Abstract
Gold nanoparticles and near infrared-absorbing light are each innocuous to tissue but when combined can destroy malignant tissue while leaving healthy tissue unharmed. This study investigated the feasibility of photothermal ablation therapy for esophageal adenocarcinoma using chitosan-coated gold/gold sulfide (CS-GGS) nanoparticles. A rat esophagoduodenal anastomosis model was used for the in vivo ablation study, and three human esophageal cell lines were used to study the response of cancer cells and benign cells to near infrared light after treatment with CS-GGS. The results indicate that both cancerous tissue and cancer cells took up more gold nanoparticles and were completely ablated after exposure to near infrared light. The benign tissue and noncancerous cells showed less uptake of these nanoparticles, and remained viable after exposure to near infrared light. CS-GGS nanoparticles could provide an optimal endoluminal therapeutic option for near infrared light ablation of esophageal cancer.
Background
Esophageal cancer is the seventh most common cause of cancer-related deaths worldwide. Citation1 Although squamous cell carcinoma is still the dominant histological type in Europe and Asia, the incidence of adenocarcinoma has been on the increase in the US and Europe, and today accounts for approximately 30% of all esophageal cancers.Citation2–Citation4 The reasons for the increasing incidence are not clear, but hypotheses include population trends in obesity that increase the risk of gastroesophageal reflux, as well as the continuing decline in Helicobacter pylori infection.Citation5 Only a subpopulation of patients appears to benefit from treatment, which is generally either surgery or a combination of radiation and chemotherapy for localized tumors. Survival rates are poor for patients with esophageal cancer; patients with advanced metastatic cancer who are treated with palliative chemotherapy have a median survival of less than one year, and the 5-year survival rate of all patients diagnosed is only 14%.Citation6
Multimodality therapy remains the optimal treatment strategy for esophageal cancer, and includes surgery, radiotherapy, chemotherapy, and endoscopic therapy. However, limitations of all therapies persist in regard to performance status, comorbidities, intolerance to therapy, and limited local and systemic antineoplastic activity. Neither radiotherapy nor chemotherapy has shown a significant survival advantage.Citation7 In addition, esophageal stenosis worsens during the course of radiation therapy, and patients may experience dysphagia and intake disorders,Citation8 which can substantially decrease quality of life. Thus, there is a need for novel strategies to improve current therapy. The concept of using the combination of a light source and nanoparticles to ablate cancerous tissue without damaging healthy tissue could have a competitive advantage when compared with the current therapeutic strategies for esophageal adenocarcinoma.Citation9
Recently, strategies for cancer treatment using polymeric nanoparticles have been developed. Polymeric nanoparticles, which are solid colloidal particles smaller than 1000 nm, can be classified into two main subgroups, ie, nanospheres and nanocapsules.Citation10,Citation11 Because nanoparticles have unique characteristics, including a very small particle size, a high surface area, and the possibility of surface modification, they have been attracting much interest for drug delivery purposes during recent years. However, despite much initial promise, their success has so far been limited. Problems related to nanoparticle drug delivery include the limited quantity of nanoparticle preparations available and the toxicity of chemotherapeutic drugs. In addition, targeting specificity remains a key problem.Citation12
Light-absorbing metallic nanoparticles designed to have maximal resonance at a particular wavelength can be used in conjunction with a near infrared light source to trigger a photothermal effect in the particles, whereby electronic oscillations at the particle surface are converted into heat.Citation13 Several classes of near infrared-absorbing nanoparticle formulations have resulted in particles that are nontoxic to biological tissues and have demonstrated the potential to deliver heat to tumors with minimal damage to healthy surrounding tissues.Citation14 These include nanorods,Citation15–Citation18 nanocages,Citation11,Citation19 nanoshells,Citation20–Citation23 and gold/gold sulfide (GGS) nanoparticles.Citation24,Citation25 The resulting localized hyperthermia has been shown to induce damage to individual cells, creating a very elegant targeted and potent therapeutic agent. When the nanoparticles get comparatively hot, several effects are produced, including denaturing target cells, triggering bubble nucleation, and imparting a mechanical stress to target cells, the end result of which is cell death. In previous studies, we have demonstrated the photothermal ablation effect of gold nanoparticles targeted to particular cells,Citation26,Citation27 and the use of gold sulfide nanoparticles in in vitro and in vivo applications. In the research presented in this paper, GGS nanoparticles were coated with a natural polymer (chitosan) to offer distinctive advantages for biocompatibility.Citation9 We used chitosan-coated gold/gold sulfide (CS-GGS) nanoparticles to investigate the effect of photothermal ablation in both esophageal cell lines in vitro and a rat esophageal cancer model in vivo established in our laboratory using surgical esophagoduodenal anastomosis (EDA).
Materials and methods
Preparation of CS-GGS nanoparticles
GGS nanoparticles with controllable near-infrared absorption were prepared by mixing 1.71 mM HAuCl4 (Au 49.68%, Alfa Aesar, Ward Hill, MA, USA) with 3 mM Na2S2O3 5H2O (99.999%, Sigma-Aldrich, St Louis, MO, USA) solutions. A Na2S2O3 solution was added rapidly to the HAuCl4 solution at a volume ratio to give the desired final peak near infrared wavelength. The size of the GGS nanoparticles observed by transmission electron microscopy was in the range of 30–90 nm, with a hydrodynamic size of approximately 290 nm.
EDA model to induce esophageal cancer
We established an EDA model to induce stepwise carcinogenetic transformation of esophageal cancer.Citation28,Citation29 We have demonstrated that the EDA model is consistent with the clinically relevant pathological changes of esophageal cancer.Citation30,Citation31 The EDA surgical procedure was performed as described previously. In brief, 8-week-old Sprague-Dawley rats were anesthetized with 60 mg/kg of sodium pentobarbital. The gastroesophageal junction was ligated flush with the stomach, and the distal esophagus was transected proximal to the ligature. An enterotomy was made 1 cm distal to the pylorus on the antimesenteric border. The distal esophagus was anastomosed to the duodenal enterotomy with mucosal-to-mucosal opposition.
Preparation of CS-GGS nanoparticles
GGS nanoparticles with controllable near infrared absorption were prepared by mixing 1.71 mM HAuCl4 and 3 mM Na2S2O3 · 5H2O solutions.
Murine endoscopy, GGS nanoparticle delivery, and near infrared light exposure
In this study, we used a murine endoscopeCitation32 to guide delivery of the gold nanoparticles into the esophageal mucosa, and used a 400 μm fiber optic probe for exposure to near infrared light in the EDA rat. Gold nanoparticles were delivered by a 22-gauge aspiration needle through a 2.8 mm protective sheath with the assistance of a microendoscope. A bare fiber optic probe was inserted into the esophageal lumen through the protective sheath. The light source used for exposure to near infrared light was a 30 W FAP-I system (Coherent, Santa Clara, CA, USA), with a wavelength of 818 nm. For treatment, a power density of 3 W/cm2 was used, based on previous studies.Citation22 Two procedures were used for exposure to near infrared light, ie, external and internal exposure. The rats were anesthetized for both procedures. For external exposure, the rat was shaved on the abdominal area, with the EDA site directly underneath, and 20% glycerol was applied to the skin to prevent direct injury by near infrared light. The near infrared light probe was placed vertically on the abdominal area directly over the EDA (10 cm). The near infrared light exposure area was 2 cm in diameter on the abdominal skin. Internal exposure was performed under microendoscopy, with the near infrared light probe located at the targeted esophageal epithelial tissue. Twenty-four hours after delivery of the CS-GGS nanoparticles, exposure to near infrared light was performed at the local targeted tissue, both at high exposure (one minute at 3 W/cm2) or low exposure (30 seconds at 1 W/cm2).
Histopathology
The entire esophagus was removed and opened longitudinally to examine for evidence of gross morphological changes. Evidence of reflux esophagitis, Barrett’s esophagus, and esophageal adenocarcinoma was sought microscopically in the esophageal epithelium as reported elsewhere.Citation33 Uptake of the nanoparticles was detected by silver enhancement.
TUNEL assay of ex vivo samples
In addition to the histology study, a TUNEL assay was used to evaluate cell death in esophageal epithelium closest to the area of near infrared light exposure. An ApopTag® in situ apoptosis detection kit (Intergen Company, Purchase, NY, USA) was used to detect apoptotic cells following a method reported previously.Citation34,Citation35
In vitro testing
Three esophageal cell lines were used to analyze further the response of the esophageal cells to the CS-GGS nanoparticles and near infrared light. The cells used were a human squamous esophageal epithelium cell line (Het-1A), a Barrett’s epithelium cell line (BAR-T), and an esophageal adenocarcinoma cell line (OE-19). The three cell types were seeded in 24-well plates at a density of 2 × 105 cells per well for treatment with CS-GGS nanoparticles. Two days after plating, the cells were treated with serum-free medium for 12 hours, and then treated with CS-GGS nanoparticles at a concentration of 1 OD (diluted from a 25 OD stock solution of CS-GGS nanoparticles). The particle concentration at 1 OD is estimated to be 3 × 1011 particles per mL based on scattering theory and geometric/size analysis. Twenty-four hours after application of the nanoparticles, the cells were exposed to near infrared light using an FAP-I laser system at a wavelength of 818 nm and a power density of high exposure (one minute at 3 W/cm2) or low exposure (30 seconds at 1 W/cm2). Silver staining was used to detect nanoparticle loading in the cells.
Cell viability assay
To study cell viability after treatment with CS-GGS nanoparticles and near infrared light, we performed a 3-(4, 5-dimethyl-thiazol-2-yl)-2, 5-diphenyltetrazolium bromide (MTT, Sigma-Aldrich) reduction assay according to previous reports. One day after seeding, the cells were treated with either nanoparticles for 12 hours or exposed to near infrared light at either high or low exposure. MTT was added to the cultured cells at 10 μL/well (5 mg/mL in phosphate-buffered solution) 4 hours before the end of treatment with the nanoparticles and near infrared light. Absorbance was determined spectrophotometrically using a plate reader at a wavelength of 570 nm. Mean optical density values from triplicate wells for each treatment were used as the index of cell viability.
Western blotting
To study the response of the cells to treatment with nanoparticles and near infrared light, we performed Western blotting to determine the presence of protective proteins (catalase, manganese superoxide dismutase, copper/zinc superoxide dismutase, and heat shock protein 60) along with the cell death effector (caspase-3) in esophageal epithelium.
Statistical analysis
The Student’s t-test was performed, assuming unequal variance. The results are expressed as the mean ± standard deviation. Comparisons were made between groups using analysis of variance. A P value < 0.05 was considered to be statistically significant.
Results
EDA-induced metaplasia and adenocarcinoma in rat esophageal epithelium
In previous studies, we reported that the endoscopic appearance of EDA esophageal mucosa is characterized by wall thickening, polypoid fold formation, ulceration, and wall hypomotility. In contrast with EDA mucosa, the normal rat esophageal mucosa has a squamous epithelium that looks smooth and white, with blood vessels that are clearly visible beneath the surface.Citation32 In this study, we performed murine endoscopy on the EDA rats 4 months after the surgical procedure. At endoscopy, we found morphological changes similar to our previous findings in EDA mucosa. In addition, we found suspicious red-colored glandular epithelium in the EDA mucosa (). Before performing photothermal ablation therapy with nanoparticles and laser light, three EDA rats were sacrificed to verify the presence of Barrett’s metaplasia and esophageal adenocarcinoma. EDA specimens from three rats were harvested and opened longitudinally to examine for gross abnormalities. The tumor could be seen growing in the EDA site, while the red and texturized mucosa was patched in polypoid folders (). Both the tumor tissue and red mucosa were then isolated and prepared for histological study. Hematoxylin and eosin staining and microscopic imaging demonstrated that the red mucosa and tumor tissue was metaplasia and adenocarcinoma, respectively. On endoscopy, Barrett’s metaplasia and adenocarcinoma were found at the anastomotic site but separate from the duodenal epithelium. On microscopy, Barrett’s metaplastic epithelium and adenocarcinoma were seen as submucosal ducts with the appearance of goblet cells. The histological changes for Barrett’s metaplastic epithelium and adenocarcinoma were consistent with our endoscopic findings. Representative histology of Barrett’s esophagus and esophageal adenocarcinoma on staining with hematoxylin and eosin are shown in .
Figure 1 Endoscopic view, gross anatomy, and histology. (A) Endoscopic view of the esophageal epithelium. (A1) Normal esophageal epithelium; (A2) suspicious glandular epithelium appearing red-colored in the mucosa of the esophagoduodenal anastomosis, which was subsequently demonstrated to be Barrett’s metaplasia by histology; and (A3) suspicious glandular epithelium, which was later demonstrated to be esophageal adenocarcinoma by histology. (B) Gross anatomy. (B1) Normal esophageal epithelium; (B2) polypoid folders caused by esophagoduodenal anastomosis, with red mucosal patch in the esophageal epithelium; and (B3) tumor growing in the esophagoduodenal anastomotic site. (C) Histology of esophageal epithelium. (C1) Normal esophageal epithelium; (C2) Barrett’s metaplasia; and (C3) esophageal adenocarcinoma.
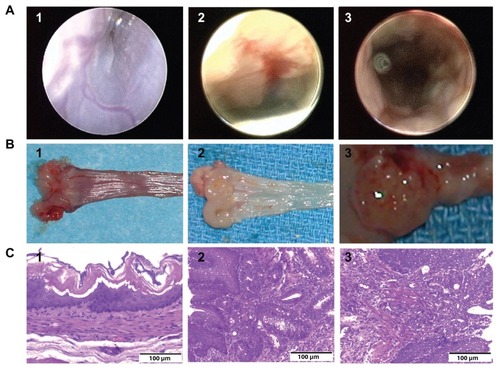
Killing of cells loaded with CS-GGS nanoparticles and exposed to near infrared light in vivo
After the pilot study for endoscopic determination of metaplasia and adenocarcinoma, we compared the effect of uptake of gold nanoparticles and exposure to near infrared light in EDA and normal esophageal mucosa. CS-GGS nanoparticles were delivered through the endoscope to the lower esophageal lumen, where the appearance of Barrett’s metaplasia and adenocarcinoma in the mucosa was highest. Twenty-four hours after delivery of the nanoparticles, the lower esophagus was exposed to near infrared light in the treatment group; the control group received neither nanoparticles nor exposure to near infrared light (). After exposure, the esophageal tissues were harvested to investigate uptake of CS-GGS nanoparticles and the cellular response to subsequent exposure to near infrared light. Hematoxylin and eosin staining and the TUNEL assay were used to assess esophageal epithelial cell death and apoptosis. In the slides stained with hematoxylin and eosin, we found that exposure to near infrared light without nanoparticle loading had no effect on the normal esophageal mucosa, metaplastic mucosa, or adenocarcinoma mucosa (data not shown). In contrast, exposure to near infrared light showed cell death when gold nanoparticle loading was evident. Gold nanoparticle loading was demonstrated by histology with silver staining (). As expected, the esophageal mucosa not containing gold nanoparticles remained viable after exposure to near infrared light. To evaluate the tissue adjacent to the region exposed to near infrared light, we did a TUNEL assay on the paraffin-embedded slides, and numerous TUNEL-positive cells were found in the esophageal epithelium of the animals treated with nanoparticles and exposure to near infrared light. However, in control animals which received neither nanoparticles nor near infrared light, the number of TUNEL-positive cells in the esophageal epithelium was much lower. An apoptotic index was obtained by counting the percentage of TUNEL-positive epithelial cells, and this was significantly increased in EDA tissue exposed to near infrared light compared with that in the esophageal epithelium of controls (P < 0.05). Representative apoptosis using the apoptotic index is shown in .
Figure 2 Mucosal ablation in the esophageal lumen as a result of loading with gold nanoparticles and exposure to near infrared light. (A) Delivery of gold nanoparticles and exposure to near infrared light in the esophageal lumen. Gross anatomy and histology in the ablated area is shown. (B) Apoptosis associated with thermal ablation in the adjacent tissue after exposure to near infrared light.
Abbreviations: UT, untreated; GN +nIR, gold nanoparticles treated with infrared light.
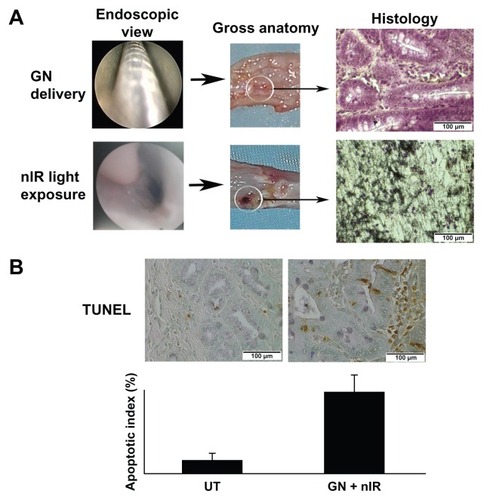
Exposure to near infrared light destroyed cancer cells with high affinity to CS-GGS nanoparticles in vitro
We evaluated death in cells induced by loading CS-GGS nanoparticles and exposure to near infrared light in vitro. Three types of epithelial cells (HET-1A, benign; BAR-T, metaplasia; OE-19, adenocarcinoma) were treated with CS-GGS nanoparticles for 24 hours, and then exposed to near infrared light. We found that the OE-19 cells showed very high affinity for the nanoparticles, and most OE-19 cells loaded with nanoparticles were completely ablated upon exposure to near infrared light. Under the microscope, most Het-1A cells did not show uptake of CS-GGS nanoparticles, whereas a few BAR-T cells showed a small amount of uptake compared with OE-19 cells (). The BAR-T cells did not show any uptake of nanoparticles, with most of the nanoparticles remaining clustered in the extracellular regions. Exposure to near infrared light destroyed most of the OE-19 cells, whereas the HET-1A and BAR-T cells remained viable ().
Figure 3 Cytotoxic effect of loading with gold nanoparticles in three esophageal cell lines (first column is lower magnification and second column is higher magnification).
Notes: Arrow: gold nanoparticle loading in cells. Red arrow head: cells were removed after near infrared light exposure.
Abbreviations: UT, untreated; GN, gold nanoparticles; GN + nIR, gold nanoparticles treated with infrared light.
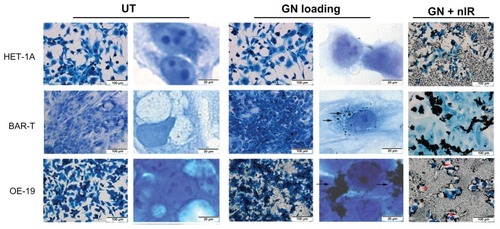
Effects of CS-GGS nanoparticles and near infrared light on benign, metaplastic, and cancer cells
We demonstrated that near infrared light could destroy the cells loaded with gold nanoparticles but not the control cells. This observation potentially reflects a photothermal effect occurring when the gold nanoparticles are heated. We then evaluated the effects of the nanoparticles and near infrared light exposure on cell viability in vitro. To quantify cell viability in response to exposure to near infrared light and to the nanoparticles, we performed an MTT assay in which optical density was used as an index for cell growth. As shown in , neither treatment with CS-GGS nanoparticles nor exposure to near infrared light affected the viability of any of the three cell lines after 24 hours when compared with controls (P > 0.05). However, the cell death rate was increased significantly in OE-19 cells loaded with the nanoparticles after exposure to near infrared light (). In addition, we evaluated whether gold nanoparticles and exposure to near infrared light could affect expression of cell defense proteins (manganese superoxide dismutase, catalase, and copper/zinc superoxide dismutase) or the cell death effector (caspase-3). These parameters were chosen because a large body of work implicates mitochondrial impairment as being an important cause of cell death, particularly given that the mitochondria serve as gatekeepers for the “life-or-death” decision.Citation36 Therefore, we studied mitochondrial manganese superoxide dismutase, which is a major cell defense protein against cell death. The other two major proteins, catalase and copper/zinc superoxide dismutase, are also important in cell defense against insults from oxidative stress. Caspase-3 is an important cell death effector in the extrinsic and mitochondrial pathways. We evaluated the responses of all these proteins to treatment with CS-GGS nanoparticles and exposure to near infrared light in the three esophageal cell lines. The Western blot results show that neither the nanoparticles nor exposure to near infrared light affected these proteins ().
Figure 4 Effects of gold nanoparticle loading and exposure to near infrared light in three esophageal cell lines. (A) Effect of gold nanoparticles and near infrared light on cell viability in all three cell lines. There is no difference in cell viability after treatment with gold nanoparticles alone or exposure to near infrared light alone. (B) The effect of gold nanoparticles and nIR light on MnSOD, Cu/ZnSOD and catalase in three cell lines. There is no difference for the key protective proteins (catalase, MnSOD, Cu/ZnSOD, and GAPDH) in three cell lines. (C) The effect of gold nanoparticles and near infrared light on caspase-3 in all three cell lines.
Notes: Caspase-3 expression is in an inactive form in all three cell lines and no active forms, such as p20 or p17, were cleaved in the three cell lines after treatment with gold nanoparticles or exposure to near infrared light.
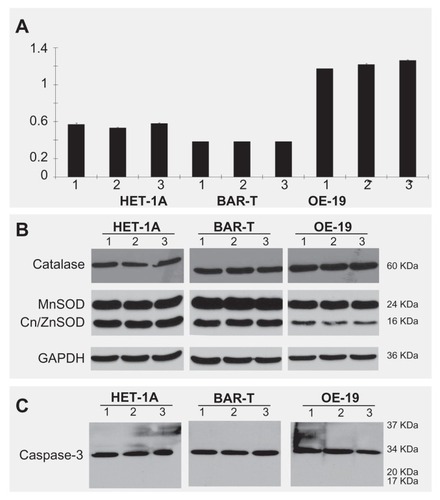
Discussion
Current multidisciplinary treatments for esophageal adenocarcinoma include radiotherapy, chemotherapy, and surgical resection. However, there is still a need to optimize local therapy using novel alternatives which overcome toxicity and intolerance and improve the present modest response rates. In this study, we investigated the possibility of treating esophageal cancer by introducing near infrared-absorbing CS-GGS nanoparticles and laser exposure through an endoscope in our established rat EDA model. The CS-GGS nanoparticles were taken up readily by tumor cells, with relatively low cytotoxicity to healthy cells. This novel material has many attractive features, including biocompatibility, no heavy metal toxicity, and strong optical absorption in the near infrared region, allowing development for photothermal therapeutic application.
This study demonstrates three advantages of a potential photoablative therapy to combat esophageal cancer:
endoscopic delivery, whereby gold nanoparticles can be delivered with the assistance of a microendoscope via a needle and protective sheath; near infrared light exposure is successfully performed using a microfiber probe integrated with a laser system in the esophageal lumen; and endoscopic delivery may conceivably provide the opportunity to ablate esophageal cancer in patients who are not candidates for surgery
two techniques which are each innocuous until combined, with near infrared light exposure not affecting normal esophageal tissue unless it was loaded with CS-GGS nanoparticles, suggesting that gold nanoparticles coupled with near infrared light exposure could enable thermal-based ablation targeted specifically at cancerous tissue, which is consistent with other reportsCitation14
an ability to target cancer cells selectively, as indicated in vitro by the high affinity of malignant OE-19 cells for CS-GGS nanoparticles, whereas the two benign cell lines, ie, BAR-T and HET-1A cells, showed less affinity; the differential uptake of CS-GGS between cancerous and benign tissue could enable targeted destruction of only cancerous tissue.
There are questions that remain to be answered, as well as technical obstacles to be overcome in future studies. First, loading with CS-GGS nanoparticles could be a dynamic process, in which accumulation of the nanoparticles in esophageal adenocarcinoma could reach a peak at a certain time point in vivo. Peak loading could involve an “optimal therapeutic window” for near infrared light exposure, and this will need to be explored in future research. Second, it needs to be confirmed that normal esophageal epithelial or submucosal cells do not take up CS-GGS nanoparticles in vivo, to ensure that near infrared light only destroys cancer cells loaded with nanoparticles and not normal healthy cells. In our study, we found that some regions on the esophageal mucosa were “burned” on exposure to near infrared light, and these regions were later demonstrated to include both cancerous and adjacent healthy tissue. In the in vitro study, we have found that HET-1A, a benign esophageal cell line, had very low affinity for gold nanoparticles, so the observed photothermal ablation effect on adjacent benign tissue could indicate infiltration by inflammatory cells, such as phagocytes, but this needs to be confirmed. Therefore, uptake of these nanoparticles in both cancerous and benign tissue needs to be evaluated further in vivo. A dynamic uptake study may help us to understand better the nature of nanoparticle loading in cancerous tissue and to what extent, if any, this occurs in benign esophageal mucosa. A dynamic study of this sort would allow us to determine if nanoparticle loading differs between benign and cancerous tissue, and if there is a time point when the nanoparticles become highly concentrated in cancerous tissue but not in healthy tissue. Third, it is generally accepted that these nanoparticles are not associated with heavy metal toxicity. We did not observe any toxic effects of CS-GSS nanoparticles on the benign HET-1A and BAR-T cell lines. However, the potential for metabolism of CS-GSS nanoparticles in the body needs to be evaluated further in vivo, particularly with regard to any toxic effects on the brain, kidney, and heart.
Regarding the technical obstacles, there are some important issues which require attention in a future study. In our study, the CS-GSS nanoparticles were sprayed onto the surface of suspicious mucosa at a high concentration (50 OD) to ensure absorption. One needs to take great care with direct injection of nanoparticles so as to minimize the risk of them becoming trapped in the interstitium of benign tissue. Our in vitro study showed that although the benign Het-1A cells did not become loaded with nanoparticles, we did find a large amount of gold nanoparticles attached on the bottom of the culture plate, which was probably the result of precoating the plate with a mixture of fibronectin, collagen type I, and bovine serum albumin, which are necessary for the growth of Het-1A cells. These extracellular matrices are very rich in interstitium, so loading of esophageal tissue with CS-GSS nanoparticles needs to be well controlled.
Another important issue is the use of a laser probe in the esophageal lumen. We used a murine endoscope to guide cannulation of a microfiber probe to the target esophageal epithelium. Although exposure to near infrared light is safe in esophageal epithelium not loaded with nanoparticles, the microfiber optic probe could cause injury to this tissue if it is too close to is directly touching the surface of the esophageal epithelium. During an attempt at external near infrared illumination, in which we placed the laser directly on top of the body, no ablation of the esophageal mucosa was observed, even though the mucosa was loaded with nanoparticles. Part of the reason for this could have been the depth of penetration of near infrared light into the body. A modified radiofrequency might need to be used to improve penetration of near infrared light into deeper sites of interest.
In this study, we investigated the ability of near infrared-absorbing CS-GGS nanoparticles to destroy esophageal cancer cells using an endoscopic procedure. Taken together, our study shows that introducing near infrared-absorbing CS-GGS nanoparticles into the esophageal mucosa to ablate cancer tissue under endoscopy is technically feasible. This technology holds exciting potential for use in patients with esophageal cancer who are not candidates for surgery, although considerable research on this strategy remains to be done in the future.
Acknowledgment
This work was supported by The Clinical and Translational Science Pilot Grant Program’s Basic Award and Innovative Award at University of Louisville 2010, and by the Coulter Foundation Clinical Translational Research Award. BAR-T is a human hTERT-immortalized benign Barrett’s cell line, which was generously gifted by Rhonda Souza and SJ Spechler at the Department of Medicine, VA North Texas Health Care System and the University of Texas Southwestern Medical School, Dallas, TX, USA.
Disclosure
None of the authors has a commercial or institutional association, currently or within the past five years, that might pose a potential, perceived, or real conflict of interest in this work.
References
- JemalASiegelRWardEHaoYXuJThunMJCancer statistics, 2009CA Cancer J Clin20095922524919474385
- LindbladMYeWLindgrenALagergrenJDisparities in the classification of esophageal and cardia adenocarcinomas and their influence on reported incidence ratesAnn Surg200624347948516552198
- VizcainoAPMorenoVLambertRParkinDMTime trends incidence of both major histologic types of esophageal carcinomas in selected countries, 1973–1995Int J Cancer20029986086812115489
- AltorkiNKOliveriaSSchrumpDSEpidemiology and molecular biology of Barrett’s adenocarcinomaSemin Surg Oncol1997132702809229415
- BlotWJMcLaughlinJKThe changing epidemiology of esophageal cancerSemin Oncol1999262810566604
- FarrowDCVaughanTLDeterminants of survival following the diagnosis of esophageal adenocarcinoma (United States)Cancer Causes Control199673223278734825
- KuGYIlsonDHRole of neoadjuvant therapy for esophageal adenocarcinomaSurg Oncol Clin N Am20091853354619500742
- LawsonJDOttoKGristWJohnstonePAFrequency of esophageal stenosis after simultaneous modulated accelerated radiation therapy and chemotherapy for head and neck cancerAm J Otolaryngol200829131918061826
- BocaSCPotaraMGabudeanAMJuhemABaldeckPLAstileanSChitosan-coated triangular silver nanoparticles as a novel class of biocompatible, highly effective photothermal transducers for in vitro cancer cell therapyCancer Lett201131113114021840122
- MoghimiSMRecent developments in polymeric nanoparticle engineering and their applications in experimental and clinical oncologyAnticancer Agents Med Chem2006655356117100563
- MoonGDChoiSWCaiXA new theranostic system based on gold nanocages and phase-change materials with unique features for photoacoustic imaging and controlled releaseJ Am Chem Soc20111334762476521401092
- MoghimiSMKisselTParticulate nanomedicinesAdv Drug Deliv Rev2006581451145517081650
- AvedisianCTCavicchiREMcEuenPLZhouXNanoparticles for cancer treatment: role of heat transferAnn N Y Acad Sci20091161627319426306
- CardinalJKluneJRChoryENoninvasive radiofrequency ablation of cancer targeted by gold nanoparticlesSurgery200814412513218656617
- GentiliDOriGComesFMDouble phase transfer of gold nanorods for surface functionalization and entrapment into PEG-based nanocarriersChem Commun (Camb)2009395874587619787126
- TakahashiHNiidomeYNiidomeTKanekoKKawasakiHYamadaSModification of gold nanorods using phosphatidylcholine to reduce cytotoxicityLangmuir2006222516378388
- NiidomeTYamagataMOkamotoYPEG-modified gold nanorods with a stealth character for in vivo applicationsJ Control Release200611434334716876898
- HuangXEl SayedIHQianWEl SayedMACancer cell imaging and photothermal therapy in the near-infrared region by using gold nanorodsJ Am Chem Soc20061282115212016464114
- ChenJWangDXiJImmuno gold nanocages with tailored optical properties for targeted photothermal destruction of cancer cellsNano Lett200771318132217430005
- GobinAMLeeMHHalasNJJamesWDDrezekRAWestJLNear-infrared resonant nanoshells for combined optical imaging and photothermal cancer therapyNano Lett200771929193417550297
- LoweryARGobinAMDayESHalasNJWestJLImmunonanoshells for targeted photothermal ablation of tumor cellsInt J Nanomedicine2006114915417722530
- O’NealDPHirschLRHalasNJPayneJDWestJLPhoto-thermal tumor ablation in mice using near infrared-absorbing nanoparticlesCancer Lett200420917117615159019
- HirschLRStaffordRJBanksonJANanoshell-mediated near-infrared thermal therapy of tumors under magnetic resonance guidanceProc Natl Acad Sci U S A2003100135491355414597719
- DayESBickfordLRSlaterJHRiggallNSDrezekRAWestJLAntibody-conjugated gold-gold sulfide nanoparticles as multifunctional agents for imaging and therapy of breast cancerInt J Nanomedicine2010544545420957166
- GobinAMWatkinsEMQuevedoEColvinVLWestJLNear-infrared- resonant gold/gold sulfide nanoparticles as a photothermal cancer therapeutic agentSmall2010674575220183810
- GobinAMO’NealDPWatkinsDMHalasNJDrezekRAWestJLNear infrared laser-tissue welding using nanoshells as an exogenous absorberLasers Surg Med20053712312916047329
- GobinAMMoonJJWestJLEphrinA I-targeted nanoshells for photothermal ablation of prostate cancer cellsInt J Nanomedicine2008335135818990944
- LiYWoJMSuRRRayMBMartinRCLoss of manganese superoxide dismutase expression and activity in rat esophagus with external esophageal perfusionSurgery200714135936717349848
- MartinRCLiuQWoJMRayMBLiYChemoprevention of carcinogenic progression to esophageal adenocarcinoma by the manganese superoxide dismutase supplementationClin Cancer Res2007135176518217785574
- WoodallCELiYLiuQHWoJMartinRCChemoprevention of metaplasia initiation and carcinogenic progression to esophageal adenocarcinoma by resveratrol supplementationAnticancer Drugs20092043744319398904
- LiYWoodallCWoJMThe use of dynamic positron emission tomography imaging for evaluating the carcinogenic progression of intestinal metaplasia to esophageal adenocarcinomaCancer Invest20082627828518317969
- SchiffmanSCLiYDrydenGLiXMartinRCPositive correlation of image analysis by mini-endoscopy with micro-PET scan and histology in rats after esophagoduodenal anastomosisSurg Endosc2010242835284120440518
- Ismail-BeigiFHortonPFPopeCEHistological consequences of gastroesophageal reflux in manGastroenterology1970581631745413015
- LiYWoJMSuRRRayMBJonesWMartinRCEsophageal injury with external esophageal perfusionJ Surg Res200512910711315921698
- LiYWoJMEllisSRayMBJonesWMartinRCA novel external esophageal perfusion model for reflux esophageal injuryDig Dis Sci20065152753216614962
- FosslienEMitochondrial medicine – molecular pathology of defective oxidative phosphorylationAnn Clin Lab Sci200131256711314862