner M, Sobotka L, Eberlein T. Basics in nutrition and wound healing. Nutrition. 2010;26(9):862–866. doi:10.1016/j.nut.2010.05.008
- Martin P, Nunan R. Cellular and molecular mechanisms of repair in acute and chronic wound healing. Br J Dermatol. 2015;173(2):370–378. doi:10.1111/bjd.13954
- Hesketh M, Sahin KB, West ZE, Murray RZ. Macrophage phenotypes regulate scar formation and chronic wound healing. Int J Mol Sci. 2017;18(7):1545. doi:10.3390/ijms18071545
- Tie L, Xiaokaiti Y, Wang X, Chen AF, Li XJ. [Molecular mechanisms of diabetic wound healing]. Sheng Li Ke Xue Jin Zhan. 2010;41(6):407–412. Chinese.
- Schürmann C, Goren I, Linke A, Pfeilschifter J, Frank S. Deregulated unfolded protein response in chronic wounds of diabetic ob/ob mice: a potential connection to inflammatory and angiogenic disorders in diabetes-impaired wound healing. Biochem Biophys Res Commun. 2014;446(1):195–200. doi:10.1016/j.bbrc.2014.02.085
- Brem H, Tomic-Canic M. Cellular and molecular basis of wound healing in diabetes. J Clin Invest. 2007;117(5):1219–1222. doi:10.1172/JCI32169
- Basu Mallik S, Jayashree BS, Shenoy RR. Epigenetic modulation of macrophage polarization- perspectives in diabetic wounds. J Diabetes Complications. 2018;32(5):524–530. doi:10.1016/j.jdiacomp.2018.01.015
- Wynn TA, Barron L. Macrophages: master regulators of inflammation and fibrosis. Semin Liver Dis. 2010;30(3):245–257. doi:10.1055/s-0030-1255354
- Deng L, Du C, Song P, et al. The role of oxidative stress and antioxidants in diabetic wound healing. Oxid Med Cell Longev. 2021;2021:8852759. doi:10.1155/2021/8852759
- Srivastava SK, Yadav UC, Reddy AB, et al. Aldose reductase inhibition suppresses oxidative stress-induced inflammatory disorders. Chem Biol Interact. 2011;191(1–3):330–338. doi:10.1016/j.cbi.2011.02.023
- Kunkemoeller B, Bancroft T, Xing H, et al. Elevated thrombospondin 2 contributes to delayed wound healing in diabetes. Diabetes. 2019;68(10):2016–2023. doi:10.2337/db18-1001
- Chen CY, Abell AM, Moon YS, Kim KH. An advanced glycation end product (AGE)-receptor for AGEs (RAGE) axis restores adipogenic potential of senescent preadipocytes through modulation of p53 protein function. J Biol Chem. 2012;287(53):44498–44507. doi:10.1074/jbc.M112.399790
- Brownlee M. Biochemistry and molecular cell biology of diabetic complications. Nature. 2001;414(6865):813–820. doi:10.1038/414813a
- Cano Sanchez M, Lancel S, Boulanger E, Neviere R. Targeting oxidative stress and mitochondrial dysfunction in the treatment of impaired wound healing: a systematic review. Antioxidants. 2018;7(8). doi:10.3390/antiox7080098
- Shamiya Y, Ravi SP, Coyle A, Chakrabarti S, Paul A. Engineering nanoparticle therapeutics for impaired wound healing in diabetes. Drug Discov Today. 2021;27:1156–1166. doi:10.1016/j.drudis.2021.11.024
- Chong DLW, Trinder S, Labelle M, et al. Platelet-derived transforming growth factor-β1 promotes keratinocyte proliferation in cutaneous wound healing. J Tissue Eng Regen Med. 2020;14(4):645–649. doi:10.1002/term.3022
- Chicharro-Alcántara D, Rubio-Zaragoza M, Damiá-Giménez E, et al. Platelet rich plasma: new insights for cutaneous wound healing management. J Funct Biomater. 2018;9(1):10. doi:10.3390/jfb9010010
- Raziyeva K, Kim Y, Zharkinbekov Z, Kassymbek K, Jimi S, Saparov A. Immunology of acute and chronic wound healing. Biomolecules. 2021;11(5):700. doi:10.3390/biom11050700
- Bao P, Kodra A, Tomic-Canic M, Golinko MS, Ehrlich HP, Brem H. The role of vascular endothelial growth factor in wound healing. J Surg Res. 2009;153(2):347–358. doi:10.1016/j.jss.2008.04.023
- Pereira RF, Bártolo PJ. Traditional therapies for skin wound healing. Adv Wound Care. 2016;5(5):208–229. doi:10.1089/wound.2013.0506
- Zhang J, Hao J, Zhang J, Luo B, Liu P. [Development research of biological dressing]. Zhongguo Xiu Fu Chong Jian Wai Ke Za Zhi. 2015;29(2):254–259. Chinese.
- Horncastle J. Wound dressings. Past, present, and future. Med Device Technol. 1995;6(1):30–34, 36.
- Jones VJ. The use of gauze: will it ever change? Int Wound J. 2006;3(2):79–86. doi:10.1111/j.1742-4801.2006.00215.x
- Shi C, Wang C, Liu H, et al. Selection of appropriate wound dressing for various wounds. Front Bioeng Biotechnol. 2020;8:182. doi:10.3389/fbioe.2020.00182
- Triller C, Huljev D, Planinsek Rucigaj T. [Modern wound dressings]. Acta Med Croatica. 2013;67(Suppl 1):81–87. Croatian.
- Shen S, Chen X, Shen Z, Chen H. Marine polysaccharides for wound dressings application: an overview. Pharmaceutics. 2021;13(10):1666. doi:10.3390/pharmaceutics13101666
- Brumberg V, Astrelina T, Malivanova T, Samoilov A. Modern wound dressings: hydrogel dressings. Biomedicines. 2021;9(9):1235. doi:10.3390/biomedicines9091235
- Aderibigbe BA, Buyana B. Alginate in wound dressings. Pharmaceutics. 2018;10(2):42. doi:10.3390/pharmaceutics10020042
- Imran FH, Karim R, Maat NH. Managing burn wounds with SMARTPORE technology polyurethane foam: two case reports. J Med Case Rep. 2016;10(1):120. doi:10.1186/s13256-016-0918-3
- Alexander A, Khan J, Saraf S, Saraf S. Polyethylene glycol (PEG)-Poly(N-isopropylacrylamide) (PNIPAAm) based thermosensitive injectable hydrogels for biomedical applications. Eur J Pharm Biopharm. 2014;88(3):575–585. doi:10.1016/j.ejpb.2014.07.005
- Patrulea V, Ostafe V, Borchard G, Jordan O. Chitosan as a starting material for wound healing applications. Eur J Pharm Biopharm. 2015;97(Pt B):417–426. doi:10.1016/j.ejpb.2015.08.004
- Chattopadhyay S, Raines RT, Glick GD. Review collagen-based biomaterials for wound healing. Biopolymers. 2014;101(8):821–833. doi:10.1002/bip.22486
- Paladini F, Pollini M. Antimicrobial silver nanoparticles for wound healing application: progress and future trends. Materials. 2019;12(16):2540. doi:10.3390/ma12162540
- Barrientos S, Stojadinovic O, Golinko MS, Brem H, Tomic-Canic M. Growth factors and cytokines in wound healing. Wound Repair Regen. 2008;16(5):585–601. doi:10.1111/j.1524-475X.2008.00410.x
- Ehrenreich M, Ruszczak Z. Update on tissue-engineered biological dressings. Tissue Eng. 2006;12(9):2407–2424. doi:10.1089/ten.2006.12.2407
- Ghomi ER, Khalili S, Khorasani SN, Neisiany RE, Ramakrishna S. Wound dressings: current advances and future directions. J Appl Polym Sci. 2019;136(27):47738.
- Liu Y, Liu Y, Deng J, Li W, Nie X. Fibroblast growth factor in diabetic foot ulcer: progress and therapeutic prospects. Front Endocrinol (Lausanne). 2021;12. doi:10.3389/fendo.2021.744868
- Shanmugapriya K, Kim H, Saravana PS, Chun B-S, Kang HW. Fabrication of multifunctional chitosan-based nanocomposite film with rapid healing and antibacterial effect for wound management. Int J Biol Macromol. 2018;118:1713–1725. doi:10.1016/j.ijbiomac.2018.07.018
- Whitesides GM. Nanoscience, nanotechnology, and chemistry. Small. 2005;1(2):172–179. doi:10.1002/smll.200400130
- Farokhzad OC, Langer R. Impact of nanotechnology on drug delivery. ACS Nano. 2009;3(1):16–20. doi:10.1021/nn900002m
- LaVan DA, McGuire T, Langer R. Small-scale systems for in vivo drug delivery. Nat Biotechnol. 2003;21(10):1184–1191. doi:10.1038/nbt876
- Jamkhande PG, Ghule NW, Bamer AH, Kalaskar MG. Metal nanoparticles synthesis: an overview on methods of preparation, advantages and disadvantages, and applications. J Drug Deliv Sci Technol. 2019;53:101174. doi:10.1016/j.jddst.2019.101174
- Ullah M, Ali ME, Abd Hamid SB. Surfactant-assisted ball milling: A novel route to novel materials with controlled nanostructure - A review. Rev Adv Mater Sci. 2014;37(1–2):1–14.
- Houriet R, Vacassy R, Hofmann H. Synthesis of powders and films using a new laser ablation technique. Nanostructured Materials. 1999;11(8):1155–1163. doi:10.1016/S0965-9773(99)00406-7
- Nie M, Sun K, Meng DD. Formation of metal nanoparticles by short-distance sputter deposition in a reactive ion etching chamber. J Appl Phys. 2009;106(5):054314. doi:10.1063/1.3211326
- Thamizharasan S, Saravanan NA. Nanosization of drug biomaterials and its solubility enhancement by high energy ball milling. J Nanosci Technol. 2017;4:237–239.
- Semaltianos NG. Nanoparticles by laser ablation. Crit Rev Solid State Mater Sci. 2010;35(2):105–124. doi:10.1080/10408431003788233
- Barcikowski S, Menendez-Manjon A, Chichkov B, Brikas M, Raciukaitis G. Generation of nanoparticle colloids by picosecond and femtosecond laser ablations in liquid flow. Appl Phys Lett. 2007;91(8):083113. doi:10.1063/1.2773937
- Ghorbani HR. A review of methods for synthesis of Al nanoparticles. Oriental J Chem. 2014;30(4):1941–1949. doi:10.13005/ojc/300456
- Johnson GE, Moser T, Engelhard M, Browning ND, Laskin J. Fabrication of electrocatalytic Ta nanoparticles by reactive sputtering and ion soft landing. J Chem Phys. 2016;145(17):174701. doi:10.1063/1.4966199
- Chandra R, Chawla AK, Ayyub P. Optical and structural properties of sputter-deposited nanocrystalline Cu 2O films: effect of sputtering gas. J Nanosci Nanotechnol. 2006;6(4):1119–1123. doi:10.1166/jnn.2006.176
- Pandey PA, Bell GR, Rourke JP, et al. Physical vapor deposition of metal nanoparticles on chemically modified graphene: observations on metal-graphene interactions. Small. 2011;7(22):3202–3210. doi:10.1002/smll.201101430
- Guzmán MG, Dille J, Godet S. Synthesis of silver nanoparticles by chemical reduction method and their antibacterial activity. Int J Chem Biol Eng. 2009;2(3):104–111.
- Yang Y, Matsubara S, Xiong L, Hayakawa T, Nogami M. Solvothermal synthesis of multiple shapes of silver nanoparticles and their SERS properties. J Phys Chem C. 2007;111(26):9095–9104. doi:10.1021/jp068859b
- Okuyama K, Lenggoro IW. Preparation of nanoparticles via spray route. Chem Eng Sci. 2003;58(3–6):537–547. doi:10.1016/S0009-2509(02)00578-X
- Wallace R, Brown AP, Brydson R, Wegner K, Milne SJ. Synthesis of ZnO nanoparticles by flame spray pyrolysis and characterisation protocol. J Mater Sci. 2013;48(18):6393–6403. doi:10.1007/s10853-013-7439-x
- Mukherjee P, Ahmad A, Mandal D, et al. Fungus-mediated synthesis of silver nanoparticles and their immobilization in the mycelial matrix: a novel biological approach to nanoparticle synthesis. Nano Lett. 2001;1(10):515–519. doi:10.1021/nl0155274
- Korbekandi H, Iravani S, Abbasi S. Production of nanoparticles using organisms. Crit Rev Biotechnol. 2009;29(4):279–306. doi:10.3109/07388550903062462
- Amoabediny G, Haghiralsadat F, Naderinezhad S, et al. Overview of preparation methods of polymeric and lipid-based (niosome, solid lipid, liposome) nanoparticles: a comprehensive review. Int J Poly Mater Poly Biomater. 2018;67(6):383–400. doi:10.1080/00914037.2017.1332623
- Thickett SC, Gilbert RG. Emulsion polymerization: state of the art in kinetics and mechanisms. Polymer. 2007;48(24):6965–6991. doi:10.1016/j.polymer.2007.09.031
- Asua JM. Emulsion polymerization: from fundamental mechanisms to process developments. J Poly Sci Part a-Poly Chem. 2004;42(5):1025–1041. doi:10.1002/pola.11096
- Muñoz-Bonilla A, van Herk AM, Heuts JPA. Preparation of hairy particles and antifouling films using brush-type amphiphilic block copolymer surfactants in emulsion polymerization. Macromolecules. 2010;43(6):2721–2731. doi:10.1021/ma9027257
- Garay-Jimenez JC, Gergeres D, Young A, Lim DV, Turos E. Physical properties and biological activity of poly(butyl acrylate–styrene) nanoparticle emulsions prepared with conventional and polymerizable surfactants. Nanomedicine. 2009;5(4):443–451. doi:10.1016/j.nano.2009.01.015
- Liu G, Liu P. Synthesis of monodispersed crosslinked nanoparticles decorated with surface carboxyl groups via soapless emulsion polymerization. Colloids Surf a Physicochem Eng Asp. 2010;354(1):377–381. doi:10.1016/j.colsurfa.2009.05.016
- Norakankorn C, Pan Q, Rempel GL, Kiatkamjornwong S. Factorial experimental design on synthesis of functional core/shell polymeric nanoparticles via differential microemulsion polymerization. J Appl Polym Sci. 2010;116(3):1291–1298.
- Jiang X, Dausend J, Hafner M, et al. Specific effects of surface amines on polystyrene nanoparticles in their interactions with mesenchymal stem cells. Biomacromolecules. 2010;11(3):748–753. doi:10.1021/bm901348z
- Lee M, Cho YW, Park JH, et al. Size control of self-assembled nanoparticles by an emulsion/solvent evaporation method. Colloid Polym Sci. 2006;284(5):506–512. doi:10.1007/s00396-005-1413-3
- Niwa T, Takeuchi H, Hino T, Kunou N, Kawashima Y. Preparations of biodegradable nanospheres of water-soluble and insoluble drugs with D,L-lactide/glycolide copolymer by a novel spontaneous emulsification solvent diffusion method, and the drug release behavior. J Controlled Release. 1993;25(1):89–98. doi:10.1016/0168-3659(93)90097-O
- Musumeci T, Ventura CA, Giannone I, et al. PLA/PLGA nanoparticles for sustained release of docetaxel. Int J Pharm. 2006;325(1–2):172–179. doi:10.1016/j.ijpharm.2006.06.023
- Mishima K. Biodegradable particle formation for drug and gene delivery using supercritical fluid and dense gas. Adv Drug Deliv Rev. 2008;60(3):411–432. doi:10.1016/j.addr.2007.02.003
- Chung JW, Lee K, Neikirk C, Nelson CM, Priestley RD. Photoresponsive coumarin-stabilized polymeric nanoparticles as a detectable drug carrier. Small. 2012;8(11):1693–1700. doi:10.1002/smll.201102263
- Qian L, Ahmed A, Zhang H. Formation of organic nanoparticles by solvent evaporation within porous polymeric materials. Chem Commun (Camb). 2011;47(36):10001–10003.
- Chereddy KK, Coco R, Memvanga PB, et al. Combined effect of PLGA and curcumin on wound healing activity. J Controlled Release. 2013;171(2):208–215. doi:10.1016/j.jconrel.2013.07.015
- Gunasekaran T, Nigusse T, Dhanaraju MD. Silver nanoparticles as real topical bullets for wound healing. J Am Coll Clin Wound Specialists. 2011;3(4):82–96. doi:10.1016/j.jcws.2012.05.001
- Chereddy KK, Lopes A, Koussoroplis S, et al. Combined effects of PLGA and vascular endothelial growth factor promote the healing of non-diabetic and diabetic wounds. Nanomedicine. 2015;11(8):1975–1984. doi:10.1016/j.nano.2015.07.006
- Kalantari K, Mostafavi E, Afifi AM, et al. Wound dressings functionalized with silver nanoparticles: promises and pitfalls. Nanoscale. 2020;12(4):2268–2291. doi:10.1039/C9NR08234D
- Sondi I, Salopek-Sondi B. Silver nanoparticles as antimicrobial agent: a case study on E. coli as a model for gram-negative bacteria. J Colloid Interface. 2007;275:177–182. doi:10.1016/j.jcis.2004.02.012
- Hamdan S, Pastar I, Drakulich S, et al. Nanotechnology-driven therapeutic interventions in wound healing: potential uses and applications. ACS Central Sci. 2017;3(3):163–175. doi:10.1021/acscentsci.6b00371
- Prasath S, Palaniappan K. Is using nanosilver mattresses/pillows safe? A review of potential health implications of silver nanoparticles on human health. Environ Geochem Health. 2019;41(5):2295–2313. doi:10.1007/s10653-019-00240-7
- Tan HT, Rahman RA, Gan SH, et al. The antibacterial properties of Malaysian tualang honey against wound and enteric microorganisms in comparison to manuka honey. BMC Complement Altern Med. 2009;9:34. doi:10.1186/1472-6882-9-34
- Zhou YZ, Chen R, He TT, et al. Biomedical potential of ultrafine Ag/AgCl nanoparticles coated on graphene with special reference to antimicrobial performances and burn wound healing. ACS Appl Mater Interfaces. 2016;8(24):15067–15075. doi:10.1021/acsami.6b03021
- Chen HL, Lan GQ, Ran LX, et al. A novel wound dressing based on a Konjac glucomannan/silver nanoparticle composite sponge effectively kills bacteria and accelerates wound healing. Carbohydr Polym. 2018;183:70–80. doi:10.1016/j.carbpol.2017.11.029
- Verma J, Kanoujia J, Parashar P, Tripathi CB, Saraf SA. Wound healing applications of sericin/chitosan-capped silver nanoparticles incorporated hydrogel. Drug Deliv Transl Res. 2017;7(1):77–88. doi:10.1007/s13346-016-0322-y
- Liu YK, Fan JC, Lv MQ, et al. Photocrosslinking silver nanoparticles-aloe vera-silk fibroin composite hydrogel for treatment of full-thickness cutaneous wounds. Regen Biomater. 2021;8(6). doi:10.1093/rb/rbab048
- Tarusha L, Paoletti S, Travan A, Marsich E. Alginate membranes loaded with hyaluronic acid and silver nanoparticles to foster tissue healing and to control bacterial contamination of non-healing wounds. J Mater Sci-Mater Med. 2018;29(2). doi:10.1007/s10856-018-6027-7
- Wang Y, Dou CY, He GD, et al. Biomedical potential of ultrafine ag nanoparticles coated on poly (Gamma-Glutamic Acid) hydrogel with special reference to wound healing. Nanomaterials. 2018;8(5):324.
- Pyun DG, Yoon HS, Chung HY, et al. Evaluation of AgHAP-containing polyurethane foam dressing for wound healing: synthesis, characterization, in vitro and in vivo studies. J Mater Chem B. 2015;3(39):7752–7763. doi:10.1039/C5TB00995B
- Archana D, Singh BK, Dutta J, Dutta PK. Chitosan-PVP-nano silver oxide wound dressing: in vitro and in vivo evaluation. Int J Biol Macromol. 2015;73:49–57. doi:10.1016/j.ijbiomac.2014.10.055
- Song S, Liu Z, Abubaker MA, et al. Antibacterial polyvinyl alcohol/bacterial cellulose/nano-silver hydrogels that effectively promote wound healing. Mater Sci Eng C-Mater Biol Appl. 2021;126:112171. doi:10.1016/j.msec.2021.112171
- Masood N, Ahmed R, Tariq M, et al. Silver nanoparticle impregnated chitosan-PEG hydrogel enhances wound healing in diabetes induced rabbits. Int J Pharm. 2019;559:23–36. doi:10.1016/j.ijpharm.2019.01.019
- Basha SI, Ghosh S, Vinothkumar K, et al. Fumaric acid incorporated Ag/agar-agar hybrid hydrogel: a multifunctional avenue to tackle wound healing. Mater Sci Eng C-Mater Biol Appl. 2020;111:110743. doi:10.1016/j.msec.2020.110743
- Choi HJ, Thambi T, Yang YH, et al. AgNP and rhEGF-incorporating synergistic polyurethane foam as a dressing material for scar-free healing of diabetic wounds. RSC Adv. 2017;7(23):13714–13725. doi:10.1039/C6RA27322J
- Xuan X, Zhou YJ, Chen AQ, et al. Silver crosslinked injectable bFGF-eluting supramolecular hydrogels speed up infected wound healing. J Mater Chem B. 2020;8(7):1359–1370. doi:10.1039/C9TB02331C
- Lee YH, Hong YL, Wu TL. Novel silver and nanoparticle-encapsulated growth factor co-loaded chitosan composite hydrogel with sustained antimicrobility and promoted biological properties for diabetic wound healing. Mater Sci Eng C-Mater Biol Appl. 2021;118:111385. doi:10.1016/j.msec.2020.111385
- Singla R, Soni S, Patial V, et al. In vivo diabetic wound healing potential of nanobiocomposites containing bamboo cellulose nanocrystals impregnated with silver nanoparticles. Int J Biol Macromol. 2017;105:45–55. doi:10.1016/j.ijbiomac.2017.06.109
- Volkova O. Pole until the dream. World of News; 2016:22.
- Esumi K, Takei N, Yoshimura T. Antioxidant-potentiality of gold-chitosan nanocomposites. Colloids Surf B Biointerfaces. 2003;32(2):117–123. doi:10.1016/S0927-7765(03)00151-6
- Rajendran NK, Kumar SSD, Houreld NN, Abrahamse H. A review on nanoparticle based treatment for wound healing. J Drug Deliv Sci Technol. 2018;44:421–430. doi:10.1016/j.jddst.2018.01.009
- Chen P, Bian LN, Hu XY. Synergic fabrication of gold nanoparticles embedded dextran/silk sericin nanomaterials for the treatment and care of wound healing. J Cluster Sci. 2021;3:1
- Li Q, Lu F, Zhou GF, et al. Silver inlaid with gold nanoparticle/chitosan wound dressing enhances antibacterial activity and porosity, and promotes wound healing. Biomacromolecules. 2017;18(11):3766–3775. doi:10.1021/acs.biomac.7b01180
- Zou YN, Xie RQ, Hu EL, et al. Protein-reduced gold nanoparticles mixed with gentamicin sulfate and loaded into konjac/gelatin sponge heal wounds and kill drug-resistant bacteria. Int J Biol Macromol. 2020;148:921–931. doi:10.1016/j.ijbiomac.2020.01.190
- Cozad MJ, Bachman SL, Grant SA. Assessment of decellularized porcine diaphragm conjugated with gold nanomaterials as a tissue scaffold for wound healing. J Biomed Mater Res A. 2011;99A(3):426–434. doi:10.1002/jbm.a.33182
- Akturk O, Kismet K, Yasti AC, et al. Collagen/gold nanoparticle nanocomposites: a potential skin wound healing biomaterial. J Biomater Appl. 2016;31(2):283–301. doi:10.1177/0885328216644536
- Xia CX, Ren B, Liu N, Zheng Y, Feasible A. Strategy of fabricating of gold-encapsulated dextran/polyvinyl alcohol nanoparticles for the treatment and care of wound healing. J Cluster Sci. 2021;4:1–9.
- Mahmoud NN, Hikmat S, Abu Ghith D, et al. Gold nanoparticles loaded into polymeric hydrogel for wound healing in rats: effect of nanoparticles’ shape and surface modification. Int J Pharm. 2019;565:174–186. doi:10.1016/j.ijpharm.2019.04.079
- Pan AQ, Zhong ML, Wu H, et al. Topical application of keratinocyte growth factor conjugated gold nanoparticles accelerate wound healing. Nanomed-Nanotechnol Biol Med. 2018;14(5):1619–1628. doi:10.1016/j.nano.2018.04.007
- Wang K, Qi ZP, Pan S, et al. Preparation, characterization and evaluation of a new film based on chitosan, arginine and gold nanoparticle derivatives for wound-healing efficacy. RSC Adv. 2020;10(35):20886–20899. doi:10.1039/D0RA03704D
- Chatterjee AK, Chakraborty R, Basu T. Mechanism of antibacterial activity of copper nanoparticles. Nanotechnology. 2014;25(13):135101. doi:10.1088/0957-4484/25/13/135101
- Cady NC, Behnke JL, Strickland AD. Copper-based nanostructured coatings on natural cellulose: nanocomposites exhibiting rapid and efficient inhibition of a multi-drug resistant wound pathogen, A. baumannii, and mammalian cell biocompatibility in vitro. Adv Funct Mater. 2011;21(13):2506–2514. doi:10.1002/adfm.201100123
- Grass G, Rensing C, Solioz M. Metallic copper as an antimicrobial surface. Appl Environ Microbiol. 2011;77(5):1541–1547. doi:10.1128/AEM.02766-10
- Deryabin DG, Aleshina ES, Vasilchenko AS, et al. Investigation of copper nanoparticles antibacterial mechanisms tested by luminescent Escherichia coli strains. Nanotechnol Russia. 2013;8(5):402–408. doi:10.1134/S1995078013030063
- Bauer SM, Bauer RJ, Velazquez OC. Angiogenesis, vasculogenesis, and induction of healing in chronic wounds. Vasc Endovascular Surg. 2005;39(4):293–306. doi:10.1177/153857440503900401
- Tiwari M, Narayanan K, Thakar MB, Jagani HV, Rao JV. Biosynthesis and wound healing activity of copper nanoparticles. Iet Nanobiotechnol. 2014;8(4):230–237. doi:10.1049/iet-nbt.2013.0052
- Xu Q, Chang ML, Zhang Y, et al. PDA/Cu bioactive hydrogel with “hot ions effect” for inhibition of drug-resistant bacteria and enhancement of infectious skin wound healing. ACS Appl Mater Interfaces. 2020;12(28):31255–31269. doi:10.1021/acsami.0c08890
- Lemraski EG, Jahangirian H, Dashti M, et al. Antimicrobial double-layer wound dressing based on chitosan/polyvinyl alcohol/copper: in vitro and in vivo assessment. Int J Nanomedicine. 2021;16:223–235. doi:10.2147/IJN.S266692
- Sun XY, Dong MN, Guo ZR, et al. Multifunctional chitosan-copper-gallic acid based antibacterial nanocomposite wound dressing. Int J Biol Macromol. 2021;167:10–22. doi:10.1016/j.ijbiomac.2020.11.153
- Tao BL, Lin CC, Deng YM, et al. Copper-nanoparticle-embedded hydrogel for killing bacteria and promoting wound healing with photothermal therapy. J Mater Chem B. 2019;7(15):2534–2548. doi:10.1039/C8TB03272F
- Wang MQ, Xia A, Wu SS, Shen J. Facile synthesis of the Cu, N-CDs@GO-CS hydrogel with enhanced antibacterial activity for effective treatment of wound infection. Langmuir. 2021;37(26):7928–7935. doi:10.1021/acs.langmuir.1c00529
- Yang Y, Dong ZG, Li M, et al. Graphene oxide/copper nanoderivatives-modified chitosan/hyaluronic acid dressings for facilitating wound healing in infected full-thickness skin defects. Int J Nanomedicine. 2020;15:8231–8247. doi:10.2147/IJN.S278631
- Tao BL, Lin CC, Guo A, et al. Fabrication of copper ions-substituted hydroxyapatite/polydopamine nanocomposites with high antibacterial and angiogenesis effects for promoting infected wound healing. J Ind Eng Chem. 2021;104:345–355. doi:10.1016/j.jiec.2021.08.035
- Klinkajon W, Supaphol P. Novel copper (II) alginate hydrogels and their potential for use as anti-bacterial wound dressings. Biomed Mater. 2014;9(4):045008. doi:10.1088/1748-6041/9/4/045008
- Sudheesh Kumar PT, Lakshmanan V-K, Anilkumar TV, et al. Flexible and microporous chitosan Hydrogel/Nano ZnO composite bandages for wound dressing: in vitro and in vivo evaluation. ACS Appl Mater Interfaces. 2012;4(5):2618–2629. doi:10.1021/am300292v
- Lin SQ, Xu HW, Xiao J, et al. Combined use of acid fibroblast growth factor, granulocyte colony-stimulating factor and zinc sulphate accelerates diabetic ulcer healing. J Health Sci. 2009;55(6):910–922. doi:10.1248/jhs.55.910
- Li M, Hu M, Zeng HL, et al. Multifunctional zinc oxide/silver bimetallic nanomaterial-loaded nanofibers for enhanced tissue regeneration and wound healing. J Biomed Nanotechnol. 2021;17(9):1840–1849. doi:10.1166/jbn.2021.3152
- Peng -C-C, Yang M-H, Chiu W-T, et al. Composite nano-titanium oxide–chitosan artificial skin exhibits strong wound-healing effect—an approach with anti-inflammatory and bactericidal kinetics. Macromol Biosci. 2008;8(4):316–327. doi:10.1002/mabi.200700188
- Jeng HA, Swanson J. Toxicity of metal oxide nanoparticles in mammalian cells. J Environ Sci Health, Part A. 2006;41(12):2699–2711. doi:10.1080/10934520600966177
- Khashan KS, Sulaiman GM, Mahdi R. Preparation of iron oxide nanoparticles-decorated carbon nanotube using laser ablation in liquid and their antimicrobial activity. Artif Cells Nanomed Biotechnol. 2017;45(8):1699–1709. doi:10.1080/21691401.2017.1282498
- Ziv-Polat O, Topaz M, Brosh T, Margel S. Enhancement of incisional wound healing by thrombin conjugated iron oxide nanoparticles. Biomaterials. 2010;31(4):741–747. doi:10.1016/j.biomaterials.2009.09.093
- Dewberry LC, Niemiec SM, Hilton SA, et al. Cerium oxide nanoparticle conjugation to microRNA-146a mechanism of correction for impaired diabetic wound healing. Nanomedicine. 1800;40:102483. doi:10.1016/j.nano.2021.102483
- Augustine R, Hasan A, Patan NK, et al. Cerium oxide nanoparticle incorporated electrospun Poly(3-hydroxybutyrate-co-3-hydroxyvalerate) membranes for diabetic wound healing applications. Acs Biomater Sci Eng. 2020;6(1):58–70. doi:10.1021/acsbiomaterials.8b01352
- Es-haghi A, Mashreghi M, Bazaz MR, Homayouni-Tabrizi M, Darroudi M. Fabrication of biopolymer based nanocomposite wound dressing: evaluation of wound healing properties and wound microbial load. Iet Nanobiotechnol. 2017;11(5):517–522. doi:10.1049/iet-nbt.2016.0160
- Arriagada F, Nonell S, Morales J. Silica-based nanosystems for therapeutic applications in the skin. Nanomedicine. 2019;14(16):2243–2267. doi:10.2217/nnm-2019-0052
- Tang L, Cheng J. Nonporous silica nanoparticles for nanomedicine application. Nano Today. 2013;8(3):290–312. doi:10.1016/j.nantod.2013.04.007
- Chen L, Zhou XJ, He CL. Mesoporous silica nanoparticles for tissue-engineering applications. Wiley Interdiscip Rev Nanomed Nanobiotechnol. 2019;11(6). doi:10.1002/wnan.1573
- Mirzahosseinipour M, Khorsandi K, Hosseinzadeh R, Ghazaeian M, Shahidi FK. Antimicrobial photodynamic and wound healing activity of curcumin encapsulated in silica nanoparticles. Photodiagnosis Photodyn Ther. 2020;29:101639. doi:10.1016/j.pdpdt.2019.101639
- Ori F, Dietrich R, Ganz C, et al. Silicon-dioxide-polyvinylpyrrolidone as a wound dressing for skin defects in a murine model. J Cranio-Maxillofacial Surg. 2017;45(1):99–107. doi:10.1016/j.jcms.2016.10.002
- Jin C, Liu XM, Tan L, et al. Ag/AgBr-loaded mesoporous silica for rapid sterilization and promotion of wound healing. Biomater Sci. 2018;6(7):1735–1744. doi:10.1039/C8BM00353J
- Alvarez GS, Helary C, Mebert AM, Wang XL, Coradin T, Desimone MF. Antibiotic-loaded silica nanoparticle-collagen composite hydrogels with prolonged antimicrobial activity for wound infection prevention. J Mater Chem B. 2014;2(29):4660–4670. doi:10.1039/c4tb00327f
- Su JY, Liu Y. Advance in the research of graphene and its derivatives in wound dressings. Zhonghua shao shang za zhi. 2019;35(8):637–640. doi:10.3760/cma.j.issn.1009-2587.2019.08.021
- Menaa F, Abdelghani A, Menaa B. Graphene nanomaterials as biocompatible and conductive scaffolds for stem cells: impact for tissue engineering and regenerative medicine. J Tissue Eng Regen Med. 2015;9(12):1321–1338. doi:10.1002/term.1910
- Zhou J, Qi X. Multi-walled carbon nanotubes/epilson-polylysine nanocomposite with enhanced antibacterial activity. Lett Appl Microbiol. 2011;52(1):76–83. doi:10.1111/j.1472-765X.2010.02969.x
- Neelgund GM, Oki A, Luo Z. Antimicrobial activity of CdS and Ag2S quantum dots immobilized on poly(amidoamine) grafted carbon nanotubes. Colloids Surf B Biointerfaces. 2012;100:215–221. doi:10.1016/j.colsurfb.2012.05.012
- Cui FC, Sun JD, Ji J, et al. Carbon dots-releasing hydrogels with antibacterial activity, high biocompatibility, and fluorescence performance as candidate materials for wound healing. J Hazard Mater. 2021;406:124330. doi:10.1016/j.jhazmat.2020.124330
- Aslan S, Deneufchatel M, Hashmi S, et al. Carbon nanotube-based antimicrobial biomaterials formed via layer-by-layer assembly with polypeptides. J Colloid Interface Sci. 2012;388(1):268–273. doi:10.1016/j.jcis.2012.08.025
- Fan ZJ, Liu B, Wang JQ, et al. Dressing based on Ag/Graphene polymer hydrogel: effectively kill bacteria and accelerate wound healing. Adv Funct Mater. 2014;24(25):3933–3943. doi:10.1002/adfm.201304202
- Xu C, Akakuru OU, Ma X, Zheng J, Zheng J, Wu A. Nanoparticle-based wound dressing: recent progress in the detection and therapy of bacterial infections. Bioconjug Chem. 2020;31(7):1708–1723. doi:10.1021/acs.bioconjchem.0c00297
- Agel MR, Baghdan E, Pinnapireddy SR, Lehmann J, Schäfer J, Bakowsky U. Curcumin loaded nanoparticles as efficient photoactive formulations against gram-positive and gram-negative bacteria. Colloids Surf B Biointerfaces. 2019;178:460–468. doi:10.1016/j.colsurfb.2019.03.027
- Hasan N, Cao J, Lee J, et al. Bacteria-targeted clindamycin loaded polymeric nanoparticles: effect of surface charge on nanoparticle adhesion to MRSA, antibacterial activity, and wound healing. Pharmaceutics. 2019;11(5):236. doi:10.3390/pharmaceutics11050236
- Lin Y-M, Chen S-L, Chao C-F. R&D expenditure, financing policy, and default risk. Int J Econ Account. 2015;6(1):81–104. doi:10.1504/IJEA.2015.068982
- Elgadir MA, Uddin MS, Ferdosh S, Adam A, Chowdhury AJK, Sarker MZI. Impact of chitosan composites and chitosan nanoparticle composites on various drug delivery systems: a review. J Food Drug Anal. 2015;23(4):619–629. doi:10.1016/j.jfda.2014.10.008
- Sahana TG, Rekha PD. Biopolymers: applications in wound healing and skin tissue engineering. Mol Biol Rep. 2018;45(6):2857–2867. doi:10.1007/s11033-018-4296-3
- MacKay D, Miller AL. Nutritional support for wound healing. Altern Med Rev. 2003;8(4):359–377.
- Wang T, Zheng Y, Shen YP, et al. Chitosan nanoparticles loaded hydrogels promote skin wound healing through the modulation of reactive oxygen species. Artif Cells, Nanomed Biotechnol. 2018;46:S138–S149. doi:10.1080/21691401.2017.1415212
- Sheir MM, Nasra MMA, Abdallah OY. Chitosan alginate nanoparticles as a platform for the treatment of diabetic and non-diabetic pressure ulcers: formulation and in vitro/in vivo evaluation. Int J Pharm. 2021;607:120963. doi:10.1016/j.ijpharm.2021.120963
- Wani TU, Raza SN, Khan NA. Rosmarinic acid loaded chitosan nanoparticles for wound healing in rats. Int J Pharm Sci Res. 2019;10(3):1138–1147.
- Karri V, Kuppusamy G, Talluri SV, et al. Curcumin loaded chitosan nanoparticles impregnated into collagen-alginate scaffolds for diabetic wound healing. Int J Biol Macromol. 2016;93:1519–1529. doi:10.1016/j.ijbiomac.2016.05.038
- Oliveira DML, Rezende PS, Barbosa TC, et al. Double membrane based on lidocaine-coated polymyxin-alginate nanoparticles for wound healing: in vitro characterization and in vivo tissue repair. Int J Pharm. 2020;591:120001. doi:10.1016/j.ijpharm.2020.120001
- Choudhary M, Chhabra P, Tyagi A, Singh H. Scar free healing of full thickness diabetic wounds: a unique combination of silver nanoparticles as antimicrobial agent, calcium alginate nanoparticles as hemostatic agent, fresh blood as nutrient/growth factor supplier and chitosan as base matrix. Int J Biol Macromol. 2021;178:41–52. doi:10.1016/j.ijbiomac.2021.02.133
- Pereira GG, Detoni CB, Balducci AG, et al. Hyaluronate nanoparticles included in polymer films for the prolonged release of vitamin E for the management of skin wounds. Eur J Pharm Sci. 2016;83:203–211. doi:10.1016/j.ejps.2016.01.002
- Chaudhari AA, Vig K, Baganizi DR, et al. Future prospects for scaffolding methods and biomaterials in skin tissue engineering: a review. Int J Mol Sci. 2016;17(12):1974. doi:10.3390/ijms17121974